Conventional Energy Sources PDF
Document Details
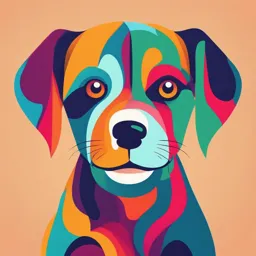
Uploaded by VigilantOnomatopoeia
Al-Balqa Applied University
Tags
Summary
This document provides an introduction to the concept of energy, covering various forms, classifications, and units of measurement. It explores the principles of energy conservation and different types of energy, including kinetic and potential energy, along with energy efficiency.
Full Transcript
Conventional Energy Sources COURSE: MEE6250 Chapter-1 Energy Basics: Energy classification, resources, and utilization Introduction Energy is a property, when changed, it will produce heat and/ or work. Major advances in human civilizations are measured by useful energy consu...
Conventional Energy Sources COURSE: MEE6250 Chapter-1 Energy Basics: Energy classification, resources, and utilization Introduction Energy is a property, when changed, it will produce heat and/ or work. Major advances in human civilizations are measured by useful energy consumption. Sometimes, energy consumption is not always useful. For example: flaring of gas from oil wells and from refinery flares. This is an energy consuming activity that is wasteful to the economyand harmful to the environment. The 3-E's Energy Economy Environment Primary Energy Primary energy in the form that it is first accounted for in a statistical energy balance before any transformation to secondary or tertiary forms of energy. *For example: coal can be converted to synthetic gas, which can be converted to electricity; in this example, coal is primary energy, synthetic gas is secondary energy, and electricity is tertiary energy. See Primary energy production and Primary energy consumption. A quantity that any kind of energy can be expressed in its units. *For example: Jouls (J), kilojouls (kJ), Megajouls (MJ), Gigajouls (GJ),British Thermal Unit (Btu), Million Btu (MBtu), Ton of Oil Equivalent (T.O.E)............................. used in countries that mostly use oil Barrel of Oil Equivalent (B.O.E)…. " " " "" " " Ton of Coal Equivalent (T.C. E)….. " " " "" " coal *For example: Jordan consumed 7.35 Million T.O.E in the year 2012. All units are transferrable to each other. * For example: 1 B.O.E = 5.8 x 106 Btu 1 T.O.E = 7 B.O.E See App. B for more conversion factors. Energy and Power Power = Energy / time = dE / dt dE = P dt E = ʃ P dt = P ʃ dt Usually, in energy and power problems, the amount that is fixed is E and t may be reduced to get higher P. An important feature of energy and power is that: 1- Energy is cumulative but power is not. 2- Energy can be stored but power cannot. Energy Efficiency Define energy efficiency for a system of energy converters as η = Energy Output / Energy Input such as the thermal efficiency that you know for a heat enginewhich is: η = Work Output / Heat Input The difference is in that the numerator hear is work. Units of Energy In SI system In British system E ≡ Joul = N.m E ≡ Btu, ft.lbf P ≡ Btu / hr , ft.lbf / s1 hp = 550 ft.lbf / s 1 kW = 1.341 hp Now if 1 W = J/ sHence 1 J = W.s 1 kJ = 1 kw.s = 1 kW-hr / 3600 1 kW-hr = 3600 kJ; a famous unit mostly use in electricity generation. Example: A 100 W bulb is switched ON for 8 hours. If the price of electricity is 50 fils / kW-hr, what is the cost of energy consumed during that period? Solution: Since the price of electricity is given in the form of fils/kW-hr, calculate the price of energy consumed in the given time period: E = P. t = 100 x 8 x 3600 = 2.88 x 106 J = 2.88 x 103 kJ = 2.88 x 103/ 3600 = 0.8 kW-hr 0.8 x 50 = 40 fils Energy classification Classification based on energy source: 1- Conventional (Non-Renewable) 2- Non-conventional (Renewable) Classification based on energy form. 1-Kinetic energy 2-Potential energy These various kinetic or potential forms of energy have been obtained from various natural phenomena. According to the law of conservation of energy, each of these forms is converted to another form. The Law of conservation of energy states that energy can neither be created nor destroyed but may be transferred from one form to another. For example, when we burn wood or fuel like gasoline or natural gas, it changes chemical energy to thermal or mechanical energy. Kinetic energy Kinetic energy is a type of energy that is obtained by the motion of an object. For example, walking, falling, flying, and throwing. It is also defined as the work required to accelerate a body of a given mass from rest to its stated velocity. After gaining this energy, the body maintains a fixed kinetic energy unless its speed changes. It can be calculated by the equation, KE = (mv2)/2 where m = mass of the object v = velocity of the object. Electrical Energy To understand where electrical energy comes from, we must start by looking at the atom, which is the building block of all matter. Each atom has a nucleus in its center. The nucleus consists of positively charged particles called protons, and particles called neutrons that have no charge. Electrons, which are negatively charged particles, orbit around the nucleus. These orbits are called shells. Each shell can contain only a certain number of electrons: the first shell can hold 2 electrons, the second shell can hold 8 electrons, the third shell can hold up to 18, and so on. Electricity and electric energy are commonly thought to be one and the same; however, this is not true. Electric energy comes from the transfer of energy resulting from the movement of electrons between atoms, which is known as work. This work generates electric energy, which is measured in Joules. This electric energy can be converted into different forms. When the electron flow of electrical energy is channeled through a conductor, like a wire, it becomes electricity. Electricity is measured in Watts. Therefore, while all electricity is electrical energy, not all electrical energy is electricity. Electricity is a type of energy that can build up in one place, or flow from one place to another. When electricity gathers in one place it is called static electricity. Electricity that moves from one place to another is called current electricity. Static electricity often happens when you rub things together. For example, when you rub a balloon on your head it causes opposite static charges to build up both on your hair and the balloon. When you pull the balloon slowly away from your head, these two opposite static charges attract one another making your hair stand up. Current electricity is the electricity that powers our homes and electrical devices. Electric energy can be kinetic or potential. Static electricity is a form of electrical potential energy. If sufficient charge builds up, the electrical energy may be discharged to form a spark, which has electrical kinetic energy. Another example is a phone battery that holds electric energy to use later. That held energy can become kinetic energy when you are ready to turn your phone on and use it. Conductors are made of atoms who’s outer, or valence, electrons have relatively weak bonds to their nuclei, as shown in this fanciful image of a copper atom. When a bunch of metal atoms are together, they gladly share their outer electrons with each other, creating a "swarm" of electrons not associated with a particular nucleus. A very small electric force can make the electron swarm move. Copper, gold, silver, and aluminum are good conductors. So is saltwater. Insulators are materials whose outer electrons are tightly bound to their nuclei. Modest electric forces are not able to pull these electrons free. When an electric force is applied, the electron clouds around the atom stretch and deform in response to the force, but the electrons do not depart. Glass, plastic, stone, and air are insulators. Even for insulators, though, electric force can always be turned up high enough to rip electrons away—this is called breakdown. That's what happens to air molecules when you see a spark. Semiconductor materials fall between insulators and conductors. They usually act like insulators, but we can make them act like conductors under certain circumstances. The most well-known semiconductor material is Silicon (atomic number \[14\]). Our ability to finely control the insulating and conducting properties of silicon allows us to create modern marvels like computers and mobile phones. The atomic-level details of how semiconductor devices work are governed by the theories of quantum mechanics. Current is reported as the number of charges per unit time passing