Viral Vectors for Gene Therapy PDF
Document Details
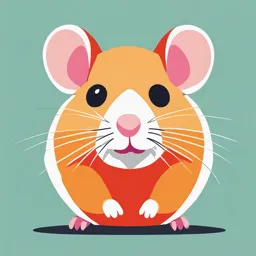
Uploaded by ValiantEmerald
C. Nóbrega et al.
Tags
Summary
This document provides a comprehensive overview of viral vectors and their applications in gene therapy. It discusses the selection of delivery systems, criteria for ideal systems, and explains the use of viral vectors in gene therapy.
Full Transcript
3 Viral Vectors for Gene Therapy The selection of the delivery system is one of the most critical points for the success (and safety) of gene therapy. First, the chosen vector must ensure that the gene is delivered to the correct target cells, or at least predominantly to those cells. Second, the...
3 Viral Vectors for Gene Therapy The selection of the delivery system is one of the most critical points for the success (and safety) of gene therapy. First, the chosen vector must ensure that the gene is delivered to the correct target cells, or at least predominantly to those cells. Second, the introduced transgene has to be activated, that is, it must go to the nucleus, be transcribed, and then translated. In a one-time cure setting, ideally the transgene should be integrated into the host genome or endure episomally, ensuring a long-term persistent expression. The ideal delivery system should meet several criteria, including (i) a good safety profile; (ii) easy production; (iii) good stability in target cells, and (iv) a high transgene capacity. As mentioned, the delivery systems can be roughly divided into two main categories: non-viral and viral systems. The non-viral methods were described in the previous chapter, whereas in this chapter the viral vectors used in gene therapy will be explained. Viruses have evolved for millions of years, aiming to efficiently introduce and replicate their genetic material in host cells. Taking advantage of this feature, gene therapy developed tools and procedures to engineer viruses and their genomes, in order to artificially deliver nucleic acids. In fact, the main advantage of viral-based systems for gene therapy is their high efficiency in delivering transgenes, surpassing anatomical and cellular barriers. For this reasons, viral vectors have been broadly used since the first gene therapy clinical trial in 1990. Data from The Journal of Gene Medicine highlights that until November 2017 around 68% of gene therapy clinical trials worldwide used viral vectors as a delivery system (Fig. 3.1) [1]. Recently, viral vectors became more than a promise in gene therapy with the approval of several gene therapy products using these delivery vectors (see Chap. 1 for a list of currently approved gene therapy products). Moreover, several other products using viral systems are in the final stages of clinical trial development [2], highlighting that new products could be approved in the next years. This broad use also emphasizes that viral vectors are now considered safe for human use, despite their natural infectious profile. Nevertheless, the safety issues were and still are major concern in the development and use of viral vectors. For that, several engineering solutions were developed trying to enhance viral vectors’ safety, without compromising their efficiency, such as (i) avoiding their replication; (ii) promoting their inactivation; and (iii) attenuating their natural toxicity. Besides their efficiency, another important advantage of viral vectors is the wide range of existing viruses, which have different features, like the type of genetic material, natural tropism, and size, which provide an enormous offer of systems for gene delivery. On the other hand, besides their safety concerns in gene therapy, viral vectors also have other disadvantages, such as their limited cloning capacity (Table 3.1).Several criteria are used to classify © Springer Nature Switzerland AG 2020 C. Nóbrega et al., A Handbook of Gene and Cell Therapy, https://doi.org/10.1007/978-3-030-41333-0_3 39 3 40 Viral Vectors for Gene Therapy Fig. 3.1 Vectors used in gene therapy clinical trials until 2017, at which time viral vectors accounted for 68% of all vectors used in these studies. Table 3.1 Main advantages and disadvantages of viral vectors used in gene therapy. Advantages High efficiency in gene transfer both in vitro and in vivo Long-term persistence (in some cases) Broad cell targets Broad range of viruses to use Natural tropism towards infection Evolved mechanisms of endosomal escape Disadvantages Potential for immune and/or inflammatory response triggering Limited cloning capacity Complex production Limited tropism to certain types of cells (in some cases) Possibility of insertional mutagenesis Molecular infection mechanisms not fully understood virus [3], including (i) the nature and form of their nucleic acid (DNA or RNA), (ii) symmetry of the capsids, (iii) presence of an envelope, (iv) the size of the virion and (v) the site of viral replication (cytoplasm or nucleus). Thus, choosing and engineering a virus for gene therapy must consider these and other features in order to ensure a successful and safe delivery of the transgene. In this section, we detail several aspects of the viral vectors most commonly used in gene therapy: lentivirus, gamma retrovirus, adenovirus, adeno-associated virus, herpes simplex virus, vaccinia, and baculovirus. 3.1 Lentiviral Vectors Lentivirus belongs to the Retroviridae family (retroviruses), which is constituted by RNAtype viruses characterized by the use of viral reverse transcriptase (RT) and the ability to insert their viral genetic information into the host genome through the action of viral integrase (IN) [4]. The name lentivirus derives from the long period between infection and the disease onset. Contrary to other retroviruses, lentivirus can also replicate in nondividing cells. They are enveloped virus, with a size ranging from 80 to 120 nm in diameter (Fig. 3.2). Lentiviruses have different host species, including primates, differing in their genome structure and entry receptors, among other features. As the majority of the lentiviral vectors used in gene therapy are based on human immunodeficiency virus 1 (HIV-1), the following descriptions are centered on this virus. HIV-1 has a single-stranded positive RNA genome with around 9 kb size, with three main open reading frames encoding for nine viral proteins (Fig. 3.3, Table 3.2) [5]. Each viral particle has two identical, positive-sense copies of the genomic RNA, each one encoding the full information needed for viral replication, although 3.1 Lentiviral Vectors 41 Fig. 3.2 Lentivirus morphology highlighting its main structural components. HIV-1 is a retrovirus with two identical copies of a single-stranded positive RNA molecule. Virions are