Physiology of Microorganisms PDF
Document Details
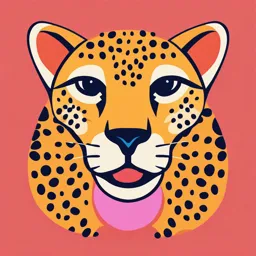
Uploaded by EffortlessNarcissus4194
Beni-Suef National University
2024
Prof. Dr. Ahmed Shawky
Tags
Related
- Bacteriology/MLSBACTC Past Paper PDF 2024-2025
- Week 2: Bacterial Physiology, Metabolism, and Infection (PDF)
- Microbial Physiology (2002) by Moat, Foster, Spector - PDF
- The Basic Structures of Bacteria Anatomy and Physiology PDF
- Microbial Biotechnology PPT 44 WDO2 (1) PDF
- MICR20010 Lecture 5 2024 - Microbiology Lecture Notes PDF
Summary
This document provides an overview of the physiology of microorganisms, focusing on the structure and function of prokaryotic cells. It covers topics like flagella, fimbriae, pili, slime layers, and capsules. The document is part of a Biochemistry-Microbiology program.
Full Transcript
Beni Suef National University Faculty of Science Biochemistry-Microbiology Program Physiology of Microorganisms By Prof. Dr. Ahmed Shawky For Third level st...
Beni Suef National University Faculty of Science Biochemistry-Microbiology Program Physiology of Microorganisms By Prof. Dr. Ahmed Shawky For Third level students Biochemistry-Microbiology Program 2024/2025 Physiology of microorganisms (Microbial Physiology) 1 Cell Structure and Function I-Structure and Function of Procaryotic Cell Bacterial cell structure: The bacterial cell consists of the surface layer and protoplast. The surface layer includes flagella, cilia, capsule and cell wall while the protoplast consists of the cytoplasmic membrane, cytoplasm, nuclear material, stored materials, ribosomes and vacuoles. A: Prokaryotic Cell Surfaces 1. Flagella It is a long filament structure made up of a protein of a special type called flagelin. The flagella originate from a basal body embedded in the cell wall and cytoplasmic membrane. Function: needed for motility and swarming. 2. Fimbriae and pili: Fimbriae and pili are hair-like appendages present on the bacterial cell wall. Some bacterial cells have fimbriae that are shorter than flagella and consist of the protein fimbrilin. It helps the cell to adhere to surfaces, especially in pathological conditions. Pili appendages are found in abundance in the negative bacteria and consist of the protein pilin. Pili are fine filaments longer than fimbriae and only one or two per cell These appendages perform a number of functions in the bacterial cell, Forms the place where bacteriophage is fixed, It also fixes the bacteria themselves in hemagglutination reactions. It involved in the process of bacterial conjugation 2 3 3-Slime layer or capsule The bacterial cell is surrounded by a sticky gelatinous layer called a capsule. The capsule material is mostly composed of complex polysaccharide carbohydrates and in some species amino acids are included in its composition. Importance of the capsule The capsule protects bacteria from adverse environmental conditions, especially drought, and provides resistance against cellular phagocytosis. In addition to helping the cell adhere to surfaces, it is useful in distinguishing between species. 4. Cell Wall: Eubacteria have a cell wall that is a rigid membrane that represents about 20% of the dry weight of the cell. The cell wall consists of several layers of polysaccharides, lipids, and some amino acids that together form what are called mucopeptides or what is called peptidoglycan. The carbohydrates that enter into its composition are two compounds: the amino sugar N- acetyl glucose amine and the other is N-acetyl muramic acid linked together by beta 1-4 glycosidic bonds. Importance of the cell wall: Cell wall gives cells their external shape and protects them from osmotic pressure. The cell wall forms the substrate on which bacteriophages can attach. B: Protoplast 1- Cytoplasmic membrane The plasma membrane or bacterial cytoplasmic membrane is composed of a phospholipid bilayer. 4 5 The phospholipids of bacteria contain ester-linked phospholipids whereas the archaea contain fatty alcohols linked to glycerol via either diether or tetraether bonds. Unlike eukaryotes, bacterial membranes (with some exceptions e.g. Mycoplasma and methanotrophs) generally do not contain sterols. Function: -acting as a permeability barrier for most molecules -serving as the location for the transport of molecules into the cell -serving as the location for energy conservation 2- Cytoplasm: The cytoplasm consists of all of the contents of the cell inside the plasma membrane, which mainly contains 70S ribosomes and chromatophores in green bacteria, in addition to various inclusions. 3-Ribosomes Ribosomes may be filled the cytoplasmic or loosely attached to the plasma membrane. Ribosomes are very complex structures made of both protein and ribonucleic acid (RNA). Procaryotic ribosomes are smaller than the cytoplasmic or endoplasmic reticulum- associated ribosomes of eucaryotic cells. Function: They are the site of protein synthesis. 4- Inclusion Bodies Inclusion bodies, granules of organic or inorganic substances, are present in the cytoplasmic matrix. Some inclusion bodies may be: -free in the cytoplasme e.g. polyphosphate granules, cyanophycin granules, and some glycogen granules or -enclosed by a shell e.g. poly-β-hydroxybutyrate granules, some glycogen and sulfur granules, and gas vacuoles. 6 Many inclusion bodies are used for storage and also reduce osmotic pressure. Stored organic materials: a- Organic inclusion bodies usually contain either glycogen or poly-β-hydroxybutyrate (PHB) granules. Glycogen and PHB inclusion bodies are carbon storage reservoirs providing material for energy and biosynthesis. Many bacteria also store carbon as lipid droplets. b- Cyanobacteria have two distinctive organic inclusion bodies: Cyanophycin granules are composed of large polypeptides. The granules store extra nitrogen for the bacteria. Carboxysomes are polyhedral protein and contain ribulose-1, 5-bisphosphate carboxylase, called Rubisco. Rubisco is the critical enzyme for CO 2 fixation. Carboxysomes also may be a site of CO2 fixation. c- Gas vacuoles are present in many photosynthetic bacteria and a few other aquatic procaryotes. Gas vacuoles are aggregates of small, hollow, cylindrical structures and their walls are composed of a single small protein. Gas vacuole, provides buoyancy to some aquatic procaryotes. Stored inorganic materials: Two major types of inorganic inclusion bodies are seen in procaryotes: a- Polyphosphate granules Many bacteria store phosphate as polyphosphate granules or volutin granules. Thus volutin granules function as storage reservoirs for phosphate, an important component of cell constituents (e.g. nucleic acids). b- Sulfur granules Photosynthetic bacteria can use hydrogen sulfide and store the resulting sulfur in either the periplasmic space or in special cytoplasmic globules. 7 5 - Nucleoid: The cell's DNA content is occurred in the nuclear region as coiled fine filaments that contains all or most of the genetic material. The nuclear material is not surrounded by basic proteins, is not defined by a nuclear membrane, and does not contain nuclei. Function: carrying genetic material (DNA) 6 - Plasmids: Plasmids are found in gram-positive or gram-negative bacteria. They are double-stranded circular DNA molecules outside the chromosomes, and can replicate independently of the bacterial chromosomes. Different types of plasmids may exist in a single cell. i-Transmissible plasmids: These plasmids can be transferred from one cell to another. They are large and contain about 12 genes responsible for the formation of sexual cilia and the enzymes necessary for transport. They are found in the cell in small numbers (1-3 units). ii- Non-transmissible plasmids They are small and do not contain transfer genes, They are often found in large numbers 1-60 units in the cell. Plasmids carry genes that code for the following functions and structures: resistance to antibiotic by many enzymes. resistance to heavy metals with the help of the enzyme reductase resistance to ultraviolet light with the presence of modification enzymes (DNA repair including many enterotoxins (Exogenous Toxins). 8 II-Structure and Function of Eucaryotic Cells A-Eukaryotic Cell Surfaces 1-Cilia and flagella They are the most prominent organelles associated with motility. They move the microorganism along. They differ from one another in two ways: First, cilia are typically only 5 to 20 µm in length, whereas flagella are 100 to 200 µm long. Second, their patterns of movement are usually distinctive. 2- Cell wall Both eukaryotic and prokaryotic microorganisms are often enclosed within a rigid cell wall. Many algae, as well as oomycetes, contain cellulose (cellulose, a β-1,4-linked polymer) as a major cell wall constituent. The cell walls of many fungi contain chitin, a linear polymer of N-acetyl glucosamine. Most fungi, algae, and higher plants contain microfibrils of either cellulose or chitin as a prominent skeletal component of their cell walls. Production of these microfibrils occurs at or near the surface of the cell. Chemical analysis of yeast walls reveals the presence of 29% β-glycans (both 1,6-β- glycan and 1,3-β-glycan are found), 31% mannan, and 13% protein. Yeast cell walls also contain small percentages of lipids and other materials. B: Protoplast 1- Cytoplasmic membrane (Plasma Membrane) The cell membrane consists of a lipid bilayer, made up of two layers of phospholipids with cholesterols. The membrane also contains membrane proteins, including integral proteins that serve as membrane transporters, and peripheral proteins that acting as 9 enzymes to facilitate interaction with the cell's environment. Glycolipids embedded in the outer lipid layer serve a similar purpose (serve as recognition sites for cell–cell interactions). Selectively permeable barrier with transport systems, mediates cell-cell interactions. 