Stem Cells_2024_v2.pdf
Document Details
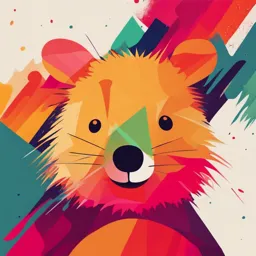
Uploaded by UnquestionableKremlin
Rutgers University
2024
Tags
Full Transcript
Diego Fraidenraich [email protected] _____________________________________________ Stem cells Stem Cells and Self-Renewal * specialized cells that continually self-divide and generate progeny cells for organ formation and maintenance * found in different tissues at all stages of life, before...
Diego Fraidenraich [email protected] _____________________________________________ Stem cells Stem Cells and Self-Renewal * specialized cells that continually self-divide and generate progeny cells for organ formation and maintenance * found in different tissues at all stages of life, before and after birth * different types (location in the body, type of cells they can produce) 1) Embryonic stem cells: very early embryos 2) Adult stem cells: within tissues of children and adults. Adapted from the ISSCR (International Society for Stem Cell Research) web site 1 1 Objectives Embryonic Stem Cells Adult Stem Cells Induced Pluripotent Stem Cells Transdifferentiation (direct differentiation) Similarities and differences Advantages and disadvantages 2 2 Potency The potential of a cell to differentiate into various cell types – Totipotency - ability to differentiate into all cell types of an organism Includes extraembryonic tissues (yolk sac, amnion) Example: morula – Pluripotency - ability to differentiate into all three germ layers Endoderm, Mesoderm, Ectoderm Excludes extraembryonic tissues Examples: ESCs, iPSCs, etc. – Multipotency - ability to differentiate into multiple, limited cell types Examples: HSCs, MSCs Oligopotency – minor subset of multipotency with limited potential – Unipotency – only differentiates into one cell type Most common in adults Embryonic stem cells * cultured cell lines derived from the inner cell mass of the blastocyst * can be grown indefinitely in their undifferentiated state * capable of differentiating into all cells of the adult body (pluripotency). Blastocyst (pre-implantation stage), source of embryonic stem cells 4 3 ESCs can only be derived from cells found in the embryo (ethical concerns). If a blastocyst is implanted back into the mother for pregnancy, it could develop into a fetus and then into a newborn baby embryo extra-embryonic tissues 5 4 ESCs remain the only SC type demonstrated to produce all the approximately 200 cell types found within the adult body. Inefficient process 12 mice superovulated 97 blastocysts 20 ESCs 3 ESCs with normal karyotype 6 5 Human ESCs and IVF Not all eggs will fertilize and develop properly into blastocysts during an IVF procedure. Not implanted blastocysts are frozen for another pregnancy, and ultimately discarded. These discarded blastocysts have been used to derive human embryonic stem cells in the laboratory, after approval of the donors and oversight by the appropriate IRB or ethics committees IVF 7 6 The value of ESCs for research and therapy * Potential to differentiation into all functional cell types. * Culture methods: ESCs into brain, heart, muscle cells, blood cells, blood vessels, skin, islet cells and bone cells (3 germ layers). * Efficiency during derivation, contamination with other cell types, scaling, dedifferentiation, potential for teratoma formation (benign tumor). * Research with ESCs will help to discover the factors necessary for regeneration and repair of tissues (drug discovery). * Growth of entire hearts, livers and even kidneys. Three-dimensional matrices (polymer matrices) 8 7 Adult stem cells * derived from different parts of the body (svz of brain, bone marrow, adipocyte) * have different properties. * limited number of cell types related to the tissue that the SCs originally came from.) Hematopoietic stem cells * adult SCs found mainly in the bone marrow * form the blood cells * required for daily blood turnover and * for fighting infections. * easy to obtain, bone marrow aspiration or stimulation with cytokines (G-CSF) to move into the peripheral blood stream * HSCs were the first SCs to be used successfully in therapies (blood cancers, e.g. leukemia) and other blood disorders. 