through a boundary. Electric current is divided into two types: Directional Current (DC) Alternating Current (AC). Directional (Direct) Current A non-varying, unidirectional electric current (Example: Current produced by batteries) Characteristics: Direction of the flow of positive and negative charges does not change with time. Direction of current (direction of flow for positive charges) is constant with time. Potential difference (voltage) between two points of the circuit does not change polarity with time Alternating Current A current which reverses in regularly recurring intervals of time and which has alternately positive and negative values, and occurring a specified number of times per second. (Example: Household electricity produced by generators; Electricity supplied by utilities.) Characteristics: · Direction of the current reverses periodically with time · Voltage (tension) between two points of the circuit changes polarity with time. · In 50 cycle AC, current reverses direction 100 times a second (two times during one cycle) - Ampere (A) Current is the rate of flow of charge. The ampere is the basic unit of electric current. It is that current which produces a specified force between two parallel wires, which are 1 meter apart in a vacuum. - Voltage (V) The volt is the International System of Units (SI) measure of electric potential or electromotive force. A potential of one volt appears across a resistance of one ohm when a current of one ampere flows through that resistance. EXAMPLES: EX1: EX2: P= E/t E= 0.5*5*10*50=1250 J EX3: EX4: Mechanical energy Mechanical energy is the energy of an object due to its position or motion. It is the basis of physics, as everything around us is driven by mechanical energy. From picking up objects to throwing them, mechanical energy can be seen in action every day. For example, an apple falling from a tree has mechanical energy. * Mechanical energy is generally defined as the sum of kinetic energy and potential energy in an object. It is accumulated due to performing some work. In other words, we can describe the energy of an object because of its motion or position, or sometimes both. There are two main types of mechanical energy. 1. Potential Energy: It is the energy stored in an object due to its position. Gravitational potential energy due to Earth’s gravity is a common type of potential energy. It depends on the object’s height from the Earth’s surface. 2. Kinetic Energy: It is the energy possessed by an object due to its motion. The movement of an object is manifested by its speed. An object possessing mechanical energy can do work by applying force. The change in mechanical energy is the work done. *Stored Mechanical Energy Stored mechanical energy is energy stored in objects by the application of a force. Compressed springs and stretched rubber bands are examples of stored mechanical energy. Sound energy Sound energy is the result when a force, either sound or pressure, makes an object or substance vibrate. That energy moves through the substance in waves. Sound waves are sometimes called mechanical waves because sound waves require a physical medium to propagate. Liquids, gases, or solid materials transfer pressure variations, creating mechanical energy in waves. Like all waves, sound waves have peaks and valleys. The peaks are called compressions, while rarefaction is the term used for the lows. The oscillations between compression and rarefaction move through gaseous, liquid, or solid media to produce energy. The number of compression/rarefaction cycles in a given period determines the frequency of a sound wave. Scientists measure sound energy’s intensity and pressure in Pascals and decibels. Sound waves are also sometimes called pressure waves because the pressure of the sound wave moves the particles through which it passes. Sound waves can be measured by wavelength, period, amplitude, and frequency are the four primary parts of a sound wave, regardless of the wave type and the medium through which the sound travels. Wavelength: Imagine a wave traveling along a horizontal axis; in that case, the wavelength is measured as the horizontal distance between two successive and equivalent points on the wave. Thus, in basic terms, a single wavelength is one cycle between the two equal points. Period: A wavelength period is the time it takes a single wavelength to pass a certain point. Generally, a more extended period indicates a lower pitch. Amplitude: We measure sound amplitude (strength or level of sound pressure) by the height of the sound wave. It’s related to the relative volume of the sound. When the wave’s amplitude is significant — as from a loud sound — the wave is high. The reverse is also true; softer sounds produce waves with a smaller amplitude. Lower volume equates to lower decibel (dB) levels; a decibel measures sound intensity. Zero decibels equates to the quietest sounds a human ear can hear. Decibels increase by a factor of six. A normal speaking voice is 60 dB. Frequency: Hertz (Hz) measures a sound wave’s frequency. Hertz measures a sound wave’s cycles per second that pass a set point on the horizontal axis. (Remember, each process has one compression and one rarefaction.) The frequency sound waves are measured in hertz. Therefore, Hertz (Hz) indicates the number of cycles per second that pass a given location. For example, if, while speaking, your diaphragm vibrates at 900 Hz, your diaphragm generates 900 compressions (increased pressure) and 900 rarefactions (decreased pressure). Pitch is a function of how the brain interprets sound frequency. A higher pitch is the result of higher frequency; lower frequency translates as lower pitch. Types of transverse waves include: Vibrations in a guitar string Sports fans standing up and sitting down in a synchronistic wave around a sport stadium Electromagnetic waves, such as light and radio waves Radiation Energy Radiant energy, also known as electromagnetic radiation (EMR), is energy transmitted without the movement of mass. Practically speaking, this is the energy found in electromagnetic waves, also known as light. Light is made of individual particles called photons, each carrying a small "packet" of energy. Because photons are so small, light energy is often measured in electron volts. Examples of radiant energy include the warmth that radiates from a hot stove and the warmth from direct sunlight. This electromagnetic wave can be seen in the following figure. Not all radiant energy is visible (as shown in bellow). Only photons within a very small range of energies can be seen by people, commonly known as visible light. Photons with lower energies are found in microwaves, radio waves, and as infrared radiation (which is felt as heat). Photons with higher energies are found in ultraviolet rays, in x-rays, and in gamma rays. Ways to use collect radiant energy include: Solar power harvests radiant energy carried by the light from our sun by converting it into electricity. Biomass from plants. Plants are able to harness and use light energy in a process called photosynthesis. They absorb radiant energy from sunlight and transform it into useful chemical energy contained in molecules within their cells. Animals can obtain some of this chemical energy as food by eating plants, or by eating animals which eat other animals or plants. The biomass created by plants can also be used in biofuels. Radioisotope thermal generators use radiant energy from a radioactive source to collect heat or even make electricity. Thermal energy Temperature and Pressure Temperature and pressure are measures of the physical state of a substance. They are closely related to the energy contained in the substance. As a result, measurements of temperature and pressure provide a means of determining energy content. Temperature: It is the degree of hotness or coldness measured on a definite scale. Heat is a form of energy; temperature is a measure of its thermal effects. In other words, temperature is a means of determining sensible heat content of the substance. In the Celsius scale the freezing point of water is 0°C and the boiling point of water is 100°C at atmospheric pressure. *To change temperature given in Fahrenheit (°F) to Celsius (°C) Start with (°F); subtract 32; multiply by 5; divide by 9; the answer is (°C) *To change temperature given in Celsius (°C) to Fahrenheit (°F) Start with (°C); multiply by 9; divide by 5; add on 32; the answer is (°F) Pressure: It is the force per unit area applied