2-The cytoplasmic matrix The many organelles of eucaryotic cells lie in the cytoplasmic matrix. The matrix is one of the most important and complex parts of the cell. It is the “environment” of the organelles and the location of many important biochemical processes. 3-The Endoplasmic Reticulum The endoplasmic reticulum (ER) is an irregular network of branching and membranous tubules. The nature of the ER varies with the functional and physiological status of the cell. In cells synthesizing a great deal of protein for purposes such as secretion, a large part of the ER is scattered on its outer surface with ribosomes and is called rough endoplasmic reticulum (RER). Other cells, such as those producing large quantities of lipids, have ER that lacks ribosomes. This is smooth ER (SER). The endoplasmic reticulum has many important functions. it transport proteins, lipids, and other materials it is also involved in the synthesis of many of the materials it transports. 4-The Golgi apparatus The Golgi apparatus is composed of flattened, saclike cisternae stacked on each other. These membranes, like the smooth ER, lack bound ribosomes. There are usually around 4 to 8 cisternae in a stack. A complex network of tubules and vesicles is located at the edges of the cisternae. These stacks of cisternae, often called dictyosomes, can be clustered in one region or scattered about the cell. 10 The Golgi apparatus packages materials and prepares them for secretion. Often participates in the development of cell membranes. 5-Lysosomes They are found in mosteucaryotic organisms, including protists, fungi, plants, and animals. Lysosomes are roughly spherical and enclosed in a single membrane. They are involved in intracellular digestion and contain the enzymes needed to digest all types of macromolecules. 6- Eucaryotic ribosomes The eucaryotic ribosome is larger than the procaryotic ribosome. Eucaryotic ribosomes can be either associated with the endoplasmic reticulum or free in the cytoplasmic matrix. Both free and ER-bound ribosomes synthesize proteins. 7-Mitochondria Mitochondria are subcellular organelles that carry out oxidative metabolism (respiration) in eukaryotic cells. Tricarboxylic acid cycle activity and the generation of ATP by electron transport and oxidative phosphorylation take place here. Mitochondria usually are cylindrical structures and bounded by two membranes, an outer membrane separated from an inner membrane by intermembrane space. The inner membrane has special enfoldings called cristae (s., crista), which greatly increase its surface area. Enzymes and electron carriers involved in electron transport and oxidative phosphorylation that yield energy in the form of ATP are located only in the inner membrane. The enzymes of the tricarboxylic acid cycle and catabolism of fatty acids are located in the matrix. 11 8- Nucleus The nucleus is the most prominent organelle in eukaryotic cells. The nucleus is the storehouse for the cell’s genetic information and is its control center. Nuclear Structure Nuclei are membrane-delimited spherical bodies. Chromatin (DNA-containing part of the nucleus) can be seen within the nucleoplasm of the nucleus. In nondividing cells, chromatin is dispersed, but it condenses during cell division to become visible as chromosomes. The nucleus is bounded by the nuclear envelope, a complex structure consisting of inner and outer membranes. Many nuclear pores penetrate the envelope and serve as a transport track between the nucleus and surrounding cytoplasm. 12 13 Microbial Nutrition Microbes, like other living organisms, need nutrients that help them carry out their biosynthesis reactions, generate energy, and build their living matter. First: The common Nutrient Requirements Water, which constitutes about 70-80% of the cell weight, is a general and basic need for all microbes in addition to their need for the elements that make up their cells, which are: carbon, oxygen, hydrogen, nitrogen, sulfur, phosphorus, potassium, calcium, magnesium, and iron, which are called macroelements or macronutrients. They are essential and required in relatively large quantities by microorganisms. The first six elements (P-S-N-H-O-C) form sugar compounds (carbohydrates), fats, proteins and nucleic acids. While the remaining elements play many important roles in all cell activities. In addition, all microorganisms need very small amounts of other nutrients, called trace elements or microelement elements, such as zinc, cobalt, molybdenum, nickel, copper and iodine. These elements are often part of the enzymes that mediate thousands of chemical reactions on which metabolism in microorganisms depends. Some microorganisms also need very small amounts of substances that they cannot self- synthesize and are usually called growth factors, including vitamins, amino acids and some components of nucleic acids. These factors are also involved as cofactors in the action of enzymes. Second: Nutritional Types of Microorganisms All microorganisms need carbon, hydrogen, oxygen, energy and electrons to perform synthesis and construction processes during their growth. The ability of these organisms to perform synthesis processes varies, as do the sources of carbon, energy and electrons they use when carrying out their biological processes of different types. Classification of microorganisms according to the source of energy, carbon and electrons:- 14 A. According to the source of carbon: Microorganisms are classified according to the source of carb on into: 1. Autotrophs, which are microorganisms that use carbon dioxide gas as a source of carbon. 2. Heterotrophs, which are microorganisms that use organic compounds as a source of carbon. B. According to the energy source: Microorganisms are classified according to the energy source into: 1. Phototrophs, which are microscopic organisms in which light is a source of energy 2. Chemotrophs, which are organisms that oxidize organic or mineral compounds to obtain the energy needed for various vital processes. C- According to the source of electrons: Microorganisms are classified according to the source of electrons into: 1. Lithotrophs The source of electrons is inorganic molecules 2. Organotrophs The source of electrons is organic molecules Third: Uptake of nutrients by the cell Growth as a dynamic process requires a quantity of energy and food in order to build various compounds. Since food is present in the external environment, the first step in the growth of microorganisms is the absorption of these nutrients and their transport into the cell. Chemotaxis has been observed towards amino acids and sugars in some motile bacteria due to the presence of protein or sugar chemical carriers in these bacteria. The amino acid Serine attracts E. coli bacteria and Leucine repels them. It is necessary to distinguish between how complex compounds with high molecular weight are used and the permeability of compounds with low molecular weight. n the first stage, complex compounds such as cellulose, proteins and fats are digested by external enzymes secreted by microorganism cells into the external environment through 15 the cell wall. Most external enzymes begin to break down complex compounds, converting them into small, simple units, such as the enzyme Amylases, which decomposes starch, enabling it to pass into the cell. The entry of low molecular weight compounds is done in one of the following ways: A. Simple diffusion Dissolved nutrients such as glycerol enter and exit through the semi-permeable cytoplasmic membrane according to the laws of diffusion (the speed of entry and exit of materials depends on the size of the molecules and their charge and the difference in concentration of these materials inside and outside the cell) and without using any cellular energy. B. Facilitated diffusion Where it is noted that when the concentration of materials outside the cell increases, the speed of entry and exit of these materials increases through the semi-permeable cytoplasmic membrane (selective) using protein carriers that are sometimes called transport enzymes Permeases and are located within the plasma membrane. C. Active transport Where nutrients enter by a group of active transport enzymes located in the cytoplasmic membrane, which are characterized first by their specialization in transport (Specificity). As the specialization of the enzyme group B-galactosides permease of E. Coli bacteria to transport sugars, and other groups in the same bacteria specialize in transporting amino acids and then by their association with membrane proteins, as The previous specialization was noted to be related to the type of protein compounds present on the cytoplasmic membrane. It is also characterized by its use of cellular energy. 