Viral Vectors for Gene Therapy PDF
Document Details
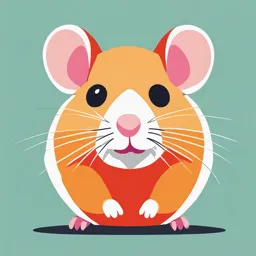
Uploaded by ValiantEmerald
C. Nóbrega et al.
Tags
Summary
This document discusses the use of viral vectors in gene therapy. It details the selection process, safety concerns, and advantages of viral vectors. The document also explores the various types of viral vectors commonly used in gene therapy research.
Full Transcript
3 Viral Vectors for Gene Therapy The selection of the delivery system is one of the most critical points for the success (and safety) of gene therapy. First, the chosen vector must ensure that the gene is delivered to the correct target cells, or at least predominantly to those cells. Second, the...
3 Viral Vectors for Gene Therapy The selection of the delivery system is one of the most critical points for the success (and safety) of gene therapy. First, the chosen vector must ensure that the gene is delivered to the correct target cells, or at least predominantly to those cells. Second, the introduced transgene has to be activated, that is, it must go to the nucleus, be transcribed, and then translated. In a one-time cure setting, ideally the transgene should be integrated into the host genome or endure episomally, ensuring a long-term persistent expression. The ideal delivery system should meet several criteria, including (i) a good safety profile; (ii) easy production; (iii) good stability in target cells, and (iv) a high transgene capacity. As mentioned, the delivery systems can be roughly divided into two main categories: non-viral and viral systems. The non-viral methods were described in the previous chapter, whereas in this chapter the viral vectors used in gene therapy will be explained. Viruses have evolved for millions of years, aiming to efficiently introduce and replicate their genetic material in host cells. Taking advantage of this feature, gene therapy developed tools and procedures to engineer viruses and their genomes, in order to artificially deliver nucleic acids. In fact, the main advantage of viral-based systems for gene therapy is their high efficiency in delivering transgenes, surpassing anatomical and cellular barriers. For this reasons, viral vectors have been broadly used since the first gene therapy clinical trial in 1990. Data from The Journal of Gene Medicine highlights that until November 2017 around 68% of gene therapy clinical trials worldwide used viral vectors as a delivery system (Fig. 3.1) [1]. Recently, viral vectors became more than a promise in gene therapy with the approval of several gene therapy products using these delivery vectors (see Chap. 1 for a list of currently approved gene therapy products). Moreover, several other products using viral systems are in the final stages of clinical trial development [2], highlighting that new products could be approved in the next years. This broad use also emphasizes that viral vectors are now considered safe for human use, despite their natural infectious profile. Nevertheless, the safety issues were and still are major concern in the development and use of viral vectors. For that, several engineering solutions were developed trying to enhance viral vectors’ safety, without compromising their efficiency, such as (i) avoiding their replication; (ii) promoting their inactivation; and (iii) attenuating their natural toxicity. Besides their efficiency, another important advantage of viral vectors is the wide range of existing viruses, which have different features, like the type of genetic material, natural tropism, and size, which provide an enormous offer of systems for gene delivery. On the other hand, besides their safety concerns in gene therapy, viral vectors also have other disadvantages, such as their limited cloning capacity (Table 3.1).Several criteria are used to classify © Springer Nature Switzerland AG 2020 C. Nóbrega et al., A Handbook of Gene and Cell Therapy, https://doi.org/10.1007/978-3-030-41333-0_3 39 3 40 Viral Vectors for Gene Therapy Fig. 3.1 Vectors used in gene therapy clinical trials until 2017, at which time viral vectors accounted for 68% of all vectors used in these studies. Table 3.1 Main advantages and disadvantages of viral vectors used in gene therapy. Advantages High efficiency in gene transfer both in vitro and in vivo Long-term persistence (in some cases) Broad cell targets Broad range of viruses to use Natural tropism towards infection Evolved mechanisms of endosomal escape Disadvantages Potential for immune and/or inflammatory response triggering Limited cloning capacity Complex production Limited tropism to certain types of cells (in some cases) Possibility of insertional mutagenesis Molecular infection mechanisms not fully understood virus [3], including (i) the nature and form of their nucleic acid (DNA or RNA), (ii) symmetry of the capsids, (iii) presence of an envelope, (iv) the size of the virion and (v) the site of viral replication (cytoplasm or nucleus). Thus, choosing and engineering a virus for gene therapy must consider these and other features in order to ensure a successful and safe delivery of the transgene. In this section, we detail several aspects of the viral vectors most commonly used in gene therapy: lentivirus, gamma retrovirus, adenovirus, adeno-associated virus, herpes simplex virus, vaccinia, and baculovirus. 