to the outside of a body. When we heat a gas in a confined space, we create more force, a pressure increase. For example, heating the air inside a balloon will cause the balloon to stretch as the pressure increases. Pressure, therefore, is also indicative of stored energy. Steam at high pressures contains much more energy than at low pressures. Heat: It is a form of energy, a distinct and measurable property of all matter. The quantity of heat depends on the quantity and type of substance involved. *Unit of Heat Calorie is the unit for measuring the quantity of heat. It is the quantity of heat, which can raise the temperature of 1 g of water by 1°C. Specific Heat If the same amount of heat energy is supplied to equal quantities of water and milk, their temperature goes up by different amounts. This property is called the specific heat of a substance and is defined as the quantity of heat required to raise the temperature of 1kg of a substance through 1°C. The specific heat of water is very high as compared to other common substances; it takes a lot of heat to raise the temperature of water. Also, when water is cooled, it gives out a large quantity of heat. Quantity of Heat : The quantity of heat, Q, supplied to a substance to increase its temperature by t°C depends on – mass of the substance (m) – increase in temperature (∆t) – specific heat of the substance (Cp) Calorific Value: Energy content in an organic matter (Calorific Value) can be measured by burning it and measuring the heat released. This is done by placing a sample of known mass in a bomb calorimeter, a device that is completely sealed and insulated to prevent heat loss. A thermometer is placed inside (but it can be read from the outside) and the increase in temperature after the sample is burnt completely is measured. From this data, energy content in the organic matter can be found out. The heating value of fuel is the measure of the heat released during the complete combustion of unit weight of fuel. It is expressed as Gross Calorific Value (GCV) or Net Calorific Value (NCV). The difference between GCV and NCV is the heat of vaporization of the moisture and atomic hydrogen (conversion to water vapour) in the fuel. Typical GCV and NCV for heavy fuel oil are 10,500 kcal/kg and 9,800 kcal/kg. Heat Transfer: Heat will always be transferred from higher temperature to lower temperature independent of the mode. The energy transferred is measured in Joules (kcal or Btu). The rate of energy transfer, more commonly called heat transfer, is measured in Joules/second (kcal/hr or Btu/hr). Heat is transferred by three primary modes: Conduction (Energy transfer in a solid) Convection (Energy transfer in a fluid) Radiation (Does not need a material to travel through Thermal Radiation Thermal radiation is a process in which energy is transferred by electromagnetic waves similar to light waves. These waves may be both visible (light) and invisible. A very common example of thermal radiation is a heating element on a heater. When the heater element is first switched on, the radiation is invisible, but you can feel the warmth it radiates. As the element heats, it will glow orange and some of the radiation is now visible. The hotter the element, the brighter it glows and the more radiant energy it emits. The key processes in the interaction of a substance with thermal radiation are: Absorption the process by which radiation enters a body and becomes heat. Transmission the process by which radiation passes through a body. Reflection the process by which radiation is neither absorbed nor transmitted through the body; rather it bounces off Objects receive thermal radiation when they are struck by electromagnetic waves, thereby agitating the molecules and atoms. More agitation means more energy and a higher temperature. Energy is transferred to one body from another without contact or transporting medium such as air or water. In fact, thermal radiation heat transfer is the only form of heat transfer possible in a vacuum. All bodies emit a certain amount of radiation. The amount depends upon the body's temperature and the nature of its surface. Some bodies only emit a small amount of radiant energy for their temperature, commonly called low emissivity materials (abbreviated low-E). Low-E windows are used to control the heat radiation in and out of buildings. Windows can be designed to reflect, absorb, and transmit different parts of the sun's radiant energy. The condition of a body's surface will determine the amount of thermal radiation that is absorbed, reflected or re-emitted. Surfaces that are black and rough, such as black iron, will absorb and re-emit almost all the energy that strikes them. Polished and smooth surfaces will not absorb, but reflect, a large part of the incoming radiant energy. Typical units of measure for rate of radiant heat transfer Metric (SI) Watt per square meter (W/m2 ). Evaporation The change by which any substance is converted from a liquid state and carried off as vapour. *Example: People are cooled by evaporation of perspiration from the skin and refrigeration is accomplished by evaporating the liquid refrigerant. Evaporation is a cooling process. Condensation The change by which any substance is converted from a gaseous state to liquid state. Thermodynamics laws 1- First law of Thermodynamics It states that energy may be converted from one form to another, but it is never lost from the system. 2- Second Law of Thermodynamics In any conversion of energy from one form to another, some amount of energy will be dis sipated as heat. Thus no energy conversion is 100 % efficient. This principle is used in energy equipment efficiency calculations. Law of Conservation of Matter In any physical or chemical change, matter is neither created nor destroyed, but it may be changed from one form to another. For example, if a sample of coal were burnt in an enclosed chamber, carbon in coal would end up as CO2 in the air inside the chamber; In fact, for every carbon atom there would be one carbon dioxide molecule in the combustion products (each of which has one carbon atom). So the carbon atoms would be conserved, and so would every other atom. Thus, no matter would be lost during this conversion of the coal into heat. This principle is used in energy and material balance calculations Pressure: Gauge pressure is defined relative to the prevailing atmospheric pressure (101.325 kPa at sea level), or as absolute pressure: Absolute Pressure = Gauge Pressure + Prevailing Atmospheric Pressure Units of measure of pressure: *Metric (SI) : kilopascals (kPa) *1 pascal (Pa) = 1 Newton/m2 (N/m2 ) *1 physical atmosphere (atm) = 101325 Pa = 760 mm of mercury (mm Hg) = 14.69 lb-force/in2 (psi) *1 technical atmosphere (ata) = 1 kilogram-force/cm2 (kg/cm2 )= 9.806650 × 104 Pa See this video: https://study.com/academy/lesson/what-is-thermal-energy-definition-examples.html Potential energy In physics or chemistry, potential energy is the energy held by an object due to its position relative to other objects. Gravitational, elastic, chemical, and electric potential energies are the common types of potential energy that can be measured by unit (SI) joule. It is stored energy that can be measured by the amount of work done by the object. For example, books on a table, water in a lake. Gravitational potential energy can be calculated by the equation, PE = m.g.h where m = mass of the object g = acceleration due to gravity h = height. To understand potential energy, we need to understand energy in general. Energy is defined as the ability of an object to do work on another object, which means moving that object some distance. Energy comes in many forms; some we can see (like mechanical) and others we can't (like chemical or electrical). Regardless of what form it's in, energy exists in one of two states: kinetic or potential. An object has kinetic energy when it's in motion. The amount of kinetic energy an object has depends on its mass and speed. Potential energy, on the other hand, is stored energy when an object is at rest. It describes how much work the object could do if set into motion. It's an energy we can generally control. When an object is set into motion, its potential energy transforms into kinetic energy. Potential energy exists in various forms: Chemical energy Electrical potential energy Electric potential energy is the energy that is needed to move a charge against an electric field. You need more energy to move a charge further in the electric