16 Facilitated Diffusion Active Transport ABC Transporter Function 17 Fourth: Culture Media These are the media in which microorganisms can find all their nutritional needs, and we often distinguish between two types of culture: The first is by culturing microbes in living media and distinguishes only parasitic microbes. (In-Vivo) As for the second, it is by culturing them in artificial laboratory media (In-Vitro). In this second type, the medium can be liquid and contain some necessary mineral elements, and it can also be solid by adding some special materials such as agar or gelatin. The difference in nutritional requirements for microorganisms necessitates the existence of several types of media that differ according to the type to be cultured. These types are: A- Enriched media These media consist of nutrient broth or nutrient agar to which a little blood or plant extract has been added. These media are used for heterotrophic microorganisms. B- Selective media Adding some special chemicals to the nutrient agar medium helps the growth of some microorganisms, while it does not affect others. When a certain concentration of crystal violet is added, it inhibits the growth of some gram-positive bacteria, while it does not affect the growth of some gram-negative bacteria. The same is true for some carbon compounds, where maltose is used for some bacteria, for example, while others prefer sucrose. C- Differential media: Some specific media can be used to differentiate between different species. If we add a medium of agar and blood to a bacterial colony, for example, a transparent area may appear around the growing bacterial colonies as a result of the bacteria analyzing the red blood cells. This transparent area may not appear around the colonies, which indicates that these bacteria cannot analyze the red blood cells. Thus, it is concluded that this medium has been able to differentiate between two types of bacteria, the first of which analyzes the added medium and the second of which is unable to analyze it. 18 D- Assay media Some media of specific composition and experimentally selected can be used to measure the amount of vitamins, amino acids or antibiotics resulting from the growth of some microorganisms. E- Media for Enumeration of Bacteria These are special and specific media used to estimate the numbers of a certain type of bacteria, as is the case with milk or water bacteria. These media are often of specific composition. F- Media for Characterization of Bacteria These are also specific media, used to isolate bacterial species that have a certain functional ability, as is the case with nitrogen-fixing bacteria or nitrifying bacteria...etc. G- Maintenance Media The purpose may be to maintain continued growth. Therefore, some special materials are added to preserve the colony and keep it alive for as long as possible. There is a gradation in media between solid, semi-solid, and liquid that varies according to the type of bacterial culture. TYPES OF MICROBIAL CULTURE Microbial culture processes can be carried out in different ways. There are three models of fermentation used in industrial applications: batch, continuous and fed batch fermentation. 1-Batch Fermentation A batch fermentation system is a closed system. At time t=0, the sterilized nutrient solution in the fermenter is inoculated with microorganisms and incubation is allowed to proceed at a suitable temperature and gaseous environment for a suitable period of time. In the course of the entire fermentation nothing is added, except oxygen (in case of aerobic microorganisms), an anti-foam agent, acid or base to control pH. The 19 composition of the medium, the biomass concentration and the metabolite concentration generally change constantly as a result of metabolism of the cells. 2- Continuous Fermentation In continuous fermentation an open system is set up. Sterile nutrient solution is added to the bioreactor continuously and an equivalent amount of converted nutrient solution with microorganisms is simultaneously taken out of the system. 3- Synchronous Growth Synchronous growth of a bacterial population is that during which all bacterial cells of the population are physiologically identical and in the same stage of cell division cycle at a given time. Synchronous culture is that in which the growth is synchronous, i.e., all thebacterial cells of the population are physiologically identical and in the same stage of cell division cycle at a given time. A synchronous culture can be obtained either by manipulating environmental conditions such as by repeatedly changing the temperature or by adding fresh nutrients to cultures as soon as they enter the stationary phase, or by physical separation of cells by centrifugation or filtration. 20 Microbial Growth The word growth is used in prokaryotes in general and in some eukaryotic microbes to express the increase in the number of microbial cells over the original number with which the culture began its growth. That is, growth in bacteria expresses reproduction and comes as a natural result. Their number increases without an increase in the size of their individuals. THE GROWTH CURVE The growth of microorganisms is represented graphically by drawing a curve that shows the relationship between the incubation time (t) the logarithm of the number of viable cells (log n). This curve is characterized by the presence of four phases, which are: 1-Lag phase Adding microbes to a new medium does not mean doubling their number immediately, due to the new conditions that are different from the previous ones. This also does not mean that they will enter a dormant phase. Studies have shown that a single cell in this phase increases in size, is physiologically active, and synthesizes new protoplasm. The microbial cell in these new conditions may suffer from a deficiency in its enzymes, and it needs time to adapt to this medium. Therefore, we say that this cell is dormant only in terms of its division, meaning that no growth exists. The duration of this phase varies according to: Type of microbe Age of the cultured microbe: If the microbes are young, this phase will be shorter. Nature of the new medium: The duration of this phase is limited if the medium is suitable and does not contain growth inhibitors or antibiotics. Quantity of cultured microbes: The larger the quantity, the shorter the duration of this phase as well. 2-Logarithmic phase (Exponential Phase) Microbial growth accelerates in this phase, and the growth rate is constant. The growth curve takes the form of a straight line (when the logarithm of the number of cells is 21 placed against time). The increase in the number of cells reaches its maximum due to the short time of division. In addition, microbial cells are similar in their chemical composition, metabolic activity, and the rest of their physiological characteristics. The time period of this phase is related to the beginning of the phase that follows it. 3. Stationary phase The logarithmic phase of growth ends after several hours, and the rate of reproductionbegins to decrease until it balances with the rate of cell death, in a way that the number of living cells remains constant, and thus the stationary phase begin. The occurrence of this phase is attributed to several reasons, perhaps the most important of which is the lack of nutrients and the accumulation of toxic growth products resulting from cellular activity, which leads to a change in the composition of the medium. The length of this phase depends primarily on the microbial type and its sensitivity to the surrounding conditions. The greater sensitivity leads to the shorter phase period. 4. Decline phase The next phase is the stationary phase, also called the death phase, because the rate of death of microbial cells is high, due to the depletion of nutrients and the accumulation of growth-inhibiting substances. The duration of this phase varies depending on the microbial type. While we find that the cells of some Gram-negative cocci die within 72 hours or less, we find that some cells in other types remain live for a period ranging from months to several years. 22 Microbial growth measurement Since microbial growth is the increase in the number of cells formed as a result of division, the growth can be estimated in several ways based on the following foundations: 1. Measurement of the total number of microbial cells directly using a microscope, or indirectly by colony counting. 2. Measurement cell mass directly by estimating the increase in dry or wet weight, or through one of the elements of the cellular contents such as carbon or nitrogen or through indirect method depending on the degree of medium turbidity. 3. Measurement of cellular activity, which is an indirect method based on comparing enzymatic activity, the output is the amount of growth to be measured. You can follow one of the following methods: A- Estimation of the total number of cells: This count is done directly under the microscope in colored preparations. A known volume of the microbial culture or suspension (0.01 ml) is spread evenly over a known area of a glass slide, where the resulting membrane is fixed and colored, then the individual microbes are counted by examining it under the microscope field, and from there the number of cells in (1 ml) of the suspension is calculated. Currently, special plates such as Petroff hausser are used, which have a counting chamber that helps to carry out the count accurately and quickly without coloring. The disadvantages of this method are that it gives the total number of living and dead cells, and that it is not sufficient in the case of very dilute suspensions. B- Counting colonies in plates: This method depends on cultivating microbes in suitable solid food media inside sterile Petri dishes, where (ml) of the culture to be examined is taken and inoculated into the solid culture medium, then these dishes are incubated at a suitable temperature, and the colonies growing in them are counted after a certain period of time that varies according to the type of microbe studied, for example (24 hours) for E. coli bacteria and at least three days for Azotobacter bacteria. 