coated by a phospholipid envelope derived from the host cell, with viral glycoproteins (gp41 and gp120) that are important for the viral entry in the cell. The viral genome is further protected by a capsid formed by p24 protein, and the virus has two additional structural proteins, p17 and p7. All these structural pro- teins are important for essential functions in the viral replicative cycle. Other components found in the virus include the reverse transcriptase, which mediates the converstion of the viral RNA genome into viral DNA; the integrase, which mediates the genomic integration of the proviral DNA into the host cell genome; and the viral protease that is responsible for processing of the gag and gag- pol polyproteins during viral maturation. recent studies suggest that both copies are required for is successful completion [6]. The viral genome is edged by long terminal repeats (LTRs), comprising each one by three regions, U3, R and U5, which are essential for transcription, reverse transcription and integration. enzymes (RT, IN, and PR). Still in the cytoplasm, the RNA molecule is reverse transcribed into a double-stranded proviral DNA molecule by the RT enzyme. This newly synthesized DNA molecule forms a complex with proteins (both viral and cellular) forming the pre-integration complex (PIC), which shuttles to the nucleus. The proviral DNA integrates into the cell genome (mediated by the IN enzyme) and starts to be transcribed into the viral mRNAs (by cellular RNA polymerase II) [8]. After some splicing events (Fig. 3.5) [9], the viral mRNAs are exported to the cell cytoplasm, where the viral proteins are produced. The viral proteins and two full-length RNA transcripts are assembled near the cellular membrane, and the viral particles (virions) are released by budding. Maturation of the viral particles occurs outside the cells. 3.1.1 Replicative Cycle The HIV-1 replicative cycle (Fig. 3.4) starts with the binding of the envelope glycoprotein gp120 to the CD4 receptor (or co-receptor CXCR4 or CCR5) of the host cell [7]. After this attachment, the viral envelope fuses with the cellular membrane, resulting in the entry of the viral core into the cell. There, the viral capsid proteins are uncoated, releasing the RNA genome and viral 3 42 Viral Vectors for Gene Therapy Fig. 3.3 Organization and main elements of a lentivirus genome, based on HIV-1. The RNA genome is about 9 kb long with three main coding segments, gag (group antigen), pol (polymerase), and env (envelope), flanked by two long terminal repeats (LTRs). Each LTR is divided into three regions, U3, R and U5, although the complete LTR sequence is only generated during reverse transcription and present in the proviral DNA. The LTRs are functional elements of the viral genome, being essential for viral transcription and therefore for the replicative cycle. The gag gene codes for structural proteins: matrix (MA), capsid (CA), nucleocapsid (NC) and transframe polypeptide (TF). The pol gene codes for the protease (PR), the reverse transcriptase (RT) and the integrase (IN). The third essential gene, env, codes for envelope proteins, the surface protein (SU) and the transmembrane protein (TM). These elements are common for all retroviruses; however, lentivirus genome is more complex and has additional elements. Tat and rev are essential regulators of the viral gene expression, coding for RNA-binding proteins that enhance transcription and act to induce late- phase gene expression, respectively. Additionally, the lentivirus genome includes four accessory regulatory elements, vif, vpr, vpu and nef, which are important for the replication and pathogenesis in vivo. 3.1.2 trials. Accounting for this use are several advantages, which are important features for gene therapy vectors, such as their mediated long-term expression or the possibility of transducing nondividing cells. Further advantages and disadvantages of their use in gene therapy applications are summarized in Table 3.3. rom Lentivirus to Lentiviral F Vectors Despite being based on HIV-1, lentiviral vectors have been used for the past years as an effective and safe system to deliver genes both in gene therapy-based preclinical studies and in clinical 3.1 Lentiviral Vectors 43 Table 3.2 Coding segments of retroviruses and their functionality. Common to all retrovirus Gene Functionality Protein gag Structural Capsid protein (CA) Matrix protein (MA) Nucleoprotein (NC) pol Replication Protease (PR) Reverse transcriptase (RT) RNase H vpr Integrase (IN) Surface glycoprotein (SU) Transmembrane protein (TM) Transactivator protein RNA splicing regulator Negative regulating factor Viral infectivity protein Virus protein r vpu Virus protein unique env Specific of complex lentivirus tat Envelope Regulatory rev nef vif Accessory Since the first use of lentiviral vectors in gene therapy, several modifications have been introduced in order to increase safety, without compromising efficiency [10]. Because lentiviruses have high rates of mutation and recombination, even the vector production could be dangerous, especially with the possibility of generating replication-competent lentiviruses (RCLs). One of the developed strategies to increase lentiviral vector safety production, trying to overcome the RCL issue, was to separate the essential lentivirus genes in different plasmids and delete some accessory genes. This strategy is the main difference between the four generations of lentiviral vectors that were developed in the last years (Fig. 3.6) [11], although many other features and alterations can be found inthe different generations (Table 3.4). Another important advance in lentiviral vectors’ development for gene therapy applications was the pseudotyping engineering, in which non-lentiviral envelope proteins are Function Formation of the capsid Formation of the inner membrane layer Formation of the nucleoprotein/RNA complex Proteolytic cleavage of gag precursor protein; release of structural proteins and viral enzymes Transcription of viral RNA into proviral DNA Degradation of viral RNA in the viral RNA/DNA replication complex Integration of proviral DNA into the host genome Attachment of virus to the target cell Anchorage of gp120, fusion of viral and cell membrane Activator of transcription of viral genes Regulation of the export of non-spliced and partially spliced viral mRNA Enhancement of infectivity of viral particles Critical for infectious virus production in vivo Component of virus particles, interaction with p6, facilitates virus infectivity Efficient virus particle release, control of CD4 degradation, intracellular trafficking modulation used in the process of producing lentiviral vectors. For example, one of the most popular