field, but also more energy to move it through a stronger electric field. Just like mass in a gravitational field has gravitational potential energy, charges in an electric field have an electric potential energy. A charge's electric potential energy describes how much stored energy it has, when set into motion by an electrostatic force, that energy can become kinetic, and the charge can work. Like a bowling ball sitting at the top of a tower, a positive charge in close proximity to another positive charge has a high potential energy; left free to move, the charge would be repelled away from the like charge. A positive test charge placed near a negative charge would have low potential energy, analogous to the bowling ball on the ground. To instill anything with potential energy, we have to do work by moving it over a distance. In the case of the bowling ball, the work comes from carrying it up 163 floors, against the field of gravity. Similarly, work must be done to push a positive charge against the arrows of an electric field (either towards another positive charge, or away from a negative charge). The further up the field the charge goes, the more work you have to do. Likewise, if you try to pull a negative charge away from a positive charge--against an electric field--you have to do work. For any charge located in an electric field its electric potential energy depends on the type (positive or negative), amount of charge, and its position in the field. Electric potential energy is measured in units of joules (J). So, the electric potential, or voltage, is the difference in potential energy per unit charge between two locations in an electric field. When we talked about electric field, we chose a location and then asked what the electric force would do to an imaginary positively charged particle if we put one there. To find the electrical potential at a chosen spot, we ask how much the electrical potential energy of an imaginary positively charged particle would change if we moved it there. Just like when we talked about electric field, we don’t actually have to place a positively charged particle at our chosen spot to know how much electrical potential energy it would have. Electric potential builds upon electric potential energy to help define how much energy is stored in electric fields. It's another concept which helps us model the behavior of electric fields. Electric potential is not the same thing as electric potential energy! At any point in an electric field the electric potential is the amount of electric potential energy divided by the amount of charge at that point. It takes the charge quantity out of the equation and leaves us with an idea of how much potential energy specific areas of the electric field may provide. Electric potential comes in units of joules per coulomb (J/C), which we define as a volt (V). In any electric field there are two points of electric potential that are of significant interest to us. There's a point of high potential, where a positive charge would have the highest possible potential energy, and there's a point of low potential, where a charge would have the lowest possible potential energy. One of the most common terms we discuss in evaluating electricity is voltage. A voltage is the difference in potential between two points in an electric field. Voltage gives us an idea of just how much pushing force an electric field has. Nuclear energy It is the form of energy released due to the splitting of atomic nuclei. We used nuclear fission reactions for the production of usable nuclear energy. The nuclear fusion reaction that occurs in the interior of the sun is the main source of energy for our earth. When a heavy nucleus like 92U235 is bombarded by a neutron, the total mass of nuclei is not equal to the sum of the masses of the heavy nucleus and the neutron. Similarly, when two light nuclei like 1H2 fused together to form a heavier and stable nucleus, the mass of the product is not equal to the sum of masses of the initial lighter nuclei. This shows that there is always a difference in mass, called mass defect, in a nuclear reaction. The difference in mass is converted into energy which is known as nuclear energy. Nuclear Fission When a heavy unstable nucleus of an atom breaks up into two or more lighter nuclei, a large amount of energy is released. This phenomenon is called nuclear fission. For example, The energy is released in the process due to the difference in mass of the initial nucleus and its products. The initial mass is greater than the sum of masses of the products and the difference in mass(Δm) is converted into energy according to Einstein's mass energy relation E = Δm.c2 A chain reaction is a self-propagating process in which number of neutrons goes on multiplying rapidly almost in geometrical progression during fission till all the fissionable material is disintegrated. There are two types of chain reaction. 1. Controlled chain reaction: The number of neutrons and energy is controlled to a desired level in this reaction. This principle is used in nuclear reactors. 2. Uncontrolled chain reaction: The neutrons are allowed to multiply indefinitely in this reaction. This principle is used in atom bomb. Nuclear fusion Nuclear fusion is a process in which two or more lighter nuclei combine or fuse together to form a heavier and stable nuclide. In nuclear fusion, the sum of mass of lighter nuclei is always greater than the mass of heavy nucleus. The difference in mass (Δm) resulting in the process is converted into energy according to Einstein's mass energy relation E = Δmc2 which is released during fusion reaction. For example, Fusion reactions constitute the fundamental energy source of stars, including the Sun. Hydrogen "burning" initiates the fusion energy source of stars and leads to the formation of helium. Generation of fusion energy for practical use also relies on fusion reactions between the lightest elements that burn to form helium. The difference between the masses before and after the reaction corresponds to the reaction energy, according to the mass-energy relation E = Δm.c2. If initial particles A and B interact to produce final particles C and D, the reaction energy Q is defined as: Gravitational potential energy gravitational potential energy is the energy stored in the object because of its height above the ground within the gravitational field. We are all experiencing the force of gravity within the gravitational field of the earth. If the work is done on the object against the gravitational force, the object will gain gravitational potential energy. For example, when an object is lifted to a height ‘h’, work is done against the gravitational force which is pulling it down. This work done on the object is stored as potential energy. If this lifted object is released from height ‘h’ then its potential energy gets converted into the energy of motion or kinetic energy. The amount of gravitational potential energy an object has depends on the mass of the object and height of the object above ground. Objects that are at large height above the ground have more potential energy. Similarly, objects that are at small height above the ground have less potential energy. Objects that have more mass have more potential energy. Similarly, objects that have less mass have less potential energy. Let's take for example, object C has more potential energy than object B because object C has more mass than object B. Similarly, object A has less potential energy than objects C because object A is present at less height. Energy Conversion Energy Energy Conversion Energy is defined as "the ability to do work." In this sense, examples of work include moving something, lifting something, warming something, or lighting something. The following is an example of the transformation of different types of energy into heat and power. For example, Heat engine is a type of engine, (like the motor in a car) that produces macroscopic motion from heat. * Gasoline (chemical) is put into our cars, and with the help of electrical energy from a battery, provides mechanical (kinetic) energy. Another example, A Steam Turbine is a mechanical device that extracts thermal energy from pressurized steam and transforms it into mechanical work. The principle of the conservation of energy states that energy cannot be created or destroyed. It can only be converted into another type of energy, a process known as energy conversion. There are many ways energy can be