23 In this way, we can obtain a large number of colonies whose borders overlap, and the possibility of error becomes large, so the studied sample is gradually diluted to a degree that allows the appearance of a number of colonies in the dishes ranging between (30 - 300) colonies, and by knowing the dilution, we can know the number of microbes present in (1 ml). This method gives good results when cultivating bacteria in milk, water, food, etc., because it is an easy and accurate method. C- Turbidimetric method Microbes grow in liquid media, and this growth is accompanied by an increase in the turbidity of the medium due to the increase in the number of cells in it. If we pass a light beam through the medium, the bacterial cells absorb and scatter some of these light rays. In fact, the amount of light absorbed and scattered is proportional to the mass of cells in the medium, as the increase in the number of cells leads to an increase in the scattering and absorption of light. To measure the extent of this scattering, either a spectrophotometer or a turbidimeter is used. This method is widely used in studying bacterial growth due to its speed and accuracy. However, it cannot be used in media that are heavily colored or contain suspended materials other than bacterial cells, or when the growth of the culture is weak. It should be noted that this method takes into account both dead and living cells. D. Estimating the increase in dry weight of the cell: This method is used in the case of microbial cultures with dense growth, and here the cells must be washed well from impurities and suspended materials, and it is also considered one of the insensitive methods. Continuous culture: The extension of the logarithmic phase in bacterial cultures is an important topic for both scientific research and industrial progress, and this can be achieved in the laboratory, by making the culture medium constant, either by adding a constant stream of the medium used continuously so that the concentration of ions and nutrients remains the same using a device called a Chemostat, or by adding fresh culture medium when the turbidity of the culture reaches a certain limit in a device called a Turbidostat. This growth is called balanced growth or constant growth. 24 Factors Affecting Microbial Growth Various environmental factors affect the growth of microbes, whether these microbes are found in their natural or artificial environments. The following are the most important of these factors: 1. Nutrients: The need for nutrients by microorganisms varies depending on the microbial species.In all cases, nutrients must be available that are used in the production processes of the cell and in the respiration processes. Therefore, growth increases significantly when the appropriate nutrients are present in sufficient quantities, while a deficiency in any of them leads to stopping growth. 2. Moisture and dryness: Microorganisms need moisture and free water to transport nutrients in dissolved form into the cell, wastes out of it and to maintain the water content of the protoplasm. Therefore some microorganisms especially those living in marine or freshwater, as well as pathogenic species, die quickly if exposed to dehydration. It is generally observed that microorganisms can tolerate drought either by stopping growth or by forming spores. A large number of them can remain alive for a long time without growing or multiplying if they are exposed to drying under low pressure (high vacuum) where vital activities decrease and food exchanges stop completely. 3. Temperature Microorganisms do not have special devices to regulate their internal temperature, and their temperature is the temperature of the environment in which they live. Therefore, this environment temperature controls the biochemical reactions carried out by microbes, affecting their speed and thus determining the speed and amount of the overall growth of these microbes. All microbes can live in a certain temperature range, and when they exceed it, they lose the ability to continue. Therefore, each type of microbe has a minimum temperature, a maximum temperature and optimum temperature lie between the two previous temperatures and are known as the optimum growth temperature, where growth reaches its maximum. It is worth noting that this temperature may not be optimal 25 for the rest of the cell's other activities. Temperature changes affect metabolic activities, and even the shape of the microbial cell. Microbes can also be divided into three groups according to their temperature preference: A-Psychrophiles These microbes are characterized by their ability to grow at a minimum temperature of zero degrees Celsius or close to it, and a maximum of about 30 m, but the ideal ͦ. temperature for their growth is between 15 - 20 C ͦ , while However, there are species that have adapted to live at a temperature close to -7 C ͦ. Some types of soil and marine bacteria belong to their ideal growth is between 20 - 30 C this group, as well as some species that cause rotting of fish and aquatic plants, which cause spoilage of food preserved by refrigeration. B - Mesophiles These are microbes whose best growth occurs between 25-40°C. Their minimum temperature is between 10-15°C and their maximum temperature reaches 45°C. This group includes a large number of Saprophiles, and all pathogenic microbes that are parasitic on humans and warm-blooded animals and grow well at 37°C. C - Thermophiles The best growth of these microbes is achieved between 45-60 °C, noting that some growth rates of these microbes qithin the limits of moderate thermophilic microbes. The minimum temperature is between 25 and 45 °C, while its maximum temperature reaches 75 °C and sometimes 90 °C. Microbes of this group are spread in hot springs and animal dung, contributing to raising the temperature of natural fertilizers when fermented as some of them occur in some preserved food. 4. Pressure Pressure is one of the important factors that affect the life and growth of microorganisms and we must distinguish between two types of pressure: 26 a- External or mechanical pressure: Some types of microbes can withstand very high pressure exceeding 1200 kg2/cm, as is the case with some bacteria that live in the depths of the seas and oceans and those that live in the petroleum layers, where they are exposed to high pressures that suit their lifestyle, so they grow and reproduce, while they cannot grow and reproduce at normal pressures and are called pressure-loving microbes Parophiles. Other than that, most non- Spore-forming bacteria die when pressure ranging from 300 to 6000 atmospheres is applied to them within 45 minutes, while Spore-forming bacteria need more than 20,000 pressures. b-Solution pressure: The concentration of nutrient solutions greatly affects the growth of microorganisms, and solutions are generally divided into three categories: Hypotonic Solutions: with weak concentration or with low solution pressure. Hypertonic Solutions: with high concentration or with high solution pressure. Isotonic Solutions: with equal concentration or with neutral solution pressure. If the microbial cell is placed in a liquid with a high solubility pressure, water comes out of it and it shrinks (plasmolysis). However, if it is placed in a liquid with a low solubility pressure, water enters it and it swells and may rupture. Studies indicate that most microbes are not affected by a change in the concentration of the solution within the limits of (0.5 - 3) because the solubility pressure inside the cell is approximately equal to (5 -25) atmospheric pressure, while high solubility pressure (90 - 100 atmospheric pressure) causes a harmful effect on them, with the exception of halophilic microbes that live in environments containing a high concentration of salt (15- 25%). 5-Oxygen concentration Microorganisms need oxygen for the oxidation and reduction processes that occur 27 during respiration, and oxygen and carbon dioxide are among the most important gases that affect the life and growth of microbes. Therefore, they are divided according to their need for oxygen into five sections: A- Obligate aerobe They are bacteria that grow in the presence of large amounts of oxygen, such as the genus Azotobacter and Nitrobacter because it contains only oxidation enzymes (cytochromes - peroxidases). B - Strict anaerobe They grow in the absence of oxygen, such as the genera Bacteriodes and Clostridium, because they contain only dehydrogenation enzymes. They obtain the energy needed for their vital actions through the fermentation process, where they remove hydrogen through a series of oxidation-reduction reactions between organic compounds, some of which act as hydrogen donors (so they are oxidized) and others as hydrogen acceptors (so they are reduced). Thus, these bacteria seek to make the internal oxidation and reduction energy suitable for the conditions of their structural processes and growth. C - Facultative anaerobe Some types of bacteria are able to grow in the presence or absence of oxygen, as in the genus Escherichia. They obtain the energy needed for their vital activities through the fermentation process when oxygen is lost from the medium, and they carry out the process of respiration if it is present. D - Aerotolerant anaerobe Aerotolerant anaerobe D - Aerotolerant anaerobe Uses fermentative activities to produce ATP and obtain energy. It is an anaerobic bacteria, but it can protect itself from the toxic effects of oxygen because it possesses the enzyme superoxide dismutase and Peroxidase. But it does not possess the enzyme catalase like Propionibacterium acnes. 28 E – Microaerophile It grows and performs its vital activities in the presence of very small amounts of oxygen, as in Lactobacillus. Where their cells cells are equipped with some oxidation enzymes makes it very sensitive to large amounts of oxygen. To grow aerobic microbes, it is sufficient to leave the culture tubes under normal conditions to provide their oxygen needs The development of anaerobic microbes requires special methods, as atmospheric oxygen must be removed from the atmosphere surrounding the culturing the bacteria. Therefore, the following is done: 1. Adding some reducing compounds to the medium, such as sodium thioglycolate. Sodium Thioglycolate where oxygen is absent and the medium becomes anaerobic. 2. Automatically remove oxygen from microbial cultures by vacuuming (vacuuming) using an automatic vacuum pump and replacing air with nitrogen gas or a mixture of nitrogen and carbon dioxide. 