alterations is the replacement of the native Env glycoprotein with the viral VSV-G protein, from the vesicular stomatitis virus envelope glycoprotein G. This modification increased viral stability, which permitted improvements in production and an increase in the viral titer yielded. Moreover, this protein has a ubiquitous receptor, which allowed the targeting of a much broader set of cells. First Generation Replication-deficient lentiviral vectors of the first generation are produced using three different plasmids: (i) the packaging construct, containing all the essential genes (except the env gene) and all the regulatory and accessory proteins, (ii) an env plasmid encoding a viral envelope glycoprotein, and (iii) a transfer vector containing the desired transgene and all the essential cis-acting 44 3 Viral Vectors for Gene Therapy Fig. 3.4 Main phases of the replicative cycle of a lentivirus, based on HIV-1 cycle. Viral particle entry into the host cell is mediated by the gp120 glycoprotein of the envelope that binds to cell receptor CD4 (1). This leads to a conformational alteration in TM (gp41) that facilitates the fusion of the virus with the cellular membrane (2). The viral core enters the cell cytoplasm, where uncoating and release of the viral RNA takes place (3). The next step is the reverse transcription of the viral RNA into proviral DNA, mediated by the RT reverse transcriptase (4). After this step, the pre-integration complex (PIC) is assembled, being composed of the RT, IN, Vpr, MA and the proviral DNA. This complex is transported through the actin microfilaments and enters into the nucleus through the nuclear pore complex (5). Inside the nucleus, the proviral DNA is integrated into the cell genome, through the action of the IN integrase (6). After integration, the proviral DNA is normally transcribed by RNA polymerase II, and the transcription of viral genes is mediated by the U3 region of the 5’LTR (7). A single viral transcript is formed, some copies will eventually be packed into newly formed viral particles, but others will serve as template for the translation of viral proteins. Therefore, in the latter case single transcripts undergo splicing to generate the different viral mRNA segments, which are then exported from the nucleus (8). In the cytoplasm, the viral mRNAs are translated into different viral proteins, which are formed from precursor peptides that undergo cleavage by viral and cellular proteases (9). The assembly of the viral particles occurs in the cellular membrane and initiates with the interaction between precursor structural proteins and the packaging signal present in the unspliced viral RNA (10). The next stages are viral budding and release, wherein viral particles emerge from the cell surface, coated by portions of the cellular membrane containing the viral glycoproteins that were produced in the endoplasmic reticulum (11, 12). Finally, outside the cells the viral particles mature through cleavage of the viral polyproteins into individual proteins and reorganization of the structural proteins into the final viral arrangement (13). elements (LTRs, Ψ, RRE). The first two plasmids do not have the packaging signal (Ψ) or any LTRs to prevent their inclusion in the produced vector particles. Second Generation Despite the important advance provided by the three-system plasmid of the first generation, some safety issues were still important as RCLs 3.1 Lentiviral Vectors 45 Fig. 3.5 Lentivirus mRNA splicing transcripts, based on the HIV-1 genome. The integrated proviral DNA is transcribed into a single mRNA transcript of approximately 9 kb size. This single transcript is then spliced into different transcripts, which can be grouped into three main clusters: (i) the primary mRNA that codes for the Gag-Pol polyprotein; (ii) intermediate mRNAs (~4 kb long) resulting from the splicing of the primary transcript, which code for polyprotein Env or accessory proteins Vif, Vpr and Vpu; and (iii) short transcripts (~2 kb long) resulting from additional splicing events and yielding mRNAs that code for the Tat, Rev and Nef proteins. Table 3.3 Main advantages and disadvantages of lentiviral vectors for gene delivery. could still be generated. To further increase safety, in the second generation of lentiviral vectors, the accessory genes (vif, vpu, vpr, nef) were deleted while maintaining the number of plasmids. This modification did not negatively affect vector titer or infectivity, but drastically reduced the chance of generating RCLs. Advantages Transduction of dividing and nondividing cells Integration into the host genome Long-term expression Possibility for no integration Cloning capacity of around 8 kb Low immunogenicity Disadvantages Need for pseudotyping Possible generation of replication-competent lentivirus (RCLs) Insertional mutagenesis Safety problems due to the presence of viral regulatory proteins Third Generation The next advance in lentiviral vectors brought the elimination of an essential gene (tat) and the introduction of the rev gene in a fourth plasmid. The need for Tat protein was overcome by replacing the U3 region in the 5’LTR by a strong heterologous promoter, such as the CMV (cyto- 46 Fig. 3.6 Different lentiviral vector generations used in gene therapy and their modifications engineered from the wild-type HIV-1. The first generation of lentiviral vectors was produced using three plasmids encoding the viral proteins. In these vectors, only the transgene contained the essential cis-acting elements (LTRs, Ψ, RRE) that allowed their packaging in the produced lentiviral particles. In the second generation of lentiviral vectors, the number of plasmids was maintained, but in order to improve their safety the accessory genes were deleted. In the third gen- 3 Viral Vectors for Gene Therapy eration, the tat gene was eliminated and the viral genome divided into an additional plasmid containing the rev gene. This also included the deletion of the U3 region of the 3’LTR, generating self-inactivating virus. Finally, a fourth generation of lentiviral vectors was developed to further reduce the risk of recombination between the plasmids used. For that, the segments gag and pol were codon optimized. In this fourth generation, all the modifications of the previous generations were maintained. 