Spectroscopy 1st 3 Lec Mid PDF
Document Details
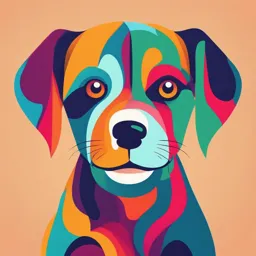
Uploaded by DynamicChrysoprase5522
Tags
Summary
This document provides an introduction to spectroscopy, covering electromagnetic radiation, its characteristics, and interactions with matter. It also details UV-Visible spectrophotometry, electronic transitions, and spectra-structure correlations.
Full Transcript
# Spectroscopy ## Introduction to Spectroscopy Spectroscopy is any procedure that uses the interaction of electromagnetic radiation (EMR) with matter to identify and/or to estimate an analyte. * **Quantitative analysis**: Analytes are generally measured by their absorbance of EMR.  * **Q...
# Spectroscopy ## Introduction to Spectroscopy Spectroscopy is any procedure that uses the interaction of electromagnetic radiation (EMR) with matter to identify and/or to estimate an analyte. * **Quantitative analysis**: Analytes are generally measured by their absorbance of EMR.  * **Qualitative analysis**: Analytes are generally identified by their characteristic EMR absorption spectrum. ## Electromagnetic Radiation (light) EMR can be described in terms of both particles and waves (Dual nature of light). Light waves consist of perpendicular and oscillating electric and magnetic fields: **Diagram:**  ## Characterizing Light Waves Light waves can be characterized by: * **Wavelength (λ):** Distance from one wave peak to the next. * Units: nanometers (nm) or Angstroms (A) * **Frequency (ν):** Number of peaks that pass a given point per second. * Units: Cycles/second or s¯¹ or Hertz (Hz) * **Wavenumber:** Number of waves per centimeter * Units: cm¯¹ * ν= 1/λ * **Velocity of Light (c):** c= ν.λ ## The Particle Nature of Light Electromagnetic radiation consists of discrete packets of energy, called photons. Each photon carries energy, E (Joules). The characteristics of light are related by: * E=hv=hc/λ * h: Planck's constant (= 6.626 x 10-34 J.s) The particle nature can explain phenomena like absorption and emission of light.  > **The greater the energy, the higher the frequency and the shorter the wavelength.** ## Classifying Electromagnetic Radiation According to λ, EMR is classified into: | Type | Frequency (ν) | Wavelength (λ) | | :--------------------------- | :-------------------- | :-------------------- | | Cosmic Rays | 1019 Hz | 10-6 nm | | γ-rays | 1017 Hz | 10-4 nm | | X-rays | 1015 Hz | 10-1 nm | | Ultraviolet light | 1015 Hz | 0.4 μm | | Visible Light | 1014 Hz | 0.8 μm | | Infrared Radiation | 1013 Hz | 102 μm | | Microwaves | 1010 Hz | 106 μm | | Radio Waves | 105 Hz | 1010 μm | | Nuclear Magnetic Resonance | 105 Hz | 1010 μm | **EMR may be visible or invisible:** * **Visible radiations:** form only a small part of the complete EMR. It extends from 400-800 nm. It is subdivided into different colors according to their wavelength (i.e. it is polychromatic). Each definite color is described as monochromatic light. * **Invisible radiations:** which are Ultraviolet (U.V.) and Infrared (I.R.)., ...etc. ## Interaction of a Substance with EMR Absorption of radiant energy by a substance molecule may occur in three ways: * **1. Raising Electrons to a Higher Energy Level (Transitional Energy)** * When molecule absorbs visible and U.V. regions * **2. Raising the Vibration of the Nuclei (Vibrational Energy)** * When a molecule absorbs I.R regions * **3. Increasing Rotation of the Molecule Around the Axis (Rotational Energy)** * When a molecule absorbs F.I.R regions **Diagram:**  When a molecule interacts with radiant energy in the visible and U.V regions, gaining of energy by the molecule leads to displacement of an outer electron (valence electron). The molecule is then said to be excited because the electron undergoes a transition from the original energy level or ground state (E ) to an excited state (E ). ## Molecular Processes and Absorption <start_of_image> Energy (kJ/mol): 1.2 x 108, 1.2 x 107, 12000, 310, 150, 0.12, 0.0012 | Molecular Process | Change in Nuclear Configuration | Ejection of Inner Shell Electrons | Electronic Excitation | Vibration | Rotation | Change in Spin of Electrons or Protons | | :----------------------------------------------------------------------------------------------------------------- | :-------------------------------------------------- | :----------------------------------- | :----------------------- | :-------- | :-------- | :------------------------------------------ | | **Bond breaking and ionization | *Change in Nuclear Configuration* | *Ejection of Inner Shell Electrons* | *Electronic Excitation* | *Vibration* | *Rotation* | *Change in Spin of Electrons or Protons* | | **Molecular processes that occur when light is absorbed in each region:** | **Change in nuclear configuration** | **Ejection of inner shell electrons** | **Electronic excitation** | **Vibration** | **Rotation** | **Change in the spin of electrons or protons** | **Diagram:**  ## Ultraviolet-Visible Spectrophotometry **What happens when a molecule absorbs UV-Visible radiations?** * When a molecule absorbs light having sufficient energy (e.g., UV-Vis radiation) to cause an electronic transition, additional vibration and rotation transitions also occur. * A molecule can absorb one photon of just the right energy to cause the following simultaneous changes: **Diagram:**  1. A transition from the ground electronic state, E to the E or E excited electronic state. 2. A change in vibrational energy from the ground vibrational state E to an excited vibrational state of E 3. A transition from one rotational state E1 to a different rotational state of E1. 4. All the above transitions are quantized which means that they require a specific amount of energy. 5. Thus, total energy absorbed = Eelec + Evib + Erot ΔEelec >> ΔEvib >> ΔErot * The relative energies of translational, vibrational and rotational are roughly in the order of 10000:100:1 **Diagram:**  ## Types of Electronic Transitions The outer electrons in an organic molecule may occupy one of three different energy levels: 1. **Sigma (σ) electrons:** These are bonding electrons, possessing the lowest energy level (most stable). 2. **Pi (π) electrons:** These are bonding electrons with higher energy than sigma electrons. 3. **Non-bonding (n) electrons:** These are of atomic orbital of heteroatoms (N, O, S or X) that do not participate in bonding; they usually occupy the highest level of the ground state. **Diagram:**  In an excited state, σ electrons occupy an antibonding energy level, denoted as σ and the transition is termed σ-σ* transition. π-electrons occupy the antibonding π* level, while n electrons occupy either π* or σ*. ## Spectra-Structure Correlation The absorbance of EMR in the U.V-VIS regions depends on the structure of the organic molecule. **Chromophores:** (Chrom=color, phore=carrier) * Chromophores are functional groups responsible for electronic absorption.  * They have unsaturated bonds such as: C=C, -C=O, -N=N and -C=N (etc. π electrons). **Auxochromes:** * Auxochromes are functional groups that cannot confer colors on substances but have the ability to increase the coloring power of chromophores. * They do not absorb radiations longer than 200 nm, but when attached to a given chromophore, they cause a shift to a longer wavelength, increasing absorption intensity. * Examples: -OH, -NH2. ## Absorption Spectrum **Diagram:**  * An absorption spectrum is characteristic of a substance. * The wavelength at which maximum absorption (λmax) is recorded is used to trace the substance strength and enhance the sensitivity and minimize error. > **Why do we carry out measurements at λmax?** ## σ-Absorption * Compounds containing only σ-electrons are the saturated hydrocarbons which absorb <170nm (i.e. in the far UV). * They are transparent in the near UV (200-300nm), making them ideal solvents to study other compounds in this region. ## n-electron Absorption * Saturated compounds containing heteroatoms or halogens show no absorption in the near UV. * Examples: Methanol at 177 nm, triethylamine at 199 nm, chloroform at 173 nm. * Alcohols and ethers absorb at shorter wavelengths than 185 nm; they act as common solvents at >200 nm. ## Solvent Cut-off * Compounds containing only σ-electrons are are saturated hydrocarbons , which absorb <170nm (i.e., in the far UV). They are transparent in the near UV (200-300nm), making them ideal solvents to study other compounds in this region. * However, their intense absorption usually extends to the edge of the near UV, producing end absorption (cut-off wavelength) in the region of 200-220 nm. **Diagram:**  ## Solvents for UV (showing high energy cutoffs) | Solvent | Cut-off λ | Solvent | Cut-off λ | | :--------- | :-------- | :----------- | :-------- | | Water | 205nm | CCl4 | 265 nm | | Ethanol | 210 nm | Chloroform | 245 nm | | Methanol | 210 nm | THF | 220 nm | | Ether | 210 nm | CH2Cl2 | 235 nm | | Acetone | 300 nm | Dioxane | 220 nm | | Benzene | 280 nm | | | > **Thus, quantitative measurements should be done after the solvent cut-off.** ## Some Important Terms 1. **Bathochromic shift (or red shift):** This is the shift of λmax to a longer wavelength due to substitution with certain functional groups (e.g., -OH and -NH2) and conjugation. 2. **Hypsochromic shift (or blue shift):** This is the shift of λmax to a shorter wavelength due to removal of conjugation. 3. **Hyperchromic effect (or shift):** This effect involves an increase in the intensity of absorption. It is usually brought about by the introduction of an auxochrome. 4. **Hypochromic effect (or shift):** This effect involves a decrease in the intensity of absorption. **Diagram:**  ## Factors Affecting Absorption Spectrum | Factor | Effect | | :---------------------------------------------- | :----------------------------------------------------------------------------- | | **Intensity** | Increase in intensity - increase in absorbance | | **Maximum wavelength** | Affects the color of the absorbed light | | **Effect of pH on absorption spectra** | The spectra of compounds containing acidic (phenolic-OH) or basic (-NH2) groups are dependent on the pH of the medium. | | **Effect of solvent on absorption spectra** | Solvents can affect the position of λmax due to their effect on the energy of transition. | | **Effect of dilution** | Dilution can affect the ionized and unionized forms of a compound. | | **Effect of conjugation** | Extending conjugation leads to a red shift. | | **Effect of temperature** | Changes in temperature may shift ionic equilibrium. | ## Effect of pH on Absorption Spectra The spectra of compounds containing acidic (phenolic-OH ) or basic (-NH2) groups are dependent on the pH of the medium. ### 1. Phenol The U.V spectrum of phenol in an acid medium where the benzenoid form is the predominant species is completely different from its spectrum in alkaline medium, where the predominant species is the corresponding phenate anion. The spectrum in alkaline medium exhibits a bathochromic shift (red shift). **Diagram:**  * On running the U.V spectrum of a known concentration of phenol as a function of pH (at different pH), the spectrum will be shifted to different λmax by changing the pH. However, all spectra will intersect at certain λ, which is known as the isosbestic point. **Diagram:**  * At the isosbestic point, the same absorbance is given for the same concentration at different pH - meaning absorbance is not pH dependent, but concentration dependent. * Analytical measurements of pH-sensitive compounds are done at the isosbestic point. ## Effect of Dilution For example, in the case of weak acids (benzoic acid), the ionized and unionized forms have different λmax. Upon dilution, the ionized form increases, shifting λmax.  > **For measurement, dilution must be carried out with an acid or a base.** ## Effect of Solvent on Absorption Spectra The same concentration of a substance may have a different UV-VIS absorption spectrum. **Diagram:**  * **Dipole-Dipole Interaction (solvent π electrons with substance)** * The solvents may have a strong effect on the position of λmax due to their effect on the energy of transition ## Effect of Conjugation Generally, extending conjugation leads to a red shift. **Diagram:**  ## Effect of Temperature Changes in temperature may shift ionic equilibrium. > **Temperature should be the same for all measurements** ## Laws of Light Absorbance When monochromatic light having intensity (I) is allowed to pass through absorbing medium, some is absorbed (I), reflected (Ir), transmitted (I), refracted (I ), and scattered (I ). The intensity or radiant power of EMR before, I, and after, I, interaction with matter can be related by two quantities: transmittance (T ) and absorbance (A ): **Diagram:**  **To minimize refraction, reflection and scattering loss:** * Scattering loss (I ) is zero for clear solutions. * I and I are canceled by means of a **control cuvette (Blank)** containing the solvent that the substance is dissolved in. ### 1. Transmittance Transmittance is the fraction of light that reaches a detector after passing through a sample. * T = I/I * 0 < T < 1 ### 2. Absorbance Absorbance is defined as:  * A = -log T * A = -log I/I * A =log (I/I) **Diagram:**  ## Beer-Lambert Law Absorbance is directly proportional to: 1. **Concentration (c):** of absorbing species in the sample (A ¤ c) 2. **Path Length (b):** of light through the sample (A ¤ b) > **Beer's Law**: When a monochromatic light enters an absorbing medium, its intensity of transmitted light is decreased exponentially with the increase of the concentration of the absorbing medium, when (b) is constant. log 1/14 (А) ¤ C or A = KC **Diagram:**  > **Lambert's Law**: When a monochromatic light enters an absorbing medium, its intensity is decreased exponentially with the increase of thickness of the absorbing medium (i.e. solution), (b) at constant concentration, C.  log 1/1 (Α) ¤ b or A = Kb **Diagram:**  ### Beer-Lambert Equation Combining Beer's and Lambert's Law, we get the Beer-Lambert Equation: A = abc  * A = log I/I₁ = absorbance * a: refers to absorptivity - the absorbance when the thickness of the solution is unity (1 cm ) and concentration is unity.  * ε: refers to the **molar absorptivity** or **epsilon**. This is the value of a when concentration is in Molarity M (mol/L). The units of *ε* are L mol-1 cm-1. * A (1% - 1cm) : This refers to the absorbance of a 1% solution when the path length is 1 cm. **Diagram:**  * Both *ε* and A (1%, 1cm) are characteristic for each substance at each wavelength and are used for qualitative purposes. * Absorptivity (a) can be calculated from the slope of the curve produced on plotting (A) as a function of (C) at fixed (b). When (b) is 1 cm: * A = ac or a = A/C * A/C =a = slope of the curve * This can be used to determine an unknown concentration using a standard curve. **Diagram:**  ## Instrumentation (Spectrophotometers) **Instruments for measuring the absorption of U.V. or visible radiation are made up of the following components:** 1. **Source of UV and Visible Radiation** 2. **Wavelength selector (that permits the employment of restricted wavelength regions)** 3. **Sample containers** 4. **Detector** 5. **Signal processor and readout** ### 1. Source of Radiation * The source of radiation should deliver highly intense, continuous, constant radiation. * **UV sources:** * Hydrogen discharge or deuterium lamp - made of quartz because glass absorbs radiation of wavelengths less than 350 nm. The electrical excitation of deuterium or hydrogen at low pressure produces a continuous UV spectrum in the range of 160-350 nm. * **Visible sources:** * Tungsten filament lamp - commonly employed as a source of visible light. The electrical heating of a tungsten filament produces continuous radiation in the wavelength range of 350-800 nm. ### 2. Wavelength Selector (Dispersion devices) In quantitative analysis, a monochromatic radiation, or at least a band of few restricted wavelengths including the analytical wavelength, is allowed to pass through a sample to enhance sample sensitivity and ensure the obedience to the Beer Lambert Law. There are two main types of wavelength selectors: 1. **Filters:** * **Colored filters:** Function by light absorption, but the obtained radiation is not monochromatic. It has a wide band of 30-50 nm. * **Types:** * **Gelatin filters:** Layer of gelatin impregnated with organic dyes. * **Liquid filters:** Solution of absorbing compound in a suitable dissolving solvent. * **Glass filters:** Sheets of glass colored with metal oxides or pigments. * **Interference filters:** Function by light interference and consist of multiple layers of low and high refractive materials. They provide constructive or destructive interference of light through different materials, taking advantage of the refraction of light. * **Interference principle:** When a crest meets another crest, the resulting wave amplitude is increased; when a crest meets a trough, they cancel each other.  * **Advantages:** Much better filtering characteristics than colored filters (narrower band of light obtained). Since light is reflected rather than absorbed, there is less heating of the filter. They also have a longer lifespan. * **Disadvantage:** Higher cost **Diagram:**  2. **Monochromators (convert polychromatic light to monochromatic)** * **Monochromator components:** * Entrance slit * Collimating lens * Dispersing device (prism or grating) * Focusing lens * Exit slit * **Working principle:** Polychromatic radiation enters the monochromator through the entrance slit, is collimated, and then strikes the dispersing element. The beam is split into its component wavelengths by the grating or prism, and wavelengths pass through the exit slit. **Diagram:**  * **Prism monochromator:** Uses a prism that disperses light by refraction (the change in direction of a wave due to a change in its speed). Dispersion occurs due to variation of the refractive index with wavelength. **Diagram:**  * **Grating monochromator:** Disperses light by diffraction - occurring when a wave encounters an obstacle, which is described as the spreading out of waves. The grating separates the incoming polychromatic beam into its constituent wavelength components (i.e., it is dispersive). **Diagram:**  * **Gratings:** Consist of a large number of grooves on glass blanks coated with aluminum. * **Advantages:** More commonly used due to less expensive fabrication costs * **Diffraction principle:** Based on the incident light to the grating and the spacing between grooves. The direction of the beams depends on the wavelength of the light, meaning the grating acts as the dispersive element. ### 3. Sample Cell Compartment (cuvette) Care must be taken when considering a sample container (commonly called a cuvette) to avoid unwanted absorption in the range of interest.  * **Glass cuvettes** are perfect for the visible range, but they absorb UV radiation. * **Quartz cuvettes** can be used for both UV and visible ranges, but they are more expensive. > **They should be of high quality with an exact known internal pathlength.** **Diagram:**  ### 4. Detector * Detectors convert radiation to electrical signals. * Detectors used in UV/VIS regions are **photoelectric detectors** * **Requirements:** 1. Give a linear response to radiation 2. Give a rapid response 3. Response to low radiation levels Photoelectric detectors can be further divided into: 1. **Photovoltiac or Barrier Layer Cells:**  * This is a method of generating electrical power by converting radiation into electricity using semiconductors. * The cell consists of a layer of copper or iron (anode electrode), on which sits a layer of semiconducting material (e.g., selenium). * The outer surface of the semiconductor is coated with a silver cathode electrode. * The whole structure is protected by a transparent envelope.  * When radiation hits the semiconductor, electrons move towards the silver layer, passing through a barrier layer and collected by the silver electrode. * When cathode and anode are connected, a current will pass. * **Advantages:** Durable and cheap * **Disadvantages:** Used in the visible region only and suffers fatigue due to prolonged exposure to light. **Diagram:**  2. **Phototubes:** * Phototubes consist of a glass envelope with a vacuum inside. * They also have a light-sensitive cathode coated with photoemissive material (e.g., sodium or potassium), and an anode.  * Light strikes the cathode, emitting negative electrons and attracting them toward a positive anode. * The current is proportional to the intensity of light falling on the cathode.  **Diagram:**  3. **Photomultiplier Tubes (PMTs):**  * Consist of a photoemissive cathode and several anodes at gradually increasing potentials.  * A photon of radiation strikes the cathode, causing electrons to be emitted. * Electrons are accelerated toward the first anode, striking it and emitting more electrons - this process is repeated through several anodes. * Electrons are collected at the last anode. * PMTs are used to detect very weak light intensities (most sensitive). **Diagram:**  ### 5. Meter The electrical signal is fed to a sensitive galvanometer attached to a scale to give absorbance or transmittance readings. ## Spectrometers ### 1. Single Beam Spectrometer * A single beam spectrometer consists of a light source, a monochromator, a sample cell, and a detector. * The light source is a UV or visible light source, such as a deuteriuim or tungsten lamp.  * The monochromator separates the light into single wavelengths. * The sample cell contains the sample being measured. * The detector measures the intensity of light passing through the sample cell. * The signal is amplified and processed by the meter. **Diagram:**  ### 2. Double Beam Spectrometer * A double beam spectrometer splits the beam of radiation and passes one beam through the sample and the other through a reference (a cuvette containing the solvent alone). * Any differences in the intensity of the two beams are measured, and the instrument often compares the absorbance to a reference standard. * This eliminates error due to fluctuations in the light source and provides a more stable and consistent measurement. **Advantages:** * Eliminates errors due to drift in source intensity. * Allows for continuous scanning of absorbing spectra. **Diagram:**  ## Colorimetry Colorimetry is an instrumental method of analysis that depends on measuring the absorption of the visible light by the system to be determined. An analyte can be analyzed colorimetrically if it meets the following criteria: 1. **The compound is self-colored.** 2. **The compound must react with a reagent (chromogen) to produce a colored product.** 3. **The compound must be converted to a derivative that reacts with a reagent to produce a colored product.** 4. **It should be intensely colored.** 5. **It should be soluble in the solvent used.** 6. **The color formation reaction should have definite stoichiometry.** 7. **The colored solution should be stable for a reasonable period of time.** 8. **The colored compound should obey Beer-Lambert Law.** **Requirements for an ideal chromogen:** 1. **Colorless or has no absorbance at the λmax of the colored compound.** 2. **Selective** 3. **It should produce one single product.** 4. **The reaction has definite stoichiometry.** 5. **Color development must be rapid.** ## Quantitative Measurement of Electromagnetic Radiation Absorption * **Spectrophotometry:** using visual or instrumental methods ### 1. Visual Methods These are only used with colored samples. * **Standard series method (using polychromatic light):** * Compare a colored sample with a standard series of colors (freshly prepared or a permanent solution). * Matching Nessler tubes are often used for freshly prepared standards. * For permanent methods, a colored disc, Lovibond comparator or sealed ampoules are used.  **Diagram:**  * **Variable depth or balancing method:** * Compare a sample with a solution of known concentration (standard solution) until the light intensity emerging from the sample and the standard are the same. * The concentration of the unknown sample can be calculated by adjusting the path length of light through the sample cell. * Two main instruments are used: * **Hehner tube:** Designed to withdraw concentrated solutions, matching the color of the sample with the color of a standard solution. * **Duboscq-type colorimeter:** Two tubes can be adjusted in depth. **Diagram:**   ### 2. Instrumental Methods * **Instruments:** Spectrometer (UV), spectrophotometer (UV/VIS), colorimeter (VIS) * The instruments are largely similar but differ in the materials used.  ### Spectrophotometers and Colorimeters **Instruments for measuring the absorption of U.V. or visible radiation are made up of the following components:** 1. **Source of UV and Visible Radiation** 2. **Wavelength selector (that permits the employment of restricted wavelength regions)** 3. **Sample containers** 4. **Detector** 5. **Signal processor and readout** **Diagram:** 