converted from one form to another. Often, we use machinery or technology to change one type of energy into another, but it can happen naturally as well. Grades of Energy High-Grade Energy: Electrical and chemical energy are high-grade energy, because the energy is concentrated in a small space. Even a small amount of electrical and chemical energy can do a great amount of work. The molecules or particles that store these forms of energy are highly ordered and compact and thus considered as high-grade energy. High-grade energy like electricity is better used for high grade applications like melting of metals rather than simply heating of water. Low-Grade Energy Heat is a low-grade energy. Heat can still be used to do work (example of a heater boiling water), but it rapidly dissipates. The molecules in which this kind of energy is stored (air and water molecules), are more randomly distributed than the molecules of carbon in a coal. This disordered state of the molecules and the dissipated energy are classified as low-grade energy. Energy Sources Various types of energy sources are there that can be divided into different parts. The sun is not only the source of energy. Geothermal energy, nuclear energy, biomass, wind energy, and petrol are some of the other important sources from which energy can be extracted. Based on the characteristic of sustainability, energy is classified into two main types of energy sources. Energy can be extracted from both renewable and non-renewable sources. Conventional and non-conventional sources of energy are two other types of energy sources that play an important role in the consumption and usage of energy. The two most important sources of energy are renewable and non-renewable energy. Natural resources of energy such as geothermal, solar, wind, tidal, hydropower, and biomass energies are renewable energies that are sustainable in nature. These energy sources are safe for the environment. Two types of natural sources of energy As time moves forward, the natural sources of energy have been changed accordingly. Good sources of energy may consist of some significant characteristics among which easy transportation, high economic value, and less polluting attributes are important (Malav et al. 2020). Natural sources of energy are divided into two types, conventional and non- conventional energy sources. Conventional energy sources are being used for a long time and these are well-known and introduced sources of energy. Non-conventional sources of energy are introduced just a few years back and are developing day by day. Petroleum, coal, and natural gas are examples of conventional sources. Biogas, solar and geothermal are examples of non-conventional energy sources. Energy Reserves Until so far that the energy requirement is going to increase in the future and also that the U.S. and the rest of the world will depend to some extent on fossil fuels. These fossil fuels are non-renewable fuels with a finite lifetime. So, the question is: Will we have enough supply for future energy requirements? The answer to this question depends on the quantity of fossil fuels we have in the ground. Energy sources that have been discovered but not produced cannot be easily measured. Trapped several feet below the surface, they cannot be measured with precision. There are several terms used to report the estimates of the energy resources. The most commonly used terms are “reserves” and “resources.” "Reserves" represent that portion of demonstrated resources that can be recovered economically with the application of extraction technology available currently or in the foreseeable future. Reserves include only recoverable energy. “Resources” represent that portion of the energy that is known to exist or even suspected to exist, irrespective of technical or economic viability. So reserves are a subset of resources. Based on data from BP (British Petroleum), proved gas reserves were dominated by three countries: Iran, Russia, and Qatar, which together held nearly half the world's proven reserves. According to the US CIA The World Factbook, the US has the 5th largest reserves of natural gas. Due to constant updates about the shale gas estimates, these are difficult to say with certainty. How Long Will the Reserves Last? How long these reserves do last depends on the rate at which we consume these reserves. For example, let’s assume that we have $100,000 in the bank (reserves) and if we draw 10,000 dollars every year (consumption) the reserve will last for 10 years (\$100,000/\$10,000 per year). However, in this case, we are assuming that we do not add any money to our deposit, and we do not increase our withdrawal. This is generally not true in the case of life of an energy reserve. We may find new reserves, and our energy consumption or production can also increase. In the case of energy reserve, although we know that we might find new resources, we do not know how much we could find. But the consumption can be predicted with some accuracy based on the past rates. Lifetime of current reserves at constant consumption We can calculate the life of current petroleum reserves by dividing the current reserves by current consumption. At the current rate of consumption, the approximate lifetime of the world’s petroleum, natural gas, and coal reserves is 50 years, 52.8 years, and 153 years, respectively. (BP Statistical Review of World Energy)(link is external) At the current rate of consumption, the current U. S. petroleum, natural gas, and coal reserves will last approximately for 4.88 years, 12.2 years, and 258 years, respectively. It is important to note that the entire U.S. petroleum consumption is not coming from the U.S. reserves because we import more than one half of the consumption. Because the U.S imports more than one half of the consumption, the petroleum reserves at the current rate will last about 11 years. If consumption increases in the future, life will be less. However, there is also a chance of adding more reserves with more exploration and discoveries. The increase in consumption can change depending on the price of petroleum and other alternative fuels. Likewise, us moving to electric cars and harnessing unconventional oil reserves can extend the lifetime of these reserves. Therefore, these lifetimes are not carved in stone. It can be debated whether the U.S. reserves will last for 6 years or 10 years or even 20 years, or we may never run out! But there is increasing consensus that we must change our lifestyle. Even if we won't run out, the environmental consequences of continued use are pushing us to change anyway, but more on that later. The Reserves-to-production ratio R/P ratio can change from year to year, similar to our bank balance. We can add more if we make more or consume more. That changes the time we can draw on the balance. The following figures illustrate that these ratios changed. Based production- Energy Reserves, cont'd. One can concentrate on fossil fuels because they are the most consumed energy sources during the late 1980's. Select the most important ones: Shale oil: 1.2 × 1021 J Tar sand: 1.8 × 1021 J Natural gas: 9.5 × 1021 J Petroleum: 11.7 × 1021 J Total: 24.2 × 1021 J In 1986, the energy consumption in the world was 24 × 1019 J, whichmeans that the world’s energy reserves may be enough for about 100 years, given the consumption remained the same as that of 1986 Energy Reserves, cont'd. Considering coal reserves: 200 × 1021 J This will add about 833 years in order to consume coal, but coal hasthe problem of pollution due to high sulfur content. Considering Uranium 235 reserves: 13.7 × 1021 JThis will add 57 years to consume it, but there is a safety problem here. Considering solar power on the U.S. only: 187,000 × 1021 W.× 3600 × 24 × 365 = 58.9 × 1021 J Which is about 2.4 times of the fossil fuel reserves, but it is costly. Considering wind power on the U.S. only: 970 × 1010 W× 3600 × 24 × 365 = 0.3 × 1021 J Which is enough for about 1.25 of the world's needs, but it is costly. CONCLUSION: THERE IS NO MAJIC SOLUTION, ECCEPT WITH CONSERVATION AND DEVELOPMENT AND INVESTMENT IN RENEWABLE ENERGY. See this link: https://www.mospi.gov.in/sites/default/files/publication_reports/Energy_Statistics_2023/Chapter%201- Reserves%20and%20Potential%20for%20Generation. 