3.1 Lentiviral Vectors 47 Table 3.4 Main features of the different generations of lentiviral vectors Constructs Packaging plasmids Accessory genes tat and rev genes gag and pol genes 3’LTR deletion Codon optimization First generation 3 1 Second generation 3 1 Yes Same plasmid Same plasmid No No No Same plasmid Third generation 4 2 Fourth generation 4 2 Same plasmid No tat absent, rev in a separate plasmid Same plasmid No tat absent, rev in a separate plasmid Same plasmid No No Yes No Yes gag and pol genes Table 3.5 Main advantages and disadvantages of third generation lentiviral vectors. Advantages Able to transduce slowly dividing and nondividing cells Delivered transgenes are more resistant to transcriptional silencing Suitable for several ubiquitous or tissue- specific promoters Safety increased by self-inactivation Disadvantages Production of high titers is more difficult Still some possibility of generating replication- competent lentiviruses megalovirus) promoter. The addition of a new plasmid, separating the rev gene, further increased the safety of the viral vectors production by reducing the possibility of RCL generation. An additional improvement was also added to these lentiviral vectors with the deletion of part of the U3 region in the 3’LTR, generating SIN (self-inactivating) vectors, thus decreasing the risk of activating nearby genes in the integration process. These lentiviral vectors have only three of the nine wild-type HIV-1 genes, which significantly increases their safety profile. The four constructs used for their production are (i) a packaging plasmid with the gag and pol genes; (ii) a plasmid with rev gene, (iii) a plasmid with the env (or VSV-G or other pseudotyped protein), and (iv) a plasmid with the transgene (and a strong heterologous promoter). Fourth Generation The potential formation of RCLs using the third generation lentiviral vectors is very reduced, however, theoretically, it is still possible that homologous recombination between the transfer and the packaging plasmids takes place. To solve this problem, codon optimization was implemented in the gag and pol genes, thus eliminating the homology between plasmids. This fourth generation of lentiviral vectors displays improved biosafety; however, viral titers were negatively affected. Maybe because of this, it has not been extensively used, and the previous lentiviral vector generations are still more commonly applied, especially the third generation due to its important advantages (Table 3.5). 3.1.3 Additional Improvements to Lentiviral Vectors Several other improvements in lentiviral vector design were introduced in the different generations to improve efficiency, to facilitate their production or, as already mentioned, to improve their biosafety (Fig. 3.7) [12]. One of those improvements was the introduction of a cis- acting polypurine tract (cPPT), to increase the viral vector transduction efficiency both in vitro and in vivo. Another strategy, improving lentiviral vector expression, was the introduction of the woodchuck hepatitis virus post- 48 3 Viral Vectors for Gene Therapy Fig. 3.7 Modifications introduced to lentiviral vectors relative to the wild-type HIV-1 virus, in order to improve their safety profile while maintaining their efficiency and production in high titers. transcriptional regulatory element (WPRE), which increases the number of unspliced RNA molecules, leading to an increase of the transgene expression in target cells. One important issue resulting from lentiviral vector-mediated integration is the possibility of transgene expression repression, due, for example, to epigenetic events. To address this problem, chromatin-insulator sequences were introduced in the lentiviral vectors. Insulators have the ability to block enhancer-promoter interactions and/or serve as barriers against silencing effects. The further engineering of lentiviral vectors also included the fusion of proteins or antibodies to envelope glycoproteins to alter their tropism and retarget the vectors to specific cells. Another commonly used strategy to direct and specify transgene expression is to include a tissue-specific promoter in the transgene construct. This strategy will not select the cells to transduce, but rather limit the expression of the transgene to a certain cell type due to the presence of the specific promoter. Limiting expression of the transgene to particular cells is particularly useful in the context of the central nervous system, where the presence of different cells types makes specific transduction difficult. SIN Vector Design Another feature included in most of the lentiviral vectors is the inclusion of a self-inactivating long terminal repeat (SIN-LTR), in which the U3 3.1 Lentiviral Vectors Fig. 3.8 Self-inactivating lentiviral vectors. In wild- type HIV-1 virus, the viral RNA is transcribed under the control of an enhancer-promoter sequence that is located in the U3 region of the LTRs, being required for the production of new viral particles and the continuation of the replicative cycle. During the process of reverse transcription 49 the U3 region of the 3’LTR is transposed to the 5’LTR. In self-inactivating vectors, the U3 region of the 3’LTR is partially deleted in order to block its enhancer-promoter function. Upon integration, the proviral DNA will harbor the partially deleted U3 region in both LTRs, thus preventing the further continuation of the replicative cycle. region in the 3’LTR has been partially deleted deficient), this region is dispensable, as the pack(Fig. 3.8) [13]. This feature reduces the risk of aged RNA expression is driven by the 5’LTR lentiviral vector recombination with wild-type region and the transgene expression by the respecviruses, thus increasing its safety. In the wild- tive promoter. The partially deleted U3 region type HIV-1, the U3 region acts as a viral enhancer/ will be transfered to both the 5’ and 3’LTR promoter, being essential for viral replication, regions during integration, so that any viral parespecially for the formation of both the 5’ and ticle progeny that is formed will harbor two 3’LTR of new viral particles. However, in the inactivated LTRs and transgene expression will recombinant lentiviral vectors (replicative- be only driven by the internal promoter. 3 50 Non-integrative Lentiviral Vectors In the context of gene therapy, one of the main advantages of using lentiviral vectors is their ability to stably integrate the desired transgene in the target cells genome. However, integration can lead to the development of undesired consequences, such as the activation of oncogenes. In fact, for some applications the development of non-integrative vectors would still be effective, having as an additional benefit a better safety profile [14]. To attain this goal, lentiviral vectors were designed containing mutations in regions that are essential for the proviral DNA integration process, namely in, (i) the U3 region of the 5’LTR, (ii) the U5 region of the 3’LTR, and (iii) the integrase protein itself. Lentiviral Vector Pseudotyping As mentioned, the inclusion of different glycoproteins into the envelope of lentiviral vectors alters and increases the variety of target cells that can be transduced, thus improving their tropism. Like it was referred, pseudotyping is the process of producing viral vectors (or virus) containing envelope proteins from a different virus [15]. Several glycoproteins from different virus have been used and studied to pseudotype lentiviral vectors providing different advantages, such as increasing their efficiency or reducing their toxicity (Table 3.6). 