3.1 Lentiviral Vectors Lentivirus belongs to the Retroviridae family (retroviruses), which is constituted by RNAtype viruses characterized by the use of viral reverse transcriptase (RT) and the ability to insert their viral genetic information into the host genome through the action of viral integrase (IN) [4]. The name lentivirus derives from the long period between infection and the disease onset. Contrary to other retroviruses, lentivirus can also replicate in nondividing cells. They are enveloped virus, with a size ranging from 80 to 120 nm in diameter (Fig. 3.2). Lentiviruses have different host species, including primates, differing in their genome structure and entry receptors, among other features. As the majority of the lentiviral vectors used in gene therapy are based on human immunodeficiency virus 1 (HIV-1), the following descriptions are centered on this virus. HIV-1 has a single-stranded positive RNA genome with around 9 kb size, with three main open reading frames encoding for nine viral proteins (Fig. 3.3, Table 3.2) [5]. Each viral particle has two identical, positive-sense copies of the genomic RNA, each one encoding the full information needed for viral replication, although 3.1 Lentiviral Vectors 41 Fig. 3.2 Lentivirus morphology highlighting its main structural components. HIV-1 is a retrovirus with two identical copies of a single-stranded positive RNA molecule. Virions are coated by a phospholipid envelope derived from the host cell, with viral glycoproteins (gp41 and gp120) that are important for the viral entry in the cell. The viral genome is further protected by a capsid formed by p24 protein, and the virus has two additional structural proteins, p17 and p7. All these structural pro- teins are important for essential functions in the viral replicative cycle. Other components found in the virus include the reverse transcriptase, which mediates the converstion of the viral RNA genome into viral DNA; the integrase, which mediates the genomic integration of the proviral DNA into the host cell genome; and the viral protease that is responsible for processing of the gag and gag- pol polyproteins during viral maturation. recent studies suggest that both copies are required for is successful completion [6]. The viral genome is edged by long terminal repeats (LTRs), comprising each one by three regions, U3, R and U5, which are essential for transcription, reverse transcription and integration. enzymes (RT, IN, and PR). Still in the cytoplasm, the RNA molecule is reverse transcribed into a double-stranded proviral DNA molecule by the RT enzyme. This newly synthesized DNA molecule forms a complex with proteins (both viral and cellular) forming the pre-integration complex (PIC), which shuttles to the nucleus. The proviral DNA integrates into the cell genome (mediated by the IN enzyme) and starts to be transcribed into the viral mRNAs (by cellular RNA polymerase II) [8]. After some splicing events (Fig. 3.5) [9], the viral mRNAs are exported to the cell cytoplasm, where the viral proteins are produced. The viral proteins and two full-length RNA transcripts are assembled near the cellular membrane, and the viral particles (virions) are released by budding. Maturation of the viral particles occurs outside the cells. 3.1.1 Replicative Cycle The HIV-1 replicative cycle (Fig. 3.4) starts with the binding of the envelope glycoprotein gp120 to the CD4 receptor (or co-receptor CXCR4 or CCR5) of the host cell [7]. After this attachment, the viral envelope fuses with the cellular membrane, resulting in the entry of the viral core into the cell. There, the viral capsid proteins are uncoated, releasing the RNA genome and viral 3 42 Viral Vectors for Gene Therapy Fig. 3.3 Organization and main elements of a lentivirus genome, based on HIV-1. The RNA genome is about 9 kb long with three main coding segments, gag (group antigen), pol (polymerase), and env (envelope), flanked by two long terminal repeats (LTRs). Each LTR is divided into three