1.6 Energy Utilization Energy utilization is an expression of the way energy is used. According to type of fuel. It may be expressed in two forms: According to economic sector Table 1.2 and Fig.1.7 show energy utilization in the U.S. in the late1980's. HW. energy utilization and production in the ………. Energy Utilization in Jordan Since the options of sources are not diversified, one may replace the source by type of fuel. According to Ministry of Energy and Mineralresources of 1993, one may come up with the following Table: Consumption, by type of fuel, % Consumption by sector, % LPG Gasoline Jet Kero- Diesel Fuel Asph- Commercial Ind. Trans. Elect. fuel sene oil alt &Residential Gen. 5 10 6 8 25 42 4 18.5 22.2 39.5 19.8 * Liquefied petroleum gas (LPG or LP gas) is a fuel gas which contains a flammable mixture of hydrocarbon gases, specifically propane, propylene, butylene,. Energy Supply and Consumption Energy Growth Fig. 1.9 shows the growth of power vs. time. Energy vs. Economy Energy-GNP Relationship efine: Energy consumption per capita= Energy consumption/ population Gross National Product (GNP) is the total economic activity in a country including all goods and services. GNP per capita = GNP/ population The energy vs. economy situation in any country may be represented byFig.1.1 Notes: If both parameters are high → good If both parameters are low → bad If below the line this is better than above the line because there is savingin energy for the same GNP. Energy-GDP Relationship Gross domestic product (GDP) measures the monetary value of final goods and services— that is, those that are bought by the final user—produced in a country in a given period of time (say a quarter or a year). It counts all of the output generated within the borders of a country. Figure 2 shows Correlation of energy consumption and GDP per person, the graph shows per capita energy consumption (kg oil equivalent) vs. per capita GDP, PPP (current international $). The size of the bubbles denotes the total population per country. All values refer to the year 2011. H.W Ch.2. FueIs A fuel can be considered as a finite resource of chemical potential energy in which energy stored in the molecular structure of compounds is released via complex chemical reactions. Chemical fuels can be classified in a variety of ways, including by phase and availability as shown in Table in below. Some of the basic requirements of a fuel include: high energy density (content), high heat of combustion (release), good thermal stability (storage), low vapor pressure (volatility) and non-toxicity (environmental impact). Any combustion system may be operated on fuel in any of the three states, gaseous, liquid, or solid. Crude oil 1. Chemical and Physical Data Crude oil is a product of the remains of prehistoric plants and animals, buried in the mud of swamps, lakes, and oceans. Over the centuries, layers of mud and organic substrate were subjected to enormous pressures and high temperatures, and a petroleum saturated rock was formed. Four elements must be present for oil to accumulate in commercially useful quantities: source rock, reservoir rock, trap, and seal. These elements allow the crude oil to remain underground and available in large quantities. A source rock is usually sedimentary rock rich in organic matter. The crude oil created by the breakdown of matter migrates from the source rock to a reservoir rock. The reservoir rock contains many tiny pores that store the oil. A trap, either stratigraphic layers of impermeable rock or structural traps, prevents the oil from migrating from the reservoir rock. An impermeable layer, or seal, prevents the oil from rising through or around the trap to the surface. Crude oil has been defined as a highly complex mixture of paraffinic, cycloparaffinic (naphthenic) and aromatic hydrocarbons, containing low percentages of sulfur and little amounts of nitrogen and oxygen compounds. Crude oils are often classified based on chemical composition, according to the proportion of hydrocarbon compounds. Paraffin. Crude oils are rich in straight-chain and branched paraffin hydrocarbons, whereas naphthenic crude oils contain mainly naphthenic and aromatic hydrocarbons. The composition and classification of many crude oils are obtained by ring analysis and by determination of the other components. Crude oils may also be classified by geological source, as arising from productive sands, sandstones, and limestones. The fractional and chemical compositions of crude oil from the same producing sand are usually very similar. There is no clear-cut relationship between the chemical composition of crude oils and their geological age or origin. Crude oils are also referred to as light, medium (intermediate) or heavy, depending on their density. Crude oils are designated in industry according to their suitability for use in various products. Thus, a crude oil may be referred to as a 'gasoline crude', a 'wax crude', a 'lube crude', an 'asphalt crude', and so forth. Chemical composition and physical properties Crude oils are complex mixtures of a vast number of individual chemical compounds. Each crude oil is a unique mixture, not matched exactly in composition or properties by another sample of crude oil. Two typical crude oils, for example, have been characterized by the American Petroleum Institute as shown in Figure n below. Although the mid-points of their respective boiling ranges are similar, they differ considerably in other physical properties, hydrocarbon composition and distribution and sulfur content. The bulk of the compounds present in crude oils are hydrocarbons. Crude oils generally contain the classes of hydrocarbons and other compounds described below. Hydrocarbon compounds (i) Alkanes (paraffins) Alkanes are straight-chain normal alkanes and branched iso-alkanes with the general formula CnH2n+2. The major paraffinic components of most crude oils are in the range Ci to C35, although smaller quantities of alkanes up to C60 or higher may be present. Crude oils vary widely in alkane content. (ii) Cycloalkanes (naphthenes) Cycloalkanes (or cycloparaffins), also called naphthene in the petroleum industry, are saturated hydrocarbons containing structures with carbon atoms linked in a ring. The cycloalkane composition in crude oil worldwide typically varies from 30% to 60%. The most common monocycloalkanes in crude oil are : in the cyclopentane series, having five carbon atoms in the ring, in the cyclohexanes, having a six-membered ring. The most common monocycloalkanes and their composition ranges in crude oil are shown in below. The polycyclic in crude oil are: ln the higher boiling fractions, such as lubricating oils, cycloalkanes with two or more rings are most common, and structures containing up to ten rings have been reported. These polycyclic structures are usually composed of fused five- and six-membered rings. (iii) Aromatic hydrocarbons The Most common aromatic compounds in crude oils are benzene, benzene derivatives and fused benzene ring compounds. The concentration of benzene in crude oil has been reported to range between 0.01 % and i %. (b) Nonhydrocarbon compounds (i) Sulfur compounds Crude oils vary widely in sulfur content, which can range from 0. 1 % to 10% by weight. The following types of sulfur compounds have been identified in crude Oils: - thiols (mercaptans) - sulfides - disulfides - thiophenes. ln the lower distillation range up to about 150°C, the most abundant sulfur compounds are thiols. ln the 150-250°C distillation range, the most abundant compounds are thiocyclo-, thiobicyclo- and thiotricycloalkanes and thiophenes. These sulfur compounds are replaced, in turn, by benzothiophenes and more complex ring structures in the higher distillation ranges. (ii) Nitrogen compounds The nitrogen content of crude oils ranges from trace amounts to 0.9% by weight. The bulk of the nitrogen in fractions that boil below about 200°C is basic nitrogen. The basic compounds often found in crude oils include pyridines and quinolines, e.g.,3-methylpyridine and quinoline, while nonbasic nitrogen compounds include pyrroles, indoles and carbazoles, e.g., carbazole, and amides. (iii) Oxygen compounds The oxygen content of crude oils ranges from 0.06% to 0.4% by weight, most components being alkane and cycloalkane (naphthenic) acids. Other minor components include ketones and phenols. The oxygen content of crude oils increases with boiling range, so that more oxygen-containing compounds are found in distillates that boil above 400°C. (iv) Metal-containing compounds Traces of many metallic compounds can be found in crude oils. Nickel and vanadium compounds have been identified in crude oils at levels