3.1.4 Lentiviral Vector Production Any delivery system used for gene transfer, either non-viral or viral, has to be produced/built preferentially with a safe and simple method of low technical complexity that, of course, yields the system in high amounts. In the case of lentiviral vectors, the safety issue was a major driving force in the development of production methods and the main reason why the viral genome was separated into different constructs. However, this important advance brought a new challenge, as all the constructs must join together in the factory cells to produce effective lentiviral vector particles. To accomplish this, two transfection methods are used: transient transfection [16] and Viral Vectors for Gene Therapy Table 3.6 Common viral glycoproteins used for lentiviral vector pseudotyping. Species/envelope Vesicular stomatitis virus (VSV-G) Feline endogenous retrovirus (RD114) Ebola Rabies Lymphocytic choriomeningitis virus (LCMV) Ross River virus Influenza virus hemagglutinin Moloney murine leukemia virus 4070 envelope Advantages Wide tropism Facilitates production using ultracentrifugation More efficient and less toxic than VSV-G in hematopoietic cells Efficiently transduces airway epithelium Retrograde transport in neuronal axons Low toxicity Transduces hepatocytes, glial cells, and neurons Transduces airway epithelium Able to transduce most cells stable transfection [11]. In the first approach, all the plasmids (three or four, depending on the generation used) are simultaneously transiently transfected into a packaging cell line (Fig. 3.9). Several transfection agents cab be used, like calcium phosphate, polyethylenimine (PEI) or cationic lipids. The second approach involves the use of stable packaging cell lines already containing one or more viral genes, thus reducing the number of plasmids to be transfected [4]. Both approaches have advantages and drawbacks, and their choice is mainly dependent on the laboratory conditions, on the safety regulations and on the viral titer needed. Other important issues to be considered in the production of lentiviral vectors include the method of viral particle purification and concentration, the procedure for titer assessment, the choice of producer cell line and the production scale (Fig. 3.10). For research purposes, the production of lentiviral vectors is well-established in human embryonic cells kidney 293 (HEK293) or HEK293T adherent cells using transient transfection methods. However, for clinical trials, the quantity and quality of the vectors needed are 3.1 Lentiviral Vectors Fig. 3.9 Plasmids used to produce lentiviral vectors in different vector generations. Depending on the generation, lentiviral vectors can be produced by transfecting three or four plasmids into producing cells. Alternatively, 51 a stable cell line expressing one or two viral genes could be used in the production, thus reducing the number of plasmids to be transfected. 52 3 Viral Vectors for Gene Therapy Fig. 3.10 Overview of the main steps of lentiviral vector production. Several methods can be used to transfect viral plasmids, including delivery mediated by liposomes. The produced viral particles can be concentrated and purified from the producing cells using different techniques, among which ultracentrifugation is the most common. Finally, several methods to quantify the lentiviral vectors titer can be used, although the quantification of p24 is the most common. much higher. Thus, large- scale methods were developed using both adherent and suspension cell cultures. The process is quite expensive and complex, and only a few sites in Europe and the USA are able to produce GMP lentiviral vector particles in large scale [11]. Concentration and purification methods are both very important in the production of lentiviral vectors and are closely related to the production scale needed (research or clinical trial). Even though, probably the method most commonly used for concentration is the centrifugation of culture medium after an initial filtration. This method can also be combined with anion- exchange chromatography for an additional purification step. However, these methods are not suitable for large-scale productions and in vivo application, as often they are not free from culture medium/process contaminants. Therefore, in large-scale productions, several methods are combined to achieve three main goals: (i) an initial purification of the vectors; (ii) an intermediate purification to remove specific impurities, and (iii) final refining to remove trace contaminants. Finally, the last step in the production of lentiviral vectors, which is the assessment of the viral production titer, is also very important, in order to adjust vector doses and to evaluate the transduction efficiency. There is a wide range of methods used for this purpose, for example (i) measuring the quantity of one vector component (commonly the viral p24 protein is used); (ii) measuring the number of provirus DNA copies in infected cells; or (iii) measuring transgene or reporter gene expression in infected cells. 3.2 Gamma Retrovirus 53 Several modifications and improvements are continuously being tested aiming to increase lentiviral vector quality and quantity and also to increase safety in the production process. 3.1.5 Lentiviral Vectors in Clinical Trials There is already one gene therapy product approved in Europe and the USA based on the delivery by lentiviral vectors. Kymriah® is an ex vivo gene therapy delivering CD19-specific CAR T-cells, indicated for the treatment of acute lymphoblastic leukemia. Due to their important advantages, lentiviral vectors were and are used with success in several clinical trials, particularly for rare diseases. More recently, they were also applied in gene therapy studies for more frequent genetic and acquired diseases, including β-thalassemia, Parkinson’s disease and cancer. Tables 3.7 and 3.8 detail two examples of gene therapy clinical trials using lentiviral vectors [17, 18]. 