regions, U3, R and U5, although the complete LTR sequence is only generated during reverse transcription and present in the proviral DNA. The LTRs are functional elements of the viral genome, being essential for viral transcription and therefore for the replicative cycle. The gag gene codes for structural proteins: matrix (MA), capsid (CA), nucleocapsid (NC) and transframe polypeptide (TF). The pol gene codes for the protease (PR), the reverse transcriptase (RT) and the integrase (IN). The third essential gene, env, codes for envelope proteins, the surface protein (SU) and the transmembrane protein (TM). These elements are common for all retroviruses; however, lentivirus genome is more complex and has additional elements. Tat and rev are essential regulators of the viral gene expression, coding for RNA-binding proteins that enhance transcription and act to induce late- phase gene expression, respectively. Additionally, the lentivirus genome includes four accessory regulatory elements, vif, vpr, vpu and nef, which are important for the replication and pathogenesis in vivo. 3.1.2 trials. Accounting for this use are several advantages, which are important features for gene therapy vectors, such as their mediated long-term expression or the possibility of transducing nondividing cells. Further advantages and disadvantages of their use in gene therapy applications are summarized in Table 3.3. rom Lentivirus to Lentiviral F Vectors Despite being based on HIV-1, lentiviral vectors have been used for the past years as an effective and safe system to deliver genes both in gene therapy-based preclinical studies and in clinical 3.1 Lentiviral Vectors 43 Table 3.2 Coding segments of retroviruses and their functionality. Common to all retrovirus Gene Functionality Protein gag Structural Capsid protein (CA) Matrix protein (MA) Nucleoprotein (NC) pol Replication Protease (PR) Reverse transcriptase (RT) RNase H vpr Integrase (IN) Surface glycoprotein (SU) Transmembrane protein (TM) Transactivator protein RNA splicing regulator Negative regulating factor Viral infectivity protein Virus protein r vpu Virus protein unique env Specific of complex lentivirus tat Envelope Regulatory rev nef vif Accessory Since the first use of lentiviral vectors in gene therapy, several modifications have been introduced in order to increase safety, without compromising efficiency [10]. Because lentiviruses have high rates of mutation and recombination, even the vector production could be dangerous, especially with the possibility of generating replication-competent lentiviruses (RCLs). One of the developed strategies to increase lentiviral vector safety production, trying to overcome the RCL issue, was to separate the essential lentivirus genes in different plasmids and delete some accessory genes. This strategy is the main difference between the four generations of lentiviral vectors that were developed in the last years (Fig. 3.6) [11], although many other features and alterations can be found inthe different generations (Table 3.4). Another important advance in lentiviral vectors’ development for gene therapy applications was the pseudotyping engineering, in which non-lentiviral envelope proteins are Function Formation of the capsid Formation of the inner membrane layer Formation of the nucleoprotein/RNA complex Proteolytic cleavage of gag precursor protein; release of structural proteins and viral enzymes Transcription of viral RNA into proviral DNA Degradation of viral RNA in the viral RNA/DNA replication complex Integration of proviral DNA into the host genome Attachment of virus to the target cell Anchorage of gp120, fusion of viral and cell membrane Activator of transcription of viral genes Regulation of the export of non-spliced and partially spliced viral mRNA Enhancement of infectivity of viral particles Critical for infectious virus production in vivo Component of virus particles, interaction with p6, facilitates virus infectivity Efficient virus particle release, control of CD4 degradation, intracellular trafficking modulation used in the process of producing lentiviral vectors. For example, one of the most popular alterations is the replacement of the native Env glycoprotein with the viral VSV-G protein, from the vesicular stomatitis virus envelope glycoprotein G. This modification increased viral stability, which permitted improvements in production and an increase in the viral titer yielded. Moreover, this protein has a ubiquitous receptor, which allowed the targeting of a much broader set of cells. First Generation Replication-deficient lentiviral vectors of the first generation are produced using three different plasmids: (i) the