3. Adding some chemicals that consume the oxygen of the medium during their reaction together. Inside closed vessels for microbial cultures, examples of materials added include a mixture of pyrogallic acid + potassium hydroxide. 6. Acidity or Alkalinity (pH) The effect of pH varies depending on the microbial type, as each microbial type has its own an optimum pH at which growth is maximum, and a minimum pH at which growth can occur, and a maximum pH which the highest pH that allows growth. The best pH for most bacteria is between (6.5 -8), but there are some Bacteria that deviate from this. Fungi prefer acidic environments (3.5 - 4) while Algae prefer alkaline media, i.e. eight and above. If you cultivate some microbes at pH = 7, this degree does not remain constant, but rather changes towards acidity or alkalinity because of the different metabolic products of these 29 bacteria, which affects their growth. Therefore, it is added sometimes, culture media contain buffer solutions that have the ability to: Combine with acids and alkalis, which maintain the pH of the medium during the growth period. These include KH2PO4 and K2HPO4. 7. Radiation Radiation is the invisible rays emitted by natural or artificial light. Some radiation has a harmful or deadly effect on microorganisms, so it is used in various sterilization processes. There are two types of radiation: Electromagnetic- Ionizing A. Ultraviolet rays Ultraviolet radiations are long-wave rays ranging between 2000 - 2950 angstroms, carrying a relatively large amount of energy that affects living cells in a harmful or fatal way. If the bacteria are exposed to these rays for a short time, the molecules of nucleic acids and proteins absorb a small amount of the rays, which leads to a change in some of their chemical bonds and increases the rate of mutations. However, if the exposure time to the rays is long, the molecules of nucleic acids and proteins are completely destroyed and damaged, which leads to cell death. Therefore, ultraviolet rays are used for sterilization in hospitals, microbiological and medical laboratories, and meat and food stores. B. Ionizing radiation: These are short-wave radiations ranging between 0.06 and 1000 angstroms. They carry a large amount of energy, which makes them highly penetrating into living tissues, leading to the ionization of nucleic acid molecules and the genes they carry, and thus destroying this molecular structure and the development of mutations that remain constant in all future generations. However, if the doses of radiation are large and the period of exposure of cells to them is also long, it will lead to inevitable death. Therefore, these types of radiation are used in the food industry and other medical purposes. 8. Effect of toxic chemicals: The effect of toxic substances on microorganisms varies according to their nature, concentration and duration of effect. They may stop the growth of microbial cells and hinder their reproduction, and are called bacteriostatic substances, as is the case with 30 some aniline dyes and sulfonamide compounds, or they may be lethal substances, and are called bactericidal substances, as is the case with halogens and their derivatives, heavy metal compounds, phenol and its derivatives, alcohols, disinfectants, lethal gases, and others. 31 Microbial metabolism The term metabolism is used to refer to the sum of all chemical reactions that take place in organisms. There are two types of metabolism: catabolism and anabolism. a- catabolism (referred to as energy-conserving reactions): The energy provided to the cell is released and conserved as ATP. These reactions involve the breakdown of complex organic molecules into smaller, simpler molecules. b- Anabolism: It is includes the synthesis of complex organic molecules from simpler ones. Anabolism requires energy (ATP) and a source of electrons stored in the form of reducing power. ENERGY AND WORK Energy can be defined as the ability to do work. Cells perform three main types of work, all of which are essential for life processes. 1-Chemical work: *Involves the synthesis of complex biological molecules from much simpler precursors (i.e., anabolism) *Energy is needed to increase the molecular complexity of a cell. 2-Transport work: *Energy is required to take up nutrients, remove wastes, and maintain ion balances. *Energy is needed because molecules and ions often must be transported across cell membranes against an electro chemical gradient. 3-Mechanical work: *Energy is required for cell motility and to transport structures within cells. *In living organisms, the form of energy is adenosine 5′-triphosphate (ATP). 32 ATP is a high-energy molecule. It breaks down to adenosine diphosphate (ADP), orthophosphate (Pi) and energy. ATP + H2O ADP+ Pi + Energy The energy released drives endergonic processes (reactions that require energy) such as anabolism, transport and mechanical work. Anabolic metabolism (Anabolism or Biosynthsis) It is a group of reactions that lead to the synthesis of complex organic molecules starting from small molecules such as NH3, NO3, CO2.....etc., as in the process of photosynthesis and chemosynthesis. Anabolic metabolism processes are often accompanied by the absorption of energy, and therefore they are endothermic reactions. Photosynthesis and chemosynthesis in bacteria Photosynthesis Some bacteria carry out photosynthesis exactly as in green plants, because they contain bacterial chlorophyll, which is distinguished from chlorophyll A by containing -CO- CH3 instead of -CH=CH2 in the first ring and hydrogen groups instead of C6H5 and CH3 in the second ring. Among the most important types of these bacteria are red sulfur bacteria and green sulfur bacteria. While plants use water, bacteria use hydrogen sulfide H 2S, and therefore oxygen is not released, but instead the element sulfur is formed, which is deposited in the red sulfur bacteria inside the cells and is released in the green sulfur bacteria into the external medium by the reaction: 33 6CO2 + 12H2O C6H12O6 + 6O2 + 6H2O Carbon dioxide + Water Glucose + Oxygen + Water There are two types of photosynthesis depending upon the production of oxygen, namely, oxygenic photosynthesis and anoxygenic photosynthesis. Oxygenic Photosynthesis Oxygenic photosynthesis is a photosynthetic process where carbon dioxide and water combine in the presence of sunlight to produce carbohydrates and oxygen. It is seen in green plants, algae, and cyanobacteria that contain chlorophyll. 6CO 2 + 12 H2O + Light → C6H12O6 + 6O2 + 6 H2O carbon dioxide + water + light energy glucose + oxygen + water Anoxygenic Photosynthesis Anoxygenic Photosynthesis is a photosynthetic process that does not produce oxygen. It is seen in certain bacterial groups that possess bacteriochlorophylls such as purple bacteria, green sulfur, heliobacteria, etc. CO2 + 2 H2A + Light → (CH2O) + 2A + H2O carbon electron light energy carbohydrate water dioxide doner* * H2A= H2O, H2S, H2 or other electron doner 34 Chemosynthesis Other bacterial species that do not contain bacterial chlorophyll fix and reduce CO2 gas without using light energy, but using chemical energy produced from certain inorganic materials. This process is called chemosynthesis, which means synthesizing carbon by reducing CO2 in the dark using the energy produced from the oxidation process. The most important groups of chemosynthetic bacteria are: 1. Nitrobacteria (Nitrifying bacteria) The oxidation of ammonium to nitrate takes place in two stages: Stage one: The oxidation of ammonium to nitrite is called nitrification, and is carried out by a typical genus, Nitrosomsnas, and other genera found in Soil, and can be represented by the following reaction: 2NH3 + 3O2 Nitrosomonas 2HNO2 + 2H2O + Energy Stage Two: The oxidation of nitrite to nitrate is called nitrification and is carried out by a typical genus, Nitrobacter, and other genera found in the soil, which can be represented by the following reaction: 2HNO2 + O2 Nitrobacter 2HNO3 + Energy The energy produced from the previous two reactions is used to complete reduction of CO2 like plants. 2. Thiobacteria (Sulfur bacteria) Most of these bacteria live in sulfurous water, some of them do not contain pigments and others contain non-green pigments. They oxidize hydrogen sulfide in two stages: 35 Stage one: It involves the oxidation of hydrogen sulfide and the formation of sulfur which appears as granules in Protoplasm of a bacterial cell, which can be represented by the following reaction: H2S + 1/2 O2 Sulpherbacteria S + H2O + Energy Stage Two: The resulting sulfur is oxidized to sulfuric acid according to the following reaction: 2S + 3O2 + 2H2O Thiobacillus thiooxidans 2H2SO4 + Energy Bacteria use the energy from oxidation to build sugars, starting from CO 2 dissolved in water or present in the atmosphere. 3. Ferrobacteria (Iron bacteria) These bacteria oxidize iron (II) compounds to its ternary compounds according to the following reaction: 4FeCO3+02+6H2O 4Fe(OH)3 + 4СО2+ Energy This is done by some genera, such as Leptothrix, in which the cells are surrounded by colloidal rust granules secreted by the bacteria. 4. Hydrogen bacteria Hydrogen bacteria obtain the energy needed for the chemical synthesis of organic matter in its cells by the oxidation of hydrogen, which is represented by the following reaction: 2H2+02 2H20 + Energy Some genera, such as Hydrogenomonas, do this. The previous reaction in the reduction of CO2 is as follows: 2H2 +СО2 (HCOH) + H20 + Energy This last energy-absorbing reaction is coupled with the first energy-producing reaction. 