3.2 Gamma Retrovirus Gamma retrovirus also belongs to the Retroviridae family (retroviruses), like the lentivirus. However, their genome organization is simpler [19], which makes its genetic engineering easier than for lentivirus (Fig. 3.11). As vectors for gene therapy, they share more or less the same advantages and disadvantages of the lentivirus. However, contrary to those, the gamma retrovirus only infects dividing cells, and maybe for that, currently, they are less used in gene therapy than lentivirus [20]. Moreover, lentiviral vectors seem to be safer than gamma retrovirus in relation to the risk of insertional mutagenesis. The gamma retrovirus was mainly used in the earlier gene therapy studies, until the previously mentioned incident with a X-linked severe combined immunodeficiency (SCID) trial (Table 3.9) [21] where insertional oncogenesis was observed in some of the trial subjects. Table 3.7 Example 1 of a gene therapy clinical trial using lentiviral vectors as the delivery system of the therapeutic gene. Study Disease Therapeutic gene Delivery vector Clinical trial Inclusion criteria Type of administration Clinical outcome Palfi, S., et al. (2014) Long-term safety and tolerability of ProSavin, a lentiviral vectorbased gene therapy for Parkinson’s disease: a dose escalation, open-label, phase 1/2 trial, Lancet 383, 1138–1146 Parkinson’s disease, which is a common neurodegenerative disease mainly characterized by motor impairments, resulting from the progressive degeneration of dopaminergic neurons in the substantia nigra that project axons to the striatum, where dopamine is released. ProSavin (genes encoding key enzymes in the dopamine metabolism, tyrosine hydroxylase, AADC, and cyclohydrolase 1) in three doses (low 1.9 × 107 TU; mid 4.0 × 107; and high 1 × 108) Lentiviral vector (based on equine infectious anemia virus) produced by triple transient transfection methods in HEK293T cells, purified and concentrated by anion-exchange chromatography and hollow fiber ultrafiltration Phase I/II (long-term safety and tolerability), open-label and 12-month follow-up study, in France and the UK 48–65 years; disease manifestation for 5 or more years and 50% or higher motor response to oral dopamine treatment Local delivery of ProSavin bilaterally into the striatum Despite some adverse events, the study found that ProSavin was safe and well tolerated. No detectable antibody response against any of the ProSavin transgene products was detected. There was an improvement in motor behavior in all the treated patients, and 11 of the patients had a reduction in the daily levodopa administration at 6 and 12 months after the therapy. 3 54 Viral Vectors for Gene Therapy Table 3.8 Example 2 of a gene therapy clinical trial using lentiviral vectors as the delivery system of the therapeutic gene. Study Disease Therapeutic gene Delivery vector Clinical trial Inclusion criteria Type of administration Clinical outcome Cartier, N., et al. (2009) Hematopoietic stem cell gene therapy with a lentiviral vector in X-linked adrenoleukodystrophy, Science 326, 818–823 X-linked adrenoleukodystrophy (ALD), which is a fatal demyelinating disease of the CNS caused by mutations in the ABCD1 gene that codifies for ALD protein, an adenosine triphosphate-binding cassette transporter localized in the peroxisomes membrane. Most of the affected boys die before reaching adolescence. Wild-type ABCD1 gene under the control of the MND (myeloproliferative sarcoma virus enhancer, negative control region deleted, dl587rev primer binding site replaced) promoter. Re-infusion of 4.6 × 106 and 7.2 × 106 cells per kilogram, for patient 1 and patient 2, respectively Replication-defective HIV-1-derived self-inactivating lentiviral vector (CG1711 hALD), in a concentration of 941 × 107 infectious particles/ml Interventional study in France Two ALD patients (aged 7.5 and 7 years) with progressive demyelination and no matched donors Ex vivo lentiviral-mediated transfer of the ABCD1 gene into CD34+ cells from ALD patients No adverse events were reported. Hematopoietic recovery occurred at days 13–15 after transplant and was sustained thereafter. Nearly complete immunological recovery occurred between 9 and 12 months. The progression in cerebral demyelination was stopped at 12–14 months after gene therapy for patient 1 and at 16 months for patient 2. A follow-up at 36 months revealed that no changes in the extent of cerebral demyelinating lesions were observed. Fig. 3.11 Organization of a gamma retrovirus genome, based on the murine leukemia virus (MLV). The RNA molecule is about 8.3 kb long with the three retroviral main coding segments gag (group antigen), pol (polymerase), and env (envelope), flanked by two long terminal repeats (LTRs). Contrary to lentiviruses, the gamma retrovirus genome does not have additional coding segments. The gag gene codes for structural proteins: matrix (MA), capsid (CA), nucleocapsid (NC), and p12. The pol gene codes for the protease (PR), the reverse transcriptase (RT) and the integrase (IN). The third essential gene, env, codes for envelope proteins, the surface protein (SU) and the transmembrane protein (TM). 3.3 linear DNA molecule as genetic material. The viral capsid has icosahedral symmetry (20 facets) a diameter of 70–100 nm and each icosahedron is composed of 240 proteins, named hexons (Fig. 3.12) [22]. Adenoviral Vectors Adenovirus belongs to the Adenoviridae family, comprising more than 100 viruses able to infect humans and several animal species. They are non-enveloped viruses, with a double-stranded 3.3 Adenoviral Vectors 55 Table 3.9 Example of a gene therapy clinical trial using gamma retroviral vectors as the delivery system of the therapeutic gene. Study Disease Therapeutic gene Delivery vector Clinical trial Inclusion criteria Type of administration Clinical outcome Cavazzana-Calvo, M., et al. (2000) Gene therapy of human severe combined immunodeficiency (SCID)-X1 disease, Science 288, 669–672 Severe combined immunodeficiency-X1 (SCID-X1) is an autosomal recessive disease caused by mutations in the IL2RG (interleukin 2 receptor subunit gamma) gene, which encodes for a critical protein of the immune system. Wild-type IL2RG cDNA introduced in CD34+ autologous cells Moloney retrovirus-derived defective vector Interventional study, with a follow-up of 10 months in France Two patients with 8 and 11 months Ex vivo gene therapy mediated by gamma retrovirus After 10 months, transgene expression was detected in T- and NK-cells in both patients. This was accompanied by clinical improvement in both patients, leading to normal development without any further treatment. Fig. 3.12 Adenovirus morphology highlighting the icosahedral symmetry of the capsid. The genome of adenovirus consists in a double-stranded linear DNA molecule, with a terminal protein in each side of the helix, which act as primers for viral replication. They are non- enveloped virus, with a diameter of 70–100 nm, and each icosahedral capsid