packaging construct, containing all the essential genes (except the env gene) and all the regulatory and accessory proteins, (ii) an env plasmid encoding a viral envelope glycoprotein, and (iii) a transfer vector containing the desired transgene and all the essential cis-acting 44 3 Viral Vectors for Gene Therapy Fig. 3.4 Main phases of the replicative cycle of a lentivirus, based on HIV-1 cycle. Viral particle entry into the host cell is mediated by the gp120 glycoprotein of the envelope that binds to cell receptor CD4 (1). This leads to a conformational alteration in TM (gp41) that facilitates the fusion of the virus with the cellular membrane (2). The viral core enters the cell cytoplasm, where uncoating and release of the viral RNA takes place (3). The next step is the reverse transcription of the viral RNA into proviral DNA, mediated by the RT reverse transcriptase (4). After this step, the pre-integration complex (PIC) is assembled, being composed of the RT, IN, Vpr, MA and the proviral DNA. This complex is transported through the actin microfilaments and enters into the nucleus through the nuclear pore complex (5). Inside the nucleus, the proviral DNA is integrated into the cell genome, through the action of the IN integrase (6). After integration, the proviral DNA is normally transcribed by RNA polymerase II, and the transcription of viral genes is mediated by the U3 region of the 5’LTR (7). A single viral transcript is formed, some copies will eventually be packed into newly formed viral particles, but others will serve as template for the translation of viral proteins. Therefore, in the latter case single transcripts undergo splicing to generate the different viral mRNA segments, which are then exported from the nucleus (8). In the cytoplasm, the viral mRNAs are translated into different viral proteins, which are formed from precursor peptides that undergo cleavage by viral and cellular proteases (9). The assembly of the viral particles occurs in the cellular membrane and initiates with the interaction between precursor structural proteins and the packaging signal present in the unspliced viral RNA (10). The next stages are viral budding and release, wherein viral particles emerge from the cell surface, coated by portions of the cellular membrane containing the viral glycoproteins that were produced in the endoplasmic reticulum (11, 12). Finally, outside the cells the viral particles mature through cleavage of the viral polyproteins into individual proteins and reorganization of the structural proteins into the final viral arrangement (13). elements (LTRs, Ψ, RRE). The first two plasmids do not have the packaging signal (Ψ) or any LTRs to prevent their inclusion in the produced vector particles. Second Generation Despite the important advance provided by the three-system plasmid of the first generation, some safety issues were still important as RCLs 3.1 Lentiviral Vectors 45 Fig. 3.5 Lentivirus mRNA splicing transcripts, based on the HIV-1 genome. The integrated proviral DNA is transcribed into a single mRNA transcript of approximately 9 kb size. This single transcript is then spliced into different transcripts, which can be grouped into three main clusters: (i) the primary mRNA that codes for the Gag-Pol polyprotein; (ii) intermediate mRNAs (~4 kb long) resulting from the splicing of the primary transcript, which code for polyprotein Env or accessory proteins Vif, Vpr and Vpu; and (iii) short transcripts (~2 kb long) resulting from additional splicing events and yielding mRNAs that code for the Tat, Rev and Nef proteins. Table 3.3 Main advantages and disadvantages of lentiviral vectors for gene delivery. could still be generated. To further increase safety, in the second generation of lentiviral vectors, the accessory genes (vif, vpu, vpr, nef) were deleted while maintaining the number of plasmids. This modification did not negatively affect vector titer or infectivity, but drastically reduced the chance of generating RCLs. Advantages Transduction of dividing and nondividing cells Integration into the host genome Long-term expression Possibility for no integration Cloning capacity of around 8 kb Low immunogenicity Disadvantages Need for pseudotyping Possible generation of replication-competent lentivirus (RCLs) Insertional mutagenesis Safety problems due to the presence of viral regulatory proteins Third Generation The next advance in lentiviral vectors brought the elimination of an essential gene (tat) and the introduction of the rev gene in a fourth plasmid. The need for Tat protein was overcome by replacing the U3 region in the 5’LTR by a strong heterologous promoter, such as the CMV (cyto-