36 5. Methanobacteria Although not all methanogenic bacteria can be considered autotrophs, some of them depend on the oxidation of an organic compound, as is the case with Bacillus methanicus which oxidizes methane according to the following reaction: CH4 + 202 CO2 + 2H2O + Energy Then the resulting energy is used to reduce CO2, and another section produces methane and returns CO2, starting from the energy released from the oxidation of some mineral materials such as hydrogen, for example) present in the medium, as is the case in the genus Methanococcus, which is widespread in nature. Difference between photosynthesis and chemosynthesis Photosynthesis Chemosynthesis Light energy (sun) Chemical energy (H2S) CO2 + H2O C6H12O6 + O2 CO2 + H2S + O2 CH2O + S + H2O Oxygen is a product Water is a product Water is a reactant Oxygen is a reactant Chlorophyll needed Chlorophyll not required Any cell with Chlorophyll Chemosynthetic bacteria (algae, and some bacteria) 37 Catabolic metabolism (Catabolism) It is a set of reactions that lead to the decomposition of organic compounds formed by structural metabolism or provided as food into simpler ones. Catabolic metabolism processes are accompanied by the release of energy present in foods. In aerobic conditions, the decomposition is complete and the energy released is large, as in respiration, while in anaerobic conditions, the decomposition is incomplete and only part of the energy is released, as in fermentation. Carbon metabolism It includes all the successive chemical reactions which lead to transformations of sugars, and which provide the microbial cell with the energy necessary for its growth and continuation of life. This occurs through oxidation processes during aerobic respiration, anaerobic respiration or fermentation. I- Aerobic respiration Microorganisms obtain energy in the presence of oxygen through cellular aerobic respiration. The ability of bacteria to consume types of sugar shows that they have enzymes that allow them to decompose sugar, such as glucose, which is oxidized in the presence of oxygen as the final oxidizing agent (acceptor). Aerobic respiration in prokaryotes occurs in three stages: 1-The first stage is the anaerobic glycolysis stage 2-The second stage is the Krebs cycle (citric acid cycle) 3-The third stage is the electron transport chain 38 1- Glycolysis: Glycolysis is the process of breaking down sugar. It is a process in which a glucose molecule (6 C) is converted into two molecules of pyruvic acid, by means of a series of enzymatic reactions for the purpose of obtaining energy. This process occurs either aerobically or anaerobically. This cycle is linked to another cycle, which is Tricarboxylic acid cycle which can oxidize pyruvate to CO2 and water. This can follow one of three paths: The first path is called Embden-Meyerhof-Parnsa (E.M.P) pathway and is found in yeasts, bacteria, and almost all living organisms. The second is the Pentose phosphate pathway and is found in some microorganisms. The third path is the Entner –Doudoroff pathway (E.D) and is found only in bacteria. In the successive glycolysis reactions, there are three types of chemical transformations: 1-Breaking down the carbon skeleton of glucose into pyruvate. 2- Phosphorylation of ADP to ATP by high-energy compounds formed during glycolysis. 3- Transfer of hydrogen ions to NAD to form NADH. i- Embden-meyerhof pathway Microorganisms break down glucose and obtain pyruvic acid by converting the glucose molecule, in the presence of energy in the form of ATP, into glucose-6-phosphate. The net reaction is as follows: Glucose + 2NAD+ + 2 ADP + 2H3PO4 2 Pyruvate + 2 NADH + 2H+ + 2 ATP Each NADH is equivalent to 3ATP, so that the net gain in glycolysis is 8ATP. 39 Embden-meyerhof pathway 40 ii- Pentose phosphate pathway (Hexose monophosphateshunt) A second glycolysis pathway, may be used at the same time as either the EMP or the Entener-Doudoroff pathway. It can operate either aerobically or anaerobically, is the major source for NADPH require for anabolic processes. The PPP is a good example of an amphibolic pathway as it has several catabolic and anabolic functions. There are two distinct phases in PPP; oxidative phase, The pathway begins with the oxidation and decarboxylation of glucose-6-phosphate (G- 6-P) to 6- phosphogluconate followed by the oxidation of 6- phosphogluconate to the ribulose 5- phosphate, CO2 and NADHs are generated during these oxidations. The overall reaction for this phase is: 3 G-6-P + 6 NADP +H2O ribulose 5- phosphate + 3CO2+ 6 NADPH + 6H+ non – oxidative phase: includes non – oxidative reactions converted Ribulose 5- phosphate to a mixture of three, four, five, six, and seven – carbon sugars. The overall result is that three G-6-P are converted to: 3 G-6-P + 6NADP + 3H2O 2 F-6-P + G-3-P + 3CO2 + 6 NADPH + H+ These intermediates are used as the following: fructose 6- phosphate can be altered back to G-6-P, glyceraldehyde-3-phosphate (G3P) can be to pyruvate by enzymes of the EMP or Alternatively two G3P may combine to form fructose 1,6- biphosphate. 41 The Pentose Phosphate Pathway 42 iii- Enter-Doudoroff pathway In this pathway, the first five steps produce new intermediate, 2- keto-3-deoxy 6- phosphogluconic acid (KDPG). It splits into one pyruvate with glyceraldehyde 3-phosphate. In these steps, ATP is released, and then the latter completes the five steps of the glycolysis cycle or Embden- Meyerhof pathway These reactions occur in a number of Gram-negative bacteria, particularly among members of the genera Pseudomonas and Azotobacter, but can also occur among members of Gram-positive bacteria, such as lactate-producing organisms such as Streptococcus faecalis, when grown on gluconitol. It is used by only prokaryotes and is not used by eukaryotes. If the Entner – Doudoroff pathway degrades glucose to pyruvate in this way, a net yield 1 ATP for every one glucose molecule processed, as well as 1 NADH and 1 NADPH. Enter-Doudoroff pathway 43 Oxidative decarboxylation of pyruvate Pyruvate is converting into acetyl-CoA and produces one NADH electron carrier while releasing a CO2 molecule. This step is also known as the link reaction or transition step, as it links glycolysis and the citric acid cycle. As glycolysis results in two pyruvates, two acetyl-CoAs and 2 NADH molecules are produced. The overall reaction may be written as follow: CH3COCOOH + CoA –SH + NAD+ CH3CO –CoA + CO2 + NADH + H+ Pyruvic acid Cocenzyme A Accetyl Conenzyme A 2-Tricarboxylic Acid Cycle (TCA) The tricarboxylic acid (TCA) cycle, also known as the Krebs or citric acid cycle, is a series of chemical reactions used to generate energy through the oxidation of acetate (acetyl-CoA) derived from carbohydrates, fats and proteins in to CO2 and chemical energy in the form of ATP. The TCA cycle consists of eight steps catalyzed by eight different enzymes. The reaction sequence is called a ‘cyclic’ because the end product (the 4-carbon oxaloacetic acid) combines with more of the starting material (acetyl CoA) and reenters the reaction sequence as soon as it is formed. TCA cycle enzymes are located in the cytoplasmic matrix of prokaryotic cells and are found in the mitochondrial matrix of eukaryotic cells. TCA cycle enzymes are widely distributed among microorganisms. In prokaryotes, they are located in the cytoplasmic matrix. In eukaryotes, they are found in the mitochondrial matrix. The citric acid cycle occurs in the matrix of the mitochondria of eukaryotic cells and in the cytoplasm of bacteria, where a series of enzyme-catalyzed reactions take place. The net output from one cycle is: 2 CO2, 3 NADH, 1 FADH2, 1 GTP (converted to ATP). 44 Tricarboxylic Acid Cycle (TCA) 45 The Krebs cycle provides carbon skeletons for building many compounds, such as some amino acids. 3- Terminal Oxidation It is the name of oxidation found in aerobic respiration that occurs towards the end of catabolic process and involves the passage of both electrons and protons of reduced coenzymes to oxygen. It consists of two processes: (i) Electron transport and (ii) Oxidative phosphorylation. Electron transport system (ETS) Overall, the electron transport system (ETS) consists of four major enzyme complexes (called complexes I-IV) along with some electron carriers that receive electrons from reduced carriers (NADH, FADH2) and move the electrons from one complex to the next until finally reaching oxygen. Oxidative phosphorylation Oxidative phosphorylation denotes the phosphorylation of ADP into ATP. As the electron transport carriers transport electrons, they actively pump hydrogen ions (protons) into the outer compartment of the mitochondrion. This process set up a concentration gradient of hydrogen ions called the proton motive force (PMF). The ATP synthase complex transport hydrogen ions (protons) from outside compartment back into the mitochondrial matrix. This process releases sufficient free energy for the synthesis of ATP from an ADP and pi. Each NADH that enters electron transport gives rise to 3 ATPs. while FADH2 synthesize 2 ATPs. Yield of ATPs from oxidative phosphorylation 46 The total of five NADHs (4 from TCA cycle and one from glycolysis) can be used to synthesize: 15 ATPs from ETS (5×3 pre electron pair) And 15 × 2 = 30 ATPs per glucose The single FADH2 producing during the TCA cycle results in : 2 × 2 = 4 ATPs per glucose Summary of aerobic respiration (1) The total yield of ATP is 40 molecules: 4 from glycolysis, 2from TCA cycle, and 34 from electron transport. However, since 2 ATPs were expended in early glycolysis, these have a maximum of 38 ATPs. The actual number may be lower in certain eukaryotic cell and bacteria. (2)Six carbon dioxide molecules are generated during TCA cycle. (3)Six oxygen molecules are consumed during electron transport. (4)Six water molecules are reduced in electron transport and one in glycolysis, but one is send in the TCA cycle, this leaves a net of 6. 