is composed of 240 proteins, named hexons. Each of the 12 vertexes is formed by another protein, named penton, constituting a base for a projecting fiber, which is important for the viral attachment to the host cell. The extremity of the fiber terminates with a globular domain, named knob. The adenovirus genome size is around 34–43 kb, flanked by two inverted terminal repeats (ITRs), which act as the DNA replication origin. The genome encodes for approximately 35 proteins, generally expressed in 2 phases from 7 transcription units, which are mostly transcribed by RNA polymerase II (Fig. 3.13, Table 3.10): (i) four early transcriptional units (E1, E2, E3, and E4), which are important for the early infection stage ensuring the control of the host cell and DNA replication, (ii) two delayed early transcriptional units (IX and IVa2) that are important after replication, and (iii) one late transcriptional unit (MLTU, subdivided into L1, L2, L3, L4, and L5), mainly responsible for viral assembly, and release of the viral particles from the host cells [23]. 3 56 Fig. 3.13 Organization of the adenovirus genome, based on serotype 5. The double-stranded linear DNA molecule has 36 kb long, flanked by an inverted terminal repeat (ITR) in each extremity, and coding for approximately 35 proteins. The different coding segments are grouped, according to their transcription, in early tran- 3.3.1 Replicative Cycle Viral Vectors for Gene Therapy scriptional units (E1, E2, E3, and E4), which are activated upon infection; delayed early transcriptional units (IX and IVa2), encoding for capsid proteins; and a late transcriptional unit (MLTU), which encodes for structural proteins and is also important for virus assembly and release from the host cell. 4. Targeting of the nucleocapsid to the nucleus of the host cell. The adenovirus replicative cycle comprises dif- 5. Viral gene expression, whereby viral mRNAs ferent stages (Fig. 3.14): are transcribed and viral proteins are synthesized. 1. Adsorption, which initiates viral entry into the 6. Viral genome replication. host cell and is mediated by the surface recep- 7. Virion assembly/maturation, through tor CAR (coxsackievirus and adenovirus which the viral genome and proteins are receptor). assembled and packaged in the cytoplasm. 2. Internalization of the virus, via clathrin- 8. Release of the new infectious virus; the newly dependent endocytosis. formed virions accumulate in the cell and are 3. Partial uncoating and escape from the endoreleased upon cell lysis [22]. some; the remaining capsid structures carry the genome to the nucleus. 3.3 Adenoviral Vectors Table 3.10 Adenovirus transcription units and their main functions. Transcription unit Function E1 Activates transcription of cellular and viral genes; induces S phase of the cell; inhibits cell apoptosis E2 Codes for proteins essential to viral DNA replication E3 Codes for proteins blocking the natural cell response to infection E4 Codes for proteins involved in DNA replication, mRNA transport, and splicing IX Activates late gene expression IVa2 Important for the activation of late promoter MLTU Codes for structural capsid proteins; promotes viral particles assembly and genome packaging Fig. 3.14 Adenovirus replicative cycle. The cycle begins with the attachment of the virus to the host cell receptors, mediated by the fiber (1), and after that the virus is internalized in clathrin-coated vesicles (2). The next steps are the escape from the endosome, the partial 57 3.3.2 From Adenovirus to Adenoviral Vectors So far, adenoviral vectors have been the most commonly used viral delivery system in gene therapy and were actually the vector chosen in the first gene therapy clinical trial. Some of their important advantages have contributed to this situation, including an easy production and high expression levels of the transgene (Table 3.11). On the other hand, one important disadvantage of their application is the pre- existing immunity (which could limit the therapeutic efficacy), as 80% of the human adult population has been naturally exposed to adenovirus serotypes 2 and 5, which are the most common serotypes in which adenoviral vectors production is based. uncoating of the viral capsid (3), and the targeting of the nucleocapsid to the nucleus (4). Next, the viral genes are transcribed (5) and the viral DNA is replicated (6). Viral assembly starts in the cell nucleus (7) and culminates with cell lysis and the release of the viral particles (8). 3 58 Table 3.11 Main advantages and disadvantages of adenoviral vectors for gene delivery. Advantages Transduction of dividing and nondividing cells Cloning capacity of up to 8 kb No integration Disadvantages Transient expression of the transgene High levels of pre-existing immunity Stimulation of a strong immune and inflammatory response High expression levels of the transgene Production at high titers Suitable as oncolytic vector Broad host range Efficient transduction As was the case for lentiviral vectors, the development of adenoviral vectors was improved over time, with the introduction of several modifications leading to the existence of three different classes of adenoviral vectors: first generation, second generation and third generation, or gutless, vectors (Fig. 3.15) [24]. In the first generation of adenoviral vectors, the main goal was to produce non-replicative and therefore safer vectors. For that, E1 or both the E1 and E3 regions were removed, providing space for the transgene cassette. This resulted in non-replicative vectors, but the presence and expression of the remaining viral genes still stimulated a strong inflammatory and immune response. To avoid this problem, the second generation of adenoviral vectors was envisioned with the deletion of additional genome regions and allowing an increase in the cloning capacity, of up to 14 kb. However, these second- generation adenoviral vectors still induced some toxicity due to the immunogenic and inflammatory potential of the remaining viral genes. Finally, a third generation of adenoviral vectors, also called gutless or helper-dependent, was developed, being characterized by the complete deletion of the viral genome, with exception of the two ITRs and the packaging signal. Gutless adenoviral vectors have some advantages, even when compared to Viral Vectors for Gene Therapy first-generation adenoviruses, which make them even more suitable as a gene therapy vector: (1) they are devoid of viral genes, which increases their safety profile; (2) they have an increased loading capacity of up to 30 kb, which allows carrying of larger genes; and (3) they have an easy production in high titers, which facilitates the translation to human trials and applications. However, as they lack all viral genes, during their production the proteins needed for genome replication, packaging and capsid formation have to be provided by a helper vector [25]. 