The Special Senses PDF
Document Details
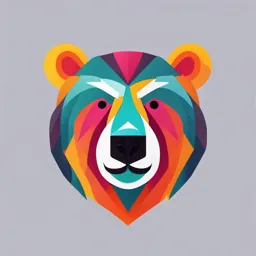
Uploaded by PhenomenalFlugelhorn
Universiti Teknologi MARA
Dr. Richard Muhammad Johari James
Tags
Related
- Human Anatomy and Physiology Chapter 15 Fall 2020 PDF
- Essentials of Human Anatomy & Physiology PDF
- Special Senses 15-02 PDF
- MLS 111L Human Anatomy and Physiology With Pathophysiology PDF
- Human Anatomy: Special Senses Study Guide (PDF)
- Essentials of Human Anatomy & Physiology Chapter 8 Special Senses PDF
Summary
These lecture notes provide an overview of the special senses, focusing on the eye, vision, chemical senses (smell and taste), and the ear. The document details the structure and function of these systems, including diagrams to aid understanding.
Full Transcript
The Special Senses Associate Prof. Ts. Dr. Richard Muhammad Johari James Integrative Pharmacogenomics Institute (iPROMISE) Learning Outcome At the end of the lecture, students will be able to describe the eye and vision, the chemical senses and the ear. Lecture outline 1. The eye and vision...
The Special Senses Associate Prof. Ts. Dr. Richard Muhammad Johari James Integrative Pharmacogenomics Institute (iPROMISE) Learning Outcome At the end of the lecture, students will be able to describe the eye and vision, the chemical senses and the ear. Lecture outline 1. The eye and vision 2. The chemical senses: smell 3. The chemical senses: taste 4. The ear 1. The eye and vision The eyes are located within the skull orbits, which provide protection for the eyes, as well as provide a place to anchor the soft tissues that support the functions of the eye. The eyelids and the lashes help to protect the eye from abrasions by blocking particles that may get onto the surface of the eye. A thin mucous membrane known as the conjunctiva folds in and covers the surface of the eye from the inner surface of each lid. Tears are produced by the lacrimal glands, they flow over the conjunctiva to wash away particles that may have gotten past the lashes and the lids. Tears flow down through the nasolacrimal ducts, located on the medial side of each orbit, into the nasal cavity. The eye is a hollow sphere composed of three layers of tissue. The outermost layer is the fibrous tunic which is the white sclera and clear cornea. The two parts of the fibrous tunic are continuous, though they have different properties. The sclera accounts for 5/6 of the surface of the eye, most of which is not visible. The cornea covers the anterior region of the eye and allows light to pass into the eye where it will eventually stimulate photoreceptors. The next layer of the eye is the vascular tunic, which is mostly composed of the choroid, a highly vascularized connective tissue that provides a blood supply to the adjacent tissue. The choroid is posterior to the ciliary body, a muscular structure that is attached to the lens by the suspensory ligament. The ciliary body focuses light on the back of the eye. Overlaying the ciliary body, and visible in the anterior eye, is the iris, the colored part of the eye that opens in the center as the pupil. The innermost layer of the eye is the neural tunic, which is the retina or the nervous tissue that is responsible for photoreception. The eye is also divided into two cavities, the anterior and posterior chamber. The anterior chamber is the space between the cornea and iris. The posterior chamber sits between the iris and the lens. Both the anterior and posterior chambers are filled with a watery fluid called the aqueous humor. The posterior vitreous chamber is posterior to the lens and is filled with a more viscous fluid called the vitreous humor. Movement of the eye within the orbit is accomplished by the contraction of six extraocular muscles that originate from the bones of the orbit and insert into the surface of the eye. The retina, where the photoreceptors are found, is located at the posterior aspect of the eye. Because both the cornea and lens have curved surfaces, they refract some of the light rays entering the eye. In doing so, they compress the image so that a large amount of visual information can be processed by a small amount of retinal tissue. At the exact center of the retina is a point where light is focused by the lens and the greatest visual acuity is found. This is known as the fovea. It is a small dimple in the layers of the retina where there are no blood vessels, ganglion cells or bipolar cells to interrupt light reaching the receptor cells. Because more light passes to the receptor cells at the fovea, it is in this region that visual acuity is the greatest. From this central point of the retina, visual acuity drops off towards the peripheral retina. A large part of neural function to support the visual system is concerned with moving the eyes and head so that important visual stimuli are centered on the fovea of the retina. The cornea refracts more light than the lens does because its surface is more curved, but the lens has the ability to change its shape, and therefore fine-tune the amount of refraction necessary to focus the light rays on the retina. This process is known as accommodation. The lens changes its shape in response to changes in tension of the ciliary muscles on the suspensory ligaments that hold the lens in place. Along with accommodation of the lens when objects are near, the pupil also tends to constrict to allow less peripheral light to enter the posterior chamber of the eye. The pupil will also constrict when conditions are bright and dilate under low light conditions. This way the retina can receive an appropriate amount of light to activate its photoreceptors without bleaching them with too much light. Sometimes the structures of the eye do not refract light appropriately. Myopia