47 The Chemiosmotic Hypothesis Applied to Mitochondria The Aerobic Respiratory System of E. coli. 48 ATP yield from Aerobic Respiration 49 II- Anaerobic respiration Cellular respiration is generally associated with the cytoplasmic membrane. In the case of anaerobic respiration, the final acceptor of electrons is represented by inorganic compounds other than oxygen. The electron transport chain in this case is similar to that operating in aerobic respiration except for the final oxidizer. Among the electron acceptors in this field that enter into the processes of anaerobic respiration are nitrate NO3 and sulfate SO4. E. coli bacteria can grow anaerobically when glucose is oxidized using nitrate as the final acceptor of electrons. As a result of this oxidation process, we produce nitrate derivatives NO2 and nitrogen gas N2. Among the anaerobic bacteria that reduce sulfates is Desulfovibrio sulfuricans bacteria. It respires by reducing sulfate SO4 to sulfide ions S in the form of H2S or to atomic sulfur S. This process of reducing sulfates to hydrogen sulfide is the reason for the blackening of the mud sometimes, in addition to the blackish color of the Black Sea, as hydrogen sulfide reacts with the ferrous ion +Fe to form the black ferrous sulfide salt. 50 III- Fermentation Fermentation is another anaerobic (non-oxygen-requiring) pathway for breaking down glucose performed by many microorganisms. These microbes either they lack electron transport chain or they repress the synthesis of electron transport chain component under aerobic conditions. In fermentation, the pyruvate made in glycolysis does not continue through the citric acid cycle and the electron transport chain. Many microorganisms use pyruvate or one of its derivatives as an electron acceptor for reoxidation of NADH in fermentation process. Major pathways for fermentation of sugars 1-Lactic acid fermentation This fermentation occurs in some Bacillus some Lactobacillus and lead to Cheese production. In lactic acid fermentation, NADH transfers its electrons directly to pyruvate, generating lactate as a byproduct. C6H12O6 (glucose) 2 CH3CHOHCOOH (lactic acid) 2-Alcohol fermentation Yeast and certain bacteria perform ethanol fermentation where pyruvate (from glucose metabolism) is broken into ethanol and carbon dioxide NADH donates its electrons to a derivative of pyruvate, producing ethanol in two-step process. 51 i- Carboxyl group is removed from pyruvate and released in as carbon dioxide, producing a two-carbon molecule called acetaldehyde. ii- acetaldehyde is reduced to ethanol in the presence of NADH. C6H12O6 (glucose) 2 C2H5OH (ethanol) + 2 CO2 (carbon dioxide) 3- Heterolactic lactic fermentations: can be produced in Anaerobic conditions in addition to lactic acid produce ethanol, acetic acid, and CO2, and glycerol can also be produced. 4- Butyric fermentation and fermentations derived from it. In this type of fermentation, bacteria produce Butyric C.butyricum, including Clostridium, such as the genus CO2, butyrate and gas (acid). 5- Propionic fermentation: Produced by adding propionic acid. Trace amounts of carbon dioxide and acetic acid such as Propionibacterium 6-Butanediol fermentation Butanediol fermentation is the anaerobic fermentation of glucose using 2,3-butanediol as one of the end products as well as the production of some ethanol, lactic acid and formic acid. It can occur in the genera Klebsiella and Enterobacter and is tested using Voges-Proskauer test. 52 Some Common Microbial Fermentations 53 Catabolism of carbohydrates other than glucose Monosaccharides The catabolic pathways for the monosaccharides glucose, fructose, mannose, and galactose include interconversion to glucose or glucose deriatives. The first three are phosphorylated using ATP and easily enter the Embden-Meyerhof pathway. In contrast, galactose must be converted to uridine diphosphate galactose after initial phosphorylation, then changed into glucose 6-phosphate. Disaccharides The common disaccharides are cleaved to monosaccharides by two mechanisms: 1-Hydrolysis Maltose, sucrose, and lactose can be directly hydrolyzed to their constituent sugars. 2- Phosphorolysis Maltose, cellobiose, and sucrose are also split by a phosphate attack on the bond joining the two sugars. Polysaccharides Polysaccharides, like disaccharides, are cleaved by both hydrolysis and phosphorolysis. Procaryotes and fungi degrade external polysaccharides by secreting hydrolytic enzymes. These exoenzymes cleave polysaccharides into smaller molecules that can be assimilated. Starch and glycogen They are hydrolyzed by amylases to glucose, maltose, and other products. Cellulose Many fungi and a few produce extracellular cellulases that hydrolyze cellulose to cellobiose and glucose. 54 Pectin Many soil bacteria and bacterial phytopathogens degrade pectin (constituent of plant cell walls) into units of galacturonic acid (a galactose derivative). Lignin Certain fungi that release peroxide-generating enzymes can degrad lignin Agar Some actinomycetes and members of Cytophaga, excrete an agarase that degrades agar. Reserve Polymers Microorganisms in the absence of exogenous nutrients catabolize intracellular stores of glycogen, starch, poly-β-hydroxybutyrate, and other carbon and energy reserves. Glycogen and starch They are dgraded by phosphorolysis which catalyzed by phosphorylases. This shortens the polysaccharide chain by one glucose and yields glucose 1-phosphate. (Glucose)n + Pi ⎯⎯→ (glucose)n-1 + glucose-1-P Glucose 1-phosphate can enter glycolytic pathways by way of glucose 6-phosphate. Poly- β-hydroxybutyrate (PHB) The soil bacterium Azotobacter hydrolyzes PHB to 3-hydroxybutyrate, then oxidizes the hydroxybutyrate to acetoacetate. Acetoacetate is converted to acetyl-CoA, which can be oxidized in the TCA cycle. 55 Carbohydrate Catabolism. Examples of enzymes and pathways used in disaccharide and monosaccharide catabolism. UDP is an abbreviation for uridine diphosphate. 56 Nitrogen metabolism Some heterotrophic microorganisms have the ability to consume nitrogen in its various forms found in nature, so microbial species differ in their ability to use a particular form of nitrogen and in the type of proteins and enzymes they contain. A. Atmospheric nitrogen Some heterotrophic bacteria can use the atmospheric nitrogen as N2 and reduce it to ammonia. This reduction of nitrogen to ammonia is called nitrogen fixation. NH3 is not liberated by the nitrogen fixers. It is toxic to the cells and therefore these fixers combine NH3 with organic acids in the cell and form amino acids. The general equation for nitrogen fixation may be described as follows: N2 + 8e– + 8H+ + 16ATP 2NH3 + H2 + 16ADP + 16Pi Some of the N2–fixers can be symbiotic (e.g Rhizobium) or free-living (e.g. Azotobacter, Bacillus, Anabaena, Nostoc). B. Organic nitrogen Proteins and amino acids Some bacteria and fungi can use proteins as their source of carbon and energy. They secrete protease enzymes that hydrolyze proteins and polypeptides to amino acids, which are transported into the cell and catabolized. The first step in amino acid use is deamination, the removal of the amino group from an amino acid. This is often followed by transamination. The amino group is transferred from an amino acid to an α-keto acid acceptor. The organic acid resulting from deamination can be oxidized in the TCA cycle to release energy. It also can be used as a source of carbon for the synthesis of cell constituents. Excess nitrogen from deamination may be excreted as ammonium ion, thus making the medium alkaline. 57 C. Inorganic nitrogen The ability of an organism to utilize other forms of inorganic nitrogen depends on the presence of enzymes or enzyme systems that are able to convert these compounds to ammonia. Ammonia Some soil and water microbes can use inorganic materials as a source of energy (electrons) during their growth. Nitrifying bacteria can convert ammonium resulting from the decomposition of organic matter in the soil into nitrite and nitrate by means of Nitrosomonas and Nitrobacter bacteria as we explained previously. (i) First, ammonia is oxidised to nitrite by the bacteria Nitrosomonas and/or Nitrococcus. 2NH3 + 3O2 → 2NO2– + 2H+ + 2H2O (ii) Then, nitrite is further oxidised to nitrate by Nitrobacter. 2NO2– + O2 → 2NO3– The ability of an organism to utilize other forms of inorganic nitrogen depends on the presence of enzymes or enzyme systems that are able to convert these compounds to ammonia. Nitrate Nitrogen Nitrates in the soil are also reduced to nitrogen during Denitrification by Pseudomonas and Thiobacillus. NO3– → NO2– → NO → NO2 → N2 58 Nitrate and Ammonia assimilation Nitrate is absorbed and reduced to ammonia with the help of two different enzymes. The first step conversion of nitrate to nitrite is catalyzed by an enzyme called nitrate reductase. NO3 - + NADH + H+ nitrate reductase NO2 - + NAD+ + H2O In the second step the nitrite so formed is further reduced to ammonia and this is catalyzed by the enzyme nitrite reductase. NO2 - + 3NADPH + 3 H+ nitrite reductase NH3 + 3NADP+ Ammonium produced is the major source of amino group. There are two major reactions for amino acid biosynthesis: i. Reductive amination reaction: In this reaction, ammonia combines with a keto acid. The most important keto acid is the alpha ketoglutaric acid produced during Krebs cycle. The keto acid then undergoes enzymatic reductive amination by glutamate dehydrogenase to produce an amino acid. 59 α-ketoglutaric acid + NH3 → glutamate dehydrogenase → Glutamic (keto acid) (amino acid) Similarly aspartic acid is produced by reductive amination of oxaloacetic acid. ii-Transamination reaction The reaction involves transfer of amino group, from an amino acid, to the keto acid. α-Ketoglutaric acid + Aspartic acid →Transaminase ⎯⎯⎯⎯⎯⎯→ Glutamic acid + Oxaloacetic acid (Keto acid) (Amino acid) (Amino acid) (Keto acid) In the above reaction, aspartic acid has transferred its amino group (NH 2) to the α- ketoglutaric acid to synthesize glutamic acid and release keto acid. The reaction is catalyzed by enzymes called transaminases. A large number of amino acids are synthesized by this transamination reaction. Amino acids are organic molecules containing nitorgen. The incorporation of amino group, from ammonium, into keto acids represents the major step for synthesis of nitrogenous organic biomolecules. Phosphorus metabolism Phosphorus enters into the production of many important organic compounds in the living cell such as ATP, nucleic acids and cell membranes, and therefore plays an important physiological role. Some types of bacteria can mineralize organic phosphorus present in the surrounding environment. Other microbial species that produce organic acids break down insoluble phosphate and make it soluble (monobasic and dibasic phosphate HPO42-, H2PO4- ) and usable by other organisms. It is worth noting that the amount of phosphate inside the microbial cell remains constant despite its changes in the external environment, as some of it enters into cellular metabolic processes and is stored in the form of polyphosphates that are used when needed. 