9 8 Example of a treatment of a leukemia patient 11 10 Mesenchymal stem cells * A well-characterized population of adult stem cells. * Found in the bone marrow, can form fat cells, cartilage, bone, tendon and ligaments, muscles cells, skin cells and even nerve cells. * Large quantities. * Maintained and propagated in culture for long periods of time. * Can be genetically manipulated (incorporation of DNA sequences) * Tumorigenic properties if improperly cultured. * Clinical trials are underway in several clinical centers. 12 11 Separation by adhesion to plate fat cells, cartilage 13 12 Umbilical cord blood stem cells * Immediately after birth (neonatal stem cells). * Rich source of hematopoietic stem cells. * Less mature than adult SCs. * non-invasive procurement and vast abundance. * Collection and banked * Alternative source for the treatment of leukemia and other blood disorders. (does not produce strong graft-versus-host disease) 14 13 Examples of adult stem cells Emerging concept: plasticity SVZ: subventricular zone (lateral ventricles) Cardiac stem cells The heart is uncapable of self-repair To treat leukemia, lymphoma Also MSCs: to replace tendons, bones, ligaments cardiomyocytes (controversial) 15 14 Adult stem cells In the niche * Renew indefinitely * Mature into more specialized, organ specific cells. * Asymmetric division (one of the daughter retains the stem cell characteristics, the other is destined for a limited number of future divisions and will produce the more organ-specific cells) However, in the petri dish… * Human hematopoietic SCs cannot renew in culture without much success. * Cocktail of growth factors increase the number of passages of HSCs * Unlike adult stem cells, embryonic stem cells renew indefinitely in the petri dish 16 15 Somatic cell nuclear transfer (SCNT) or therapeutic cloning. *To avoid rejection possibilities: “custom” embryonic stem cells or patient-specific embryonic stem cells, matching the patient’s immunological profile. * The DNA from any one cell in the body of a patient (usually a skin or muscle cell) could be removed and transferred into an unfertilized egg that previously had its own DNA removed * In a culture dish, the unfertilized egg is then coaxed into developing as if it had been fertilized. * Generation of ESCs (5-6 days) * The ESCs can now be used to generate cells and tissues for the patient * Successful in mice and dogs, and recently in humans * SCNT using somatic cells from diabetic patient (Egli) 17 16 Fix deficiency by gene-therapy 18 17 Electrical pulse and nutrition supplement to facilitate cell fusion and escape from the quiescent 19 https://doi.org/10.1016/j.cell.2018.01.02 0 20 Ethical concerns (humans) Limited supply of eggs * animal eggs *ESC generation can now be done with Federal funding, but donors can not be compensated and therefore no source of eggs is available; Teratoma potential of ESC lines derived Patient-specific (SCNT) hESCs is now possible. SCNT could not be done with human ESCs yet (Hwang- South Korea) Recent experiments show that it is possible to obtain NT-ESCs 21 18 Alternative to ESCs Induced pluripotent stem cells (iPS cells) New generation of stem cells * Dedifferentiation is possible through cloning. Dolly the sheep (Wilmut) * Minimal set of transcription factors are required dedifferentiate an adult cell (Oct3/4, Sox2, c-myc and Klf4) Mouse (Yamanaka, Jaenisch) and human iPS cells (Yamanaka, Thomson) Rigorous assays for pluripotency: morphology, expression profile, methylation pattern, teratomas when ectopically injected in mice, chimeras in mice, germ line transmission in mice, in vitro differentiation into several cell types * Proof of principle experiment: a sickle cell anemia mouse model was rescued after transplantation with murine hematopoietic progenitors obtained from iPS cells (Jaenisch) *Disease modeling in the Petri dish (Long QT syndrome with cardiac myocytes, 22 ALS with neurons) 19 Are iPSCs as good as ESCs? Tumors associated to c-myc re-expression Incorporation of retroviral sequences Random integration of dedifferentiation factors (high clonal variability) Low efficiency (0.1%) Mutations in somatic (skin) cells Epigenetic memory Differentiation potential, variation from colony to colony (even from technician to technician) immature character of differentiated cells (for example cardiac myocytes) Alternatives: other approaches for reprogramming, replacement with small molecules, modified-RNA, proteins, miRNA 23 20 Epigenetic modification on DNA and Histone DNA: methylation = inactivation Histone (vary!): methylation ≈ inactivation acetylation ≈ activation The Epigenetic modification is heritable! 