or nearsighted, is when the light is focused in front of the retina It is corrected with a concave lens Hyperopia is when the light is focused behind the retina. It is corrected with a convex lens. Light falling on the retina causes chemical changes to pigment molecules called opsins in photoreceptors, ultimately leading to a change in the activity of the retinal ganglion cells. Photoreceptor cells have two parts, the inner segment and the outer segment. The inner segment contains the nucleus and other common organelles of a cell while the outer segment is a specialized region of the cell where photoreception takes place. There are two types of photoreceptors, rods and cones, based on the shape of their outer segment. The outer segments of rod photoreceptors contain a stack of membrane-bound discs that contain a photosensitive opsin pigment called rhodopsin, which is sensitive to white light. The cone-shaped outer segments of cone cells contain one of three photosensitive opsin pigments, called photopsins. Each of the three photopsins are sensitive to a particular bandwidth of light, corresponding to the colors of red, green or blue, allowing for the ability to distinguish color. When a photoreceptor cell is activated by a photon near the wavelength it is sensitive to, the energy from the light creates a change in its opsin molecule called photoisomerization. Photoisomerization is the first step in a process that ultimately leads to a change in membrane potential of the photoreceptor. Until the opsin is changed back to its original shape, the photoreceptor cell cannot respond to light energy, which is called bleaching. When a large group of opsins are bleached, vision will be affected until enough opsins can return to the receptive state. Because rhodopsin found in the rod cells is most sensitive to white light while the cone cells are color specific, rods are suited for vision in low-light conditions and cones are suited for brighter conditions. In normal sunlight, rhodopsin will be constantly bleached and the cones are active. In a darkened room, there is not enough light to activate cone opsins, and vision is entirely dependent on rods. Transduction of the visual signal The photoreceptors, and other neuronal cells of the retina, send varied types of information to the brain. These include light intensity, colors and the spatial distribution of the information received. All of this information is then carried along the optic nerve and into the optic tract to be distributed to nuclei in the brain. At the point where the optic nerve becomes the optic tract, the optic chiasm is found. At this point, fibers carrying information from the nasal half of the retina on each side decussate or cross over, such that the information from the nasal half of the retina of the left eye crosses over to the right side of the brain and vice versa. 2. The chemical senses: smell Olfaction, or the sense of smell, is the process of creating the perception of smell. It occurs when an odor binds to a receptor within the nose, transmitting a signal through the olfactory system. The olfactory receptor neurons are incorporated into a limited region of the nasal epithelium in the superior nasal cavity. This region is referred to as the olfactory epithelium and contains bipolar sensory neurons with dendrites extending from the apical surface of the epithelium into the mucus lining the nasal cavity. The olfactory epithelium consists of a layer of olfactory receptor cells, supporting cells and basal cells. Odorants dissolve in the mucus layer and contact the cilia of the olfactory cells Axons of the olfactory cells penetrate the bony cribriform plate on their way to the central nervous system. Smell is difficult to research because any given odor may be made up of hundreds of different chemicals. Humans can distinguish 10,000 or so odors, but research suggests that the olfactory receptors are stimulated by different combinations of a more limited number of olfactory qualities. Dissolved odorants stimulate the olfactory receptors by binding to receptor proteins in the olfactory cilium membranes, opening cation channels and generating a receptor potential. Ultimately an action potential is conducted to the first relay station in the olfactory bulb. Transduction of olfactory signals The transduction of odorants uses a receptor linked to a G protein. Odorant binding to the membrane receptor proteins stimulates the G- protein, which in turn activates the adenylyl cyclase. Adenylyl cyclases synthesize cyclic AMP which binds to specific cation channels. The binding of cyclic AMP-protein induces the opening of cation channels and influx of sodium and calcium. The sodium influx leads to depolarization and impulse transmission. The calcium influx causes the transduction process to adapt, decreasing its response to a sustained stimulus. The axons of the olfactory neurons extend from the basal surface of the epithelium, through an olfactory foramen in the cribriform plate of the ethmoid bone, and into the olfactory bulb, located on the ventral surface of the frontal lobe. Collectively the axons that pass through the cribriform plate are called the olfactory nerve. From the olfactory bulb, axons project to structures within the limbic cortex, including the cortex in the temporal lobe associated with long- term memory formation. 