3.3.3 Adenoviral Vector Modifications The death of Jesse Gelsinger in 1999 during a gene therapy trial revealed one of the critical problems of adenoviruses as vectors, their immunogenicity, which results from the activation of the innate immune response, the cellular immune response and the humoral immune response [26]. To overcome this situation, several modifications were introduced, many of which actually having served as a basis for the development of the different generations of adenoviral vectors described. The wide variety of modifications that were developed had two main goals: (i) overcoming the inflammation response to viral replication and (ii) overcoming the innate immune response, which is dose-dependent. In the same line, the strategies addressing these problems can be organized into two main approaches: genetic modifications and chemical/ nonchemical modifications. The genetic modifications are based on the depletion of viral genes and led to the development of the different generations of adenoviral vectors. The chemical and nonchemical modifications are based on the shielding of the viral capsid with different materials (e.g., PEI, polyethylene glycol; HPAM, N-[2-hydroxypropyl]), to prevent its interaction with specific immune receptors. Other modifications were also introduced in adenoviral vectors in order to alter the vector tropism, including (i) capsid pseudotyping, (ii) serotype switching or (iii) binding with antibodies. 3.3 Adenoviral Vectors Fig. 3.15 Adenoviral vector generations developed for gene therapy applications. The first generation of adenoviral vectors was produced by deleting E1 or both E1 and E3 units, aiming to develop non-replicative vectors and providing cloning capacity for the transgene. The E1-deleted vectors have a cloning capacity of around 5 kb, while the E1- and E3-deleted vectors have a cloning capacity of up to 8 kb. While these vectors fail to replicate in vitro, the presence of several other viral genes stimulates a strong immune and inflammatory response. Therefore, a second generation of adenoviral vectors was developed, further 59 deleting E2 and E4 regions, providing a cloning capacity of around 14 kb. However, these second-generation adenoviral vectors still induced some toxicity, also due to the presence of viral genes. So, a third generation of adenoviral vectors was developed based on the complete deletion of the viral genome, except for the ITRs and the packaging signal. These were named adenoviral gutless vectors, having a cloning capacity of up to 30 kb and a safer profile, although their production is more complex, as all the viral components are absent. 3 60 3.3.4 Adenoviral Vector Production As mentioned above, the different generations of adenoviral vectors were developed aiming to reduce vector toxicity, mainly by deleting viral genes. However, these genes are crucial for viral replication and thus, in a viral production setting, the producer cells must be provided with the deleted elements. Normally, for the first and second generations of adenoviral vectors, the packaging cell lines used include HeLa, A549, HEK293 and PER.C6 [27]. In the case of gutless adenoviral vectors, as they lack all of the viral genes, there is the need to supply all the viral components for replication. This is achieved by the coinfection of the producer cell line with the gutless vector and a helper adenovirus (Fig. 3.16) [25]. One important disadvantage in the production of these vectors is the production of a mixed population of viral particles, derived from both the gutless vector and the helper virus. Thus, additional strategies were designed trying to separate the viral particles; however, residual contamination of the helper virus is detected in almost all of the production batches, which could lead to potential toxicity events. For example, the use of Cre or other recombinases to excise the packaging signal of the helper virus reduced the contamination by these viruses to 0.1–10%, compared to the gutless vectors [28]. Another important limitation of gutless vector production is their lower titers compared to previous generations of adenoviruses. In terms of human applications, besides the helper contamination, large-scale production is also very complex, less efficient, and also less safe, as several successive coinfections with the helper virus are needed. 3.3.5 Adenoviral Vectors in Clinical Trials Since the first gene therapy clinical trial, adenoviral vectors were in the first line as a choice vector for delivering genes. Despite several drawbacks regarding their use, their easy production, versatility and suitability as oncolytic vec- Viral Vectors for Gene Therapy tors ensure the first place among viral vectors that are most commonly used in gene therapy clinical trials (Table 3.12) [29, 30]. 3.4 Adeno-associated Virus (AAV) Adeno-associated viruses (AAVs) are helper- dependent viruses of the Dependovirus genus of the Parvoviridae family, which includes virus infecting several mammal species, including humans. AAVs are non-enveloped small virus (18–25 nm), having a capsid with icosahedral symmetry, composed of 60 proteins (Fig. 3.17) [31]. The AAV genome is composed of a single- stranded DNA molecule of around 4.7 kb, comprising two open reading frames (ORFs), rep and cap, flanked by inverted terminal repeats (ITRs) that form hairpin structures important for replication and packaging events (Fig. 3.18). Three viral promoters have been identified: p5, p19, and p40. The rep ORF encodes for four nonstructural proteins involved in replication (Rep78, Rep68, Rep52 and Rep10), whereas the cap ORF encodes for three structural proteins of the capsid (VP1, VP2, and VP3) [31, 32]. Over the last years, 12 different AAV serotypes have been isolated and identified, and more than 100 variants have been isolated from humans and other primates. The AAV serotype is defined by capsid protein motifs that are identified by different neutralizing antibodies and that provide differences in viral features, such as the type of cells/tissues the virus infect or the main receptors they use to enter the cells (Table 3.13) [33]. Genome organization is conserved across different AAV serotypes, although there are differences in their transcription profiles [31]. 3.4.1 Replicative Cycle The AAV replication cycle starts with the binding of the virus to a specific cell receptors and its internalization through receptor-mediated endocytosis. After cell entry, the virus needs to escape from the endosome, enter the nucleus through the