24 Each cell population has an epigenetic pattern that correlates with developmental potential Epigenetic reprogramming and induced pluripotency Konrad Hochedlinger1 and Kathrin Plath2 25 26 21 Published June 2014 in Nature Grafting of hESC-derived cardiomyocytes in a model of myocardial ischemia – Biggest challenge: scale Mice require ~1 million cells for a successful graft Humans would require over 1 billion cells to achieve similar results – Major findings: Cardiomyocytes can be differentiated and cryopreserved until needed Grafting 1 billion cells in a non-human primate heart produces significant benefit! New concept: transdifferentiation No need to reprogram to pluripotent state This process circumvents teratoma formation Pancreatic beta cells (insulin-secreting) from pancreatic exocrine cells (Melton) Cardiac myocytes (Tbx5, Mef2C, Gata4) from cardiac fibroblasts (Srivastava) Cardiac myocytes (Yamanaka factors), poor serum conditions (no LIF) and cardiac secreted factors (Ding) Hematopoieic progenitors from skin fibroblasts (Bhatia) Motorneurons from fibroblasts (Wernig, Eggan) Promising approach but still at early stages 34 22 Example of a rigorous study to demonstrate transdifferentiation ransdifferentiation (direct differentiation): from cardiac fibroblasts to cardiac myocy Now direct differentiation is possible with a combination of 9 chemicas (9C) Screening for Cardiomyocyte-Inducing Factors Combination of Three Transcription Factors Induces Cardiac Gene Expression in Cell 2022 Sep 1;185(18):3290-3306.e25. doi: 10.1016/j.cell.2022.07.028. Epub 2022 Aug 1. Post-gastrulation synthetic embryos generated ex utero from mouse naive ESCs Shadi Tarazi 1, Alejandro Aguilera-Castrejon 2, Carine Joubran 3, Nadir Ghanem 4, Shahd Ashouokhi 3, Francesco Roncato 3, Emilie Wildschutz 3, Montaser Haddad 5, Bernardo Oldak 3, Elidet Gomez-Cesar 3, Nir Livnat 3, Sergey Viukov 3, Dmitry Lokshtanov 3, Segev Naveh-Tassa 3, Max Rose 3, Suhair Hanna 6, Calanit Raanan 7, Ori Brenner 7, Merav Kedmi 8, Hadas Keren-Shaul 8, Tsvee Lapidot 5, Itay Maza 9, Noa Novershtern 10, Jacob H Hanna 11 Abstract In vitro cultured stem cells with distinct developmental capacities can contribute to embryonic or extraembryonic tissues after microinjection into pre-implantation mammalian embryos. However, whether cultured stem cells can independently give rise to entire gastrulating embryo-like structures with embryonic and extraembryonic compartments remains unknown. Here, we adapt a recently established platform for prolonged ex utero growth of natural embryos to generate mouse post-gastrulation synthetic whole embryo models (sEmbryos), with both embryonic and extraembryonic compartments, starting solely from naive ESCs. This was achieved by co-aggregating non-transduced ESCs, with naive ESCs transiently expressing Cdx2 or Gata4 to promote their priming toward trophectoderm and primitive endoderm lineages, respectively. sEmbryos adequately accomplish gastrulation, advance through key developmental milestones, and develop organ progenitors within complex extraembryonic compartments similar to E8.5 stage mouse embryos. Our findings highlight the plastic potential of naive pluripotent cells to self-organize and functionally reconstitute and model the entire mammalian embryo beyond gastrulation. Keywords: ESCs; PGCs; embryogenesis; embryoids; ex utero; extra-embryonic Tissues; iPSCs; naive 38 pluripotency; synthetic embryos. 2022 no egg, no sperm Prof Magdalena Zernicka-Goetz U of Cambridge/Caltech Evidence of brain and beating heart 39 Nature 2023 Embryo models or synthetic embryos (no ethical problems), Day 14, 120 cells in the right combination, 1% efficiency, To treat infertility 40