3. The chemical senses: taste Taste, or gustation, is a sense that develops through the interaction of dissolved molecules with taste buds. There are five basic tastes: sweet, salty, bitter, sour, and umami. Basic tastes Saltiness salt, mostly taste of Na+ Sourness most acids Sweetness sugars, certain proteins Bitterness K+ , Mg2+ , caffeine, alkaloids Umami glutamine Taste is associated mainly with the tongue, although there are taste receptors on the palate and epiglottis as well. The surface of the tongue, along with the rest of the oral cavity, is lined by a stratified squamous epithelium. In the surface of the tongue are raised bumps, called papilla, that contain the taste buds. There are three types of papilla, based on their appearance: vallate, foliate, and fungiform. The number of taste buds within papillae varies, with each bud containing several specialized taste cells for the transduction of taste stimuli. These receptor cells release neurotransmitters when certain chemicals in ingested substances are carried to their surface in saliva. Neurotransmitter from the gustatory cells can activate the sensory neurons in the facial and glossopharyngeal cranial nerves. When a receptor cell is activated, its membrane potential changes thus creating a receptor potential. Mechanism of transduction for the taste of saltiness On the taste receptor cells, there are amiloride-sensitive sodium channels. They are insensitive to voltage and stay open all the time. When the exterior concentration of sodium rises, sodium diffuses into the receptor cell, driven by the concentration gradient. This causes a depolarization, which in turn causes voltage-gated sodium and calcium channels to open near the synaptic vesicles, triggering the release of neurotransmitter onto the gustatory afferent axon. Mechanism of transduction for the taste of sourness The taste of sourness is mediated by protons. Proton can permeate the amiloride-sensitive sodium channels creating an inward proton current which depolarizes the cell. Protons also bind and block potassium selective channels, decreasing the permeability of the membrane to potassium which also depolarizes the cell. As the concentration of the protons increases because of ingesting acidic compounds, the depolarization of specific taste cells increases. Mechanism of transduction for the taste of sweet, bitter and umami The other three tastes; sweet, bitter and umami are transduced through G-protein coupled cell surface receptors instead of the direct diffusion of ions like with salty and sour. The sweet taste is the sensitivity of taste cells to the presence of glucose dissolved in the saliva. Molecules that are similar in structure to glucose will have a similar effect on the sensation of sweetness. Other monosaccharides such as fructose or artificial sweeteners like aspartame, saccharine, or sucralose will activate the sweet receptors as well. The affinity for each of these molecules varies, and some will taste “sweeter” than glucose because they bind to the G-protein coupled receptor differently. As for the bitter taste, the taste buds can be stimulated by a large number of molecules collectively known as alkaloids. And finally, for umami, like sweet and bitter taste, it is based on the activation of G-protein coupled receptors, in this case by amino acids, especially glutamine. Once the taste cells are activated, they release neurotransmitters onto the dendrites of sensory neurons. These neurons are part of the facial and glossopharyngeal cranial nerves, as well as a component within the vagus nerve. Axons from the three cranial nerves carrying taste information travel to the medulla. From there much of the information is carried to the thalamus and then routed to the primary gustatory cortex, located near the inferior margin of the post-central gyrus. It is the primary gustatory cortex that is responsible the sensations of taste. 4. The ear The ear is the organ of hearing and balance. The ear is divided into three major areas: external ear, middle ear, and internal ear. The external ear consists of the auricle and the external acoustic meatus. The auricle, or pinna, is composed of elastic cartilage covered with thin skin and an occasional hair. The function of the auricle is to direct sound waves into the external acoustic meatus. The external acoustic meatus or auditory canal is a short, curved tube that extends from the auricle to the eardrum. The entire canal is lined with skin bearing hairs, sebaceous glands, and modified apocrine sweat glands called ceruminous glands. These glands secrete yellow-brown waxy cerumen, or earwax, which provides a sticky trap for foreign bodies and repels insects. The middle ear consists of ossicles called malleus, incus, stapes. The ossicles amplify the sound force. They increase the sound force at the oval window and smaller surface area at the oval window. The air in the middle ear is continuous with the air in the nasal cavity via the Eustachian tube. This tube is usually closed by a valve. The inner ear is entirely enclosed within the temporal bone. It has two separate regions, the cochlea and vestibule, which are responsible for hearing and balance, respectively. The neural signals from the two regions of the inner ear are relayed to the brainstem through separate fiber bundles, but which run together as the vestibulocochlear nerve. Auditory transduction and pathways Sound information is transmitted from the middle ear to the inner ear via the stape attachment to the oval window, which is a membrane at the beginning of the cochlea. As the tympanic membrane vibrates from sound waves, the ossicles amplify that vibration, and then the oval window moves with the same vibrations. As vibrations of the tympanic membrane are transmitted through the ossicles, a wave is created within the fluid in the cochlea that displaces sections of the cochlear partition. The tube running from the oval to the round window in the cochlea is separated into two spaces. From the oval window to the tip of the cochlea the tube is referred to as the scala vestibuli and from the tip of the cochlea back to the round window it is the scala