60 Sulfur metabolism Some microbes are able to oxidize some sulfur compounds to obtain the energy stored in those compounds. Some bacteria such as Bacillus subtilis and E. coli can break down proteins or sulfur amino acids aerobically or anaerobically, producing free sulfur or H 2S, as in the breakdown of the amino acid cysteine into pyruvic acid or the amino acid serine according to the microbial enzyme: Then water bacteria such as Beggiatoa and Thiothrix oxidize H2S to water and sulfur: 2H2S + ½ O2 2H2O + 2S + Energy By this energy, bacteria can produce sugars. Some species of the genus Thiobacillus found in soil can oxidize various sulfur compounds to sulfate. 61 Secondary metabolism Microorganisms produce a range of metabolites in their lifecycle. Microbial secondary metabolites are formed during the end or near the stationary phase of growth. They are not essential for growth of the producing cultures. Its synthesis can be influenced greatly by manipulating the type and concentration of the nutrients formulating the culture media. Among these nutrients, the effect of the carbon sources has been the subject of continuous studies for both, industry and research groups. They include antibiotics, antitumor agents, cholesterol-lowering drugs, pigments, plant hormones and mycotoxins. These are a heterogeneous group of natural products that include terpenoids and steroids, alkaloids, fatty acid-derived substances and polyketides, non-ribosomal polypeptides, and enzyme cofactors. The pathways and precursors of secondary metabolism A few key intermediates of the basic metabolic pathways provide the starting points for the pathways of secondary metabolism. Some of the more important examples are shown below. 1-Polyketide pathway: The most important secondary metabolic pathway. In this case the precursor is acetyl- CoA, which is carboxylated to form malonyl-CoA (a normal event in the synthesis of fatty acids), then three or more molecules of malonyl- CoA condense with acetyl-CoA to form a chain. This chain undergoes cyclization, then the ring systems are modified to give a wide range of products (antibiotics, aflatoxins, ochratoxins and patulin). 2- Isoprenoid pathway Again, acetyl-CoA is the precursor, but three molecules of this condense to form mevalonic acid (6-carbon) which is converted to a 5-carbon isoprene unit. Then the isoprene units condense to form chains and undergo cyclization. The products of this pathway include the mycotoxins T-2 and the carotenoid pigments. 62 3- Shikimic acid pathway It used normally for the production of aromatic amino acids, provides the precursors for the secondary metabolites. 4- Aliphatic amino acids This pathway lead to production of penicillins, amatoxins and phallotoxins. 63 Regulation of transcription initiation Induction and Repression of Enzyme Synthesis Constitutive enzymes They are produced almost all of the time because they catalyze reactions in the cell. They include those of the central metabolic pathways and their functions are referred to as housekeeping functions. They are encoded by genes often referred to as housekeeping genes. These genes are expressed continuously are said to be constitutive genes. Inducible enzymes Their level rises in the presence of a small effector molecule called an inducer. They include β-galactosidase which catalyzes the hydrolysis of the disaccharide lactose to glucose and galactose. They are encoded by genes are expressed only when needed and are referred to as inducible genes. The enzyme β-galactosidase is an inducible enzyme—that is, its level rises in the presence of a small effector molecule called an inducer (in this case the lactose derivative allolactose). β-galactosidase is an enzyme that functions in a catabolic pathway and many catabolic enzymes are inducible enzymes. Inducible enzymes are required only when their substrate is available; they are missing in the absence of the inducer. 64 Enzymes involved in the biosynthesis of amino acids and other substances, on the other hand, are often called repressible enzymes. For instance, an amino acid present in the surroundings may inhibit the formation of enzymes responsible for its biosynthesis. Generally, repressible enzymes are necessary for synthesis and always are present unless the end product of their pathway is available. Control of Transcription Initiation by Regulatory Proteins Regulatory proteins are often responsible for induction and repression. Regulatory proteins can exert either negative or positive control. Negative transcriptional control Regulatory proteins inhibit initiation of transcription. These proteins are called repressor proteins. Positive transcriptional control Occurs when the regulatory proteins promotes transcription initiation. These proteins are called activator proteins. Repressor and activator proteins usually act by binding DNA at specific sites. Repressor proteins bind the operator which usually overlaps or downstreams the promoter. Thus, the repressor either blocks binding of RNA polymerase to the promoter or prevents its movement. Activator proteins bind activator-binding sites. These are often upstream of the promoter. Thus, the binding of an activator to its regulatory site promotes RNA polymerase binding. Repressor and activator proteins must exist in both active and inactive forms. The activity of regulatory proteins is modified by small effector molecules. There four basic ways in which the interactions of an effector and a regulatory protein can affect transcription: 65 (1) For negatively controlled inducible genes (e.g., those encoding enzymes needed for catabolism of a sugar), The repressor protein is active and prevents transcription when the substrate of the pathway is not available. It is inactivated by binding of the inducer (e.g., the substrate of the pathway). (2) For negatively controlled repressible genes (e.g., those encoding enzymes needed for the synthesis of an amino acid). The repressor protein is in an inactive form called the aporepressor. It is activated by binding of the corepressor. The corepressor is often the product of the pathway (e.g., an amino acid). (3) Positively regulated inducible gene The activator is activated by the inducer. (4) Positively regulated repressible gene The activator is inactivated by an inhibitor. The structural genes— the genes coding for polypeptides—are simply lined up together on the DNA, and a single, polycistronic mRNA carries all the messages. The sequence of bases coding for one or more polypeptides, together with the promoter and operator or activator-binding sites, is called an operon. 66 The Lactose Operon: Negative Transcriptional Control of Inducible Genes Lactose (lac) operon of E. coli is negative control system. The lac operon contains three structural genes controlled by the lac repressor, which is encoded by lacI. One gene codes for β-galactosidase; a second gene directs the synthesis of permease, the protein responsible for lactose uptake. The third gene codes for the enzyme β-galactoside transacetylase, whose function still is uncertain. E. coli can use lactose as a carbon and energy source. When there is no lactose, the lac repressor binds the operator sites. This prevents initiation of transcription either because RNA polymerase cannot access the promoter or because it is blocked from moving into the coding region. When lactose is available, it is taken up by the lactose permease. Once inside the cell, β- galactosidase converts lactose to allolactose, the inducer of the operon. This occurs because, as there is always a low level of permease and β -galactosidase synthesis. Allolactose binds to the lac repressor and causes the repressor to change to an inactive shape that is unable to bind any operator sites. The inactivated repressor leaves the DNA and transcription occurs. 67 The Tryptophan Operon: Negative Transcriptional Control of Repressible Genes The tryptophan (trp) operon of E. coli consists of five structural genes that encode enzymes needed for synthesis of the amino acid tryptophan. It is regulated by the trp repressor, which is encoded by the trpR gene. Because the enzymes encoded by the trp operon function in a biosynthetic pathway, it is wasteful to make the enzymes needed for tryptophan synthesis when tryptophan is readily available. Therefore, the operon functions only when tryptophan is not present. The trp repressor is synthesized in an inactive form that cannot bind the trp operator as long as tryptophan levels are low. When tryptophan levels increase, tryptophan acts as a corepressor, binding the repressor and activating it. The repressor-corepressor complex then binds the operator, blocking transcription initiation. 68