ranging from a few parts per million to 200 ppm (mg/ kg) nickel and up to 1200 ppm (mg/ kg) vanadium. These metals occur primarily as complexes which are stable and can be distilled at temperatures above 5000C. (v) Miscellaneous contaminants Crude oil, as it emerges from the well-head, is typically a heterogeneous mixture of solids, liquids and gases, including, in addition to the constituents described above, sand and other sediments, water and water vapour, salts and acid gases such as hydrogen sulfide and carbon dioxide. These contaminants are at least partially separated from the crude oil in surface treatment at the well-head to prepare it for transportation to the refinery. Production and use (a) Production Crude oil production is the process of raising well fluids to the surface and preparing them for further processing at the refinery. Since 1972, about 60 millon barrels of crude oil have been produced each day worldwide, mostly in areas of small population or limited industrial development. Crude oil production begins with preparation of a well, followed by the application of a variety of mechanisms to bring the oil to the surface. There it is treated superficially to prepare it for transport to the refinery (plant) by tanker, by pipeline, or by truck or train. (i) Preparing the well 1- The production operation begins after the well has been drilled and has been evaluated as being economically favorable for production. 2- Pipe is inserted into the well bore in a concentric series to prevent contamination by fresh water. 3- Scratching or charging of shallow sands with abnormal pressures. 4- The first such pipe placed into a well is the conductor pipe, which may be pile drilled or welded into place and may extend to a depth of 150-1500 m. 5- The conductor pipe and all other casings are attached at the surface to the casing head 6- Surface casing is inserted through the conductor pipe and deeper into the well to prevent underground formations of fresh water from becoming contaminated with well fluids and to provide a mechanism for controlling the flow of fluid from the well. (ii) Pumping the crude After finishing the well, oil starts coming up on its own because of the energy in the underground reservoir. This energy makes the oil move. There are different ways this happens, like using dissolved gas, a gas cap, water, or a mix of these to push the oil up. It's like the natural forces doing the work to bring the oil to the surface. As the well keeps producing over time, the natural forces that push the oil up might weaken. So, we might need to use a machine to bring the oil out from the underground reservoir. Some common ways to do this are by using machines like surface pumps, submersible pumps, or a process called gas lift. Sometimes, the pathways that let fluids move in the ground can get clogged (blocked), slowing down oil or gas production. To fix this, we use techniques like acidizing and hydraulic fracturing (breakdown). Acidizing treats the underground rock with special acids to clear blockages, while hydraulic fracturing involves pumping high-pressure water into the well to create deep cracks in the rock, helping oil or gas flow better. These methods keep production going smoothly. (Hi) Surface treatment After getting the crude oil out of the ground, the last step before sending it to the refinery is to clean it up. Remove the dirt, water, and separate the parts that can easily turn into gas. This makes the oil ready for the next steps in the refinery. To prepare natural gas for use, it undergoes a sweetening process. This involves removing water vapor and acidic gases like carbon dioxide and hydrogen sulfide using special materials or liquids. (iv) Transportation and storage Crude oil is transported mainly by tankers and pipelines, with trucks, and barges playing smaller roles. Tankers are loaded either from onshore facilities or offshore platforms. In an oil field, flow lines connect wells to storage tanks, and after treatment, intermediate gathering lines transport oil for further processing and measurement. (v) Production volumes World crude oil production from 1947 to 1986 is shown in Table 8 by geographical region. Over the past 40 years, production has increased more than seven-fold, from 3000 million barrels to 22 000 million barrels per year. Table 9 gives production data for the 20 countries that produced the most crude oil in 1976 and 1986. ln 1986, proven worldwide reserves of crude oil were estimated to be 700000 million barrels. (b) Use Raw crude oil has a long history, with ancient civilizations using it for various purposes like lamps, heating, and building materials. In the twentieth century, it became a crucial global resource, extracted worldwide for energy and the petrochemical industry. Today, due to its inconsistent composition, crude oil isn't directly used; instead, it's sent to refineries for processing into various products. (c) Environmental exposure Estimates indicate that 1.7-8.8 million tonnes of petroleum enter the marine environment yearly, with a best estimate of 3.2 million tonnes. In Nigeria, from 1980 to 1983, approximately 350 million m³ (370 million tonnes) of oil were produced, generating 13 million m³ of wastewater yearly. The average oil concentration in the water ranged from 11.2 to 53.9 mg/l. A study in Australia revealed crude oil as a probable source of aromatic hydrocarbons in estuarine and seawater samples. Other likely sources included refined petroleum products and distillates. In the Shatt al-Arab River in the Arabian Gulf, total hydrocarbon concentrations ranged from 2.7 to 86.7 µg/l, with higher concentrations near port areas. Similar studies in Iraq showed seasonal variations in oil residues, with concentrations ranging from 1.7 to 35.4 µg/l. Highest levels were in winter, averaging 17.4 µg/l, and lowest in summer, averaging 3.1 µg/l. Natural Gas Natural Gas Formation: 1. Methane is formed through various processes: a. Bacterial degradation of organic matter in landfills. b. Heating of petroleum deep within the earth, causing molecular splitting and methane formation. 2. Crude oil buried deeper undergoes more cracking, producing shorter carbon chains. 3. Deeper drilling yields more gas: at great depths, hydrocarbons fully crack into gas. 4. Commercial natural gas deposits are often formed through this process. 5. Coal formation can also release methane as carbon rings condense and fragment. 6. Coalbed methane is emerging as a new source of natural gas. 7. Coal formation involves the formation of carbon rings, releasing methane. Interest is growing in extracting methane from coal seams, known as coalbed methane. This not only provides a new gas source but also reduces gas hazards in coal mines. Methane in coal mines can lead to explosive accidents due to its flammability. Natural gas, often associated with petroleum in the earth's crust, is called associated gas. Under high pressure, natural gas dissolves in crude oil as dissolved gas, liberated during oil extraction. Gas can form a bubble over an oil pool, known as gas cap gas. Non-associated gas results when gas migrates and gets trapped by impervious rock in a reservoir. Note: Hydrpcarbons: Hydrocarbons are colourless gases that have very weak odours. They can be divided into three categories based on the carbon-carbon bonds present. The categories are as follows: (i) Saturated (ii) Unsaturated (ii) Aromatic hydrocarbons 1. The Alkanes General formula for alkanes: CnH2n + 2 and can be subdivided into the following three groups: the linear straight-chain alkanes, branched alkanes, and cycloalkanes. Cycloalkanes are cyclic hydrocarbons, meaning that the carbons of the molecule are arranged in the form of a ring. Cycloalkanes are also saturated, meaning that all of the carbons atoms that make up the ring are single bonded to other atoms (no double or triple bonds). There are also polycyclic alkanes, which are molecules that contain two or more cycloalkanes that are joined, forming multiple rings. Alkanes are the simplest family of hydrocarbons - compounds containing carbon and hydrogen only. Alkanes only contain carbon-hydrogen bonds and carbon-carbon single bonds. The first six alkanes are as follows: methane CH4 ethane C2H6 propane C3H8 butane C4H10 pentane C5H12 hexane C6H14 Alkanes are organic compounds that consist entirely of single-bonded carbon and hydrogen atoms and lack any other functional groups. Alkanes are often called saturated hydrocarbons because they have the