tympani. The two spaces are on either side of the scala media or also called cochlear duct, which is the space that contains the organ of Corti. The organ of Corti transduces sound into the neural signal. The organ of Corti contains hair cells with stereocilia on their apical membrane. The stereocilia bend in response to movement of the basilar membrane relative to the partially fixed tectorial membrane. Depending on which direction the stereocilia bend, they open or close ion channels, leading to signal changes in the cochlear nerve. As a standing wave is set up within the scala vestibuli and scala tympani in response to movement at the oval window, the basilar membrane responds by moving at a specific spot, dependent on the frequency of the standing wave. The cochlea encodes auditory stimuli based on frequency between 20 to 20,000 Hz, the range of human hearing. Higher frequencies cause the basilar membrane close to the base of the cochlea to move and lower frequencies cause the basilar membrane closer to the tip of the cochlea to move. Loudness is determined by the number of hair cells in an area that are stimulated. A louder sound produces greater displacement of the basilar membrane, leading to a greater number of stereocilia responding. The information sensed by the spiral organ of the cochlea is transmitted into the brain via the vestibulocochlear nerve to nuclei in the pons. At the level of the pons and related connections in the midbrain, some processing of auditory information occurs, including identifying where a sound is coming from and responding to loud noises. From the pons and midbrain, fibers carrying auditory information project to the thalamus and then, from there to the primary auditory cortex of the temporal lobe. It is here that the conscious perception of sound is located. Similar to the cochlea, the vestibular structures use hair cells with stereocilia to detect movement of fluid, in this case, in response to changes in head position or acceleration. Detection of head position when the body is stationary is termed static equilibrium. The information for static equilibrium comes from the utricle and saccule. Dynamic equilibrium is the perception of acceleration. Information for dynamic equilibrium can come from the utricle and saccule, which detect linear acceleration, and/or the semicircular canals, which detect angular acceleration. The neural signals generated from the vestibule are transmitted to the brainstem and cerebellum from sensory neurons in the vestibular ganglion. Vestibular transduction The saccule and utricle each contain a sense organ, called macula, where stereocilia and their supporting cells are found. These maculae are oriented 90 degrees to one another so that they respond to positions in different planes. The organs can respond to changes in position and acceleration because the tip of their stereocilia project into a dense otolithic membrane made up of a mixture containing granules of calcium and protein, called otoliths, which gives rise to their common name, the otolithic organs. When the otolithic organs move, gravity causes the dense otolithic membrane to move relative to the less dense cell layer beneath the stereocilia. This causes the stereocilia to bend, initiating action potentials in the vestibular nerve fibers that innervate them. Bundles of stereocilia are arranged in various directions, so that any direction of inclination will depolarize a subset of the hair cells and hyperpolarize a corresponding subset of sensory neurons. The semicircular canals are three ring-like extensions from the vestibule. One is oriented in the horizontal plane and two are in the vertical plane. The vertical canals are 45 degrees off of the sagittal plane, one is anterior and one is posterior. At the base of each semicircular canal, where it meets with the vestibule is an enlarged region known as the ampulla, which contains a hair-cell containing structure, called the crista ampullaris that responds to rotational movement. The stereocilia of the hair cells extend into the cupula, a membrane that attaches to the top of the ampulla. When the head rotates in a plane parallel to the semicircular canal, the fluid in the canal does not move as quickly as the head is moving. This pushes the cupula in the opposite direction, deflecting the stereocilia and creating a nerve impulse. Considering the semicircular canals on either side of the head, three orthogonal planes are defined, the horizontal plane with both horizontal canals, and two vertical planes 90 degrees to each other with the anterior canal from one side and the posterior canal from the other. Considering the semicircular canals on either side of the head, three orthogonal planes are defined, the horizontal plane with both horizontal canals, and two vertical planes 90 degrees to each other with the anterior canal from one side and the posterior canal from the other. Information from the vestibular apparatus projects to a wide range of structures in the brain. The information enters the brain via the vestibulocochlear nerve where it synapses at vestibular nuclei in the pons and medulla. From here, information travels to a number of other regions to stimulate reflexes and develop conscious awareness of position and movement. A yellow background with black text Description automatically generated A logo with a red and black logo Description automatically generated https://www.youtube.com/@Dr.RichardsPhysiologyNotes https://youtube.com/playlist?list=PLhQ PgS4- I3jQHpUQ32y2llTNBZoTk12Gl&si=zuXuj nsSE6EcKJox Reference Marieb E.N., Hoehn K. , Human Anatomy & Physiology. 8th Edition, Pearson International. An online version of this book is also available. The specific chapters are - Chapter 15: The Special Senses