Physical Pharmacy I Lec 2 PDF
Document Details
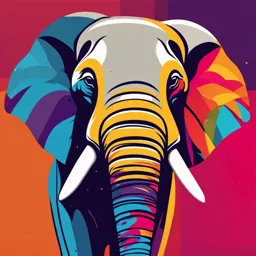
Uploaded by SuitableMinneapolis
Al-Mustaqbal University
Dr. Hayder Kadhim Drais
Tags
Summary
This document is a lecture on physical pharmacy, covering the states of matter (gases, liquids, solids), ideal gas law calculations, and molecular weight. Topics include intermolecular forces, sublimation, deposition, and liquefaction.
Full Transcript
Physical Pharmacy I Lec.2 Dr. Hayder Kadhim Drais STATES OF MATTER Gases, liquids, and crystalline solids are the three primary states of matter or phases. The molecules, atoms, and ions in the solid state are held in close proximity by intermolecula...
Physical Pharmacy I Lec.2 Dr. Hayder Kadhim Drais STATES OF MATTER Gases, liquids, and crystalline solids are the three primary states of matter or phases. The molecules, atoms, and ions in the solid state are held in close proximity by intermolecular, interatomic, or ionic forces. The atoms in the solid can oscillate only about fixed positions. As the temperature of a solid substance is raised, the atoms acquire sufficient energy to disrupt the ordered arrangement of the lattice and pass into the liquid form. Finally, when sufficient energy is supplied, the atoms or molecules pass into the gaseous state. Solids with high vapor pressures, such as iodine and camphor, can pass directly from the solid to the gaseous state without melting at room temperature. This process is known as sublimation, and the reverse process, that is, condensation to the solid state, may be referred to as deposition. Certain molecules frequently exhibit a fourth phase, more properly termed a mesophase (Greek mesos, middle), which lies between the liquid and crystalline states. This so-called liquid crystalline state. Supercritical fluids are also considered a mesophase, in this case a state of matter that exists under high pressure and temperature and has properties that are intermediate between those of liquids and gases. THE GASEOUS STATE Owing to vigorous and rapid motion and resultant collisions, gas molecules travel in random paths and collide not only with one another but also with the walls of the container in which they are confined. Hence, they exert a pressure— a force per unit area—expressed in dynes/cm2. Pressure is also recorded in atmospheres or in millimeters of mercury because of the use of the barometer in pressure measurement. Another important characteristic of a gas, its volume, is usually expressed in liters or cubic centimeters (1 cm3 = 1 mL). The temperature involved in the gas equations is given according the absolute or Kelvin scale. Zero degrees on the centigrade scale is equal to 273.15 Kelvin (K). 1 The Ideal Gas Law The gas laws formulated by Boyle, Charles, and Gay-Lussac refer to an ideal situation where no intermolecular interactions exist and collisions are perfectly elastic, and thus no energy is exchanged upon collision. Ideality allows for certain assumptions to be made to derive these laws. Boyle’s law relates the volume and pressure of a given mass of gas at constant temperature, The law of Gay-Lussac and Charles states that the volume and absolute temperature of a given mass of gas at constant pressure are directly proportional, These equations can be combined to obtain the familiar relationship In equation (2–4), P1, V1, and T1 are the values under one set of conditions and P2, V2, and T2 the values under another set. From equation (2–4) it is seen that PV/T under one set of conditions is equal to PV/T under another set, and so on. Thus, one reasons that although P, V, and T change, the ratio PV/T is constant and can be expressed mathematically as in which R is the constant value for the PV/T ratio of an ideal gas. This equation is correct only for 1 mole (i.e., 1 g molecular weight) of gas; for n moles it becomes 2 Molecular weight The approximate molecular weight of a gas can be determined by use of the ideal gas law. The number of moles of gas n is replaced by its equivalent g/M, in which g is the number of grams of gas and M is the molecular weight: The molar gas constant R: 3 Example 1: Calculation of Volume Using the Ideal Gas Law Q: What is the volume of 2 moles of an ideal gas at 25◦C and 780 mm Hg, R= 0.082205 L atm/mole deg? Answer: PV = nRT 1 atomsphere = 760 mmHg Example 2: How many moles are there in 985 mL of nitrogen at 0.0° C and 1.00 x 10-6 mm Hg, R = 0.0821 L·atm/mol·K? Answer: P = 1.00 x 10-6 mm Hg T = 0.0° C + 273 = 273 K V = 985 mL R = 0.0821 L·atm/mol·K PV = nRT n = PV/RT n = 1.00 x 10-6 mm Hg/760 mm Hg x 985 mL x 1 L/103 mL/ (0.0821 L·atm/mol·K x 273 K) = 5.78 x 10-11 moles THE LIQUID STATE Liquefaction of Gases When a gas is cooled, it loses some of its kinetic energy in the form of heat, and the velocity of the molecules decreases. If pressure is applied to the gas, the molecules are brought within the sphere of the van derWaals interaction forces and pass into the liquid state. Because of these forces, liquids are considerably denser than gases and occupy a definite volume. 4 The transitions from a gas to a liquid and from a liquid to a solid depend not only on the temperature but also on the pressure to which the substance is subjected. If the temperature is elevated sufficiently, a value is reached above which it is impossible to liquefy a gas irrespective of the pressure applied. This temperature, above which a liquid can no longer exist, is known as the critical temperature. The pressure required to liquefy a gas at its critical temperature is the critical pressure, which is also the highest vapor pressure that the liquid can have. The further a gas is cooled below its critical temperature, the less pressure is required to liquefy it. Based on this principle, all known gases have been liquefied. The critical temperature of water is 374◦C, or 647 K, and its critical pressure is 218 atm, whereas the corresponding values for helium are 5.2 K and 2.26 atm. The critical temperature serves as a rough measure of the attractive forces between molecules because at temperatures above the critical value, the molecules possess sufficient kinetic energy so that no amount of pressure can bring them within the range of attractive forces that cause the atoms or molecules to “stick” together. The high critical values for water result from the strong dipolar forces between the molecules and particularly the hydrogen bonding that exists. Aerosols Gases can be liquefied under high pressures in a closed chamber as long as the chamber is maintained below the critical temperature. When the pressure is reduced, the molecules expand and the liquid reverts to a gas. This reversible change of state is the basic principle involved in the preparation of pharmaceutical aerosols. In such products, a drug is dissolved or suspended in a propellant, a material that is liquid under the pressure conditions existing inside the container but that forms a gas under normal atmospheric conditions. Chlorofluorocarbons and hydrofluorocarbons have traditionally been utilized as propellants in these products because of their physicochemical properties. Vapor Pressure of Liquids When a liquid is placed in an evacuated container at a constant temperature, the molecules with the highest energies break away from the surface of the liquid and pass into the gaseous state, and some of the molecules subsequently return to the liquid state, or condense. When the rate of condensation equals the rate of vaporization at a definite temperature, the vapor becomes saturated and a dynamic equilibrium is established. The pressure of the saturated vapor∗ above the liquid is then known as the equilibrium vapor pressure. 5 The presence of a gas, such as air, above the liquid decreases the rate of evaporation, but it does not affect the equilibrium pressure of the vapor. As the temperature of the liquid is elevated, more molecules approach the velocity necessary for escape and pass into the gaseous state. As a result, the vapor pressure increases with rising temperature, as shown in Figure 2–5. Heat of Vaporization The relationship between the vapor pressure and the absolute temperature of a liquid is expressed by the Clausius–Clapeyron equation: Where: p1 and p2 are the vapor pressures at absolute temperatures T1 and T2, and ∆Hv is the molar heat of vaporization, 6 Example: Boiling Point If a liquid is placed in an open container and heated until the vapor pressure equals the atmospheric pressure, the vapor will form bubbles that rise rapidly through the liquid and escape into the gaseous state. The temperature at which the vapor pressure of the liquid equals the external or atmospheric pressure is known as the boiling point. All the absorbed heat is used to change the liquid to vapor, and the temperature does not rise until the liquid is completely vaporized. The atmospheric pressure at sea level is approximately 760 mm Hg; at higher elevations, the atmospheric pressure decreases and the boiling point is lowered. At a pressure of 700 mm Hg, water boils at 97.7◦C; at 17.5 mm Hg, it boils at 20◦C.The change in boiling point with pressure can be computed by using the Clausius–Clapeyron equation. The heat that is absorbed when water vaporizes at the normal boiling point (i.e., the heat of vaporization at 100◦C) is 539 cal/g or about 9720 cal/mole. For benzene, the heat of vaporization is 91.4 cal/g at the normal boiling point of 80.2◦C. These quantities of heat, known as latent heats of vaporization, are taken up when the liquids vaporize and are liberated when the vapors condense to liquids. 7 The boiling points of normal hydrocarbons, simple alcohols, and carboxylic acids increase with molecular weight because the attractive van der Waals forces become greater with increasing numbers of atoms. Branching of the chain produces a less compact molecule with reduced intermolecular attraction, and a decrease in the boiling point results. In general, however, the alcohols boil at a much higher temperature than saturated hydrocarbons of the same molecular weight because of association of the alcohol molecules through hydrogen bonding. The boiling points of carboxylic acids are more abnormal still because the acids form dimers through hydrogen bonding that can persist even in the vapor state. Nonpolar substances, the molecules of which are held together predominantly by the London force, have low boiling points and low heats of vaporization. Polar molecules, particularly those such as ethyl alcohol and water, which are associated through hydrogen bonds, exhibit high boiling points and high heats of vaporization. 8