maximum possible number of hydrogens per carbon. Methane (CH4), ethane (C2H6), and propane (C3H8) are the simplest Alkanes. General formula for alkanes: CnH2n + 2 and can be subdivided into the following three groups: the linear straight-chain alkanes, branched alkanes, and cycloalkanes. Natural Gas composition: Natural gas is a complex mixture of hydrocarbon (Hydrocarbons are colourless gases that have very weak odours. They can be divided into three categories based on the carbon-carbon bonds present. The categories are as follows:(i) Saturated,(ii) Unsaturated, (iii) Aromatic hydrocarbons) and non- hydrocarbon constituents and exists as a gas under atmospheric conditions. Virtually hundreds of different compounds may be present in natural gas in varying amounts. Even two wells producing from the same reservoir may produce gases of different composition as the reservoir is depleted. Fortunately, however, the major components in most natural gases are paraffin hydrocarbons with smaller amounts — usually only traces - of olefin hydrocarbons, naphthenic hydrocarbons, mercaptans, and non-hydrocarbon compounds. The components of natural gas are either aliphatic (chain) or cyclic (ring) hydrocarbons. Their structures are as follows. Aliphatic hydrocarbons occur in two forms: (a) Paraffin hydrocarbons (b) Olefin hydrocarbons. The most common are saturated hydrocarbons. CH4 - Methane (predominant) C2H6 - Ethane C3H8 - Propane C4H10 – Butane C5H12 – Pentane C6H14 – Hexane C7H16 – Heptane The structural formula for methane is: Isomers Isomers are compounds having the same composition and molecular weight but different properties due to a different structural arrangement. The structural formulae for butane isomers are as follows: Olefin hydrocarbons Olefin hydrocarbons have the general formula of CnH2n and are classed as unsaturated hydrocarbons. They usually occur only in traces. C2H4 – Ethene C3H6 – Propene C4H8 - Butene The structural formula for butene is: Cyclic or Ring Hydrocarbons Cyclic hydrocarbons also occur in traces most of the time. They are of two kinds: naphthenic, and aromatic hydrocarbons. For simplicity, only the ring structures will be shown. Naphthenic hydrocarbons Naphthenic hydrocarbons are saturated cyclic hydrocarbons with the general formula of CnH2n Aromatic hydrocarbons Aromatic hydrocarbons are unsaturated cyclic hydrocarbons classified by the number of six— carbon rings in the molecule. The structures of benzene, toluene, and ethyl benzene are as follows: Structures of more complex aromatics such as xylenes in which two or more methyl groups or side chains are attached to the benzene ring are shown below: Nonhydrocarbon Components Some non-hydrocarbon components of natural gas are as follows: Nitrogen N2 Carbon dioxide CO2 Hydrogen sulfide H2S Helium He Water vapor H20 Carbonyl sulfide COS Carbon disulfide CS2 Sulfur S Mercaptans *RSH Methyl Mercaptan and Ethyl Mercaptan Methyl mercaptan and ethyl mercaptan are the two mercaptan compounds most commonly found in natural gas. Their structures are as follows: Using Natural Gas: 1. Natural gas is an excellent fuel with simple composition and high calorific value. 2. Requires minimal processing compared to coal and petroleum. 3. Being in a gaseous state, it easily mixes with oxygen for efficient combustion. 4. Produces no ash residue upon combustion, as it contains no minerals. 5. Overall, natural gas is an ideal and straightforward fuel option. Processing Natural Gas: 1. When brought to the surface, pentane and hexane in natural gas turn into liquid fuel, known as natural gasoline. 2. Cooling the gas allows propane and butane to condense into liquids, valuable for the chemical industry. 3. These compounds, along with ethane, are used in various synthetic products. 4. Propane-rich liquid is marketed as Liquefied Petroleum Gas (LPG), commonly used in rural areas, camper trailers, and gas-heated barbecue grills. 5. Natural gas containing hydrogen sulfide (sour gas) is undesirable due to its foul odor and toxicity. 6. Sour gas must undergo sweetening, a process to remove H2S before sale. 7. After processing and removal of other hydrocarbons, the gas, mainly methane with small ethane amounts, is ready for sale with a heating value of about 1000 BTU/ft³. In general "The gas sold doesn't have a specific chemical makeup but must meet certain properties. Changing raw gas from the reservoir, often at pressures around 4000 kPa to higher pressures like 35000 kPa, and saturated with water vapor. This processing involves removing acid gases (H2S and CO2), potentially converting H2S to elemental sulfur for economic or environmental reasons. All free liquids, including hydrocarbons and water, are removed. Typically, hydrocarbons heavier than pentanes, as well as substantial amounts of propane and butane, are also taken out. Understanding fluid flow, distillation, heat transfer, and other topics, which will be discussed later in the book, is crucial for this removal process." Transporting Natural Gas: 1. Mainly transported through pipelines, where gas can be at 65 atmospheres pressure, moving at about 15 miles per hour. (as used in USA) 2. liquefied natural gas (LNG), turning methane into liquid at -164 °C, is shipped in refrigerated-tank ships, acting like large floating thermos bottles. (as used Algeria) Natural Gas Combustion: 1. Natural gas is a premium fuel, especially for homes, with the highest calorific value among fossil fuels (1000 BTU/ft³ or 24,000 BTU/lb). 2. Heat generation is easily controlled with instantaneous on/off capability of natural gas burners. 3. It is a clean fuel, producing no ashes or mess during combustion. 4. No fuel storage space is needed at home, allowing better use of space. 5. Gas is automatically available when the furnace control valve is opened, eliminating the need for arrangements with fuel companies. 6. Handling fluids (gases and liquids) is more convenient than solids. Coal Coal is a non-clastic sedimentary rock. They are the fossilized remains of plants and are in flammable black and brownish-black tones. Its main element is carbon, but it can also contain different elements such as hydrogen, sulfur and oxygen. Unlike coal minerals, it does not have a fixed chemical composition and crystal structure. Depending on the type of plant material, varying degrees of carbonization and the presence of impurities, different types of coal are formed. There are 4 recognized varieties: Lignite (leg night) is the lowest grade and is the softest and least charred. Sub-bituminous (sub bet uminos /or/sub- byt uminos) coal is dark brown to black. Bituminous coal is the most abundant and is often burned for heat generation. Anthracite (anthra site) is the highest grade and most transformed form of coal. It contains the highest percentage of low-emission carbon and would be an ideal fuel if it weren’t for comparatively less. Coal is mainly used as a fuel. Coal has been used for thousands of years, but its real use began with the invention of steam engines after the industrial revolution. Coal provides two-fifths of electricity production worldwide and coal is used as the main fuel in iron and steel production facilities. Coal Classification 1- Anthracite contains 86%–97% carbon and generally has the highest heating value of all ranks of coal. The highest rank of coal. It is a hard, brittle, and black lustrous (lust rose )coal, often referred to as hard coal, containing a high percentage of fixed carbon and a low percentage of volatile matter. the highest grade of coal, is a harder, glossy black coal used primarily for residential and commercial space heating. 2- Bituminous coal contains 45%–86% carbon. Bituminous coal is a middle rank coal between subbituminous and anthracite. Bituminous coal usually has a high heating (Btu) value. a dense sedimentary rock, usually black, but sometimes dark brown, often with well-defined bands of shiny and dull material. It is primarily used as a fuel in the production of steam-electric power and in the production of coke. 3- Subbituminous coal typically contains 35%–45% carbon, and it has a lower heating value than bituminous coal. Subbituminous coal is black in color and is mainly dull (not shiny). Subbituminous coal has low-to-moderate heating values and is mainly used in electricity generation. 4- Lignite contains 25%–35% carbon and has the lowest energy content of all coal ranks. Lignite coal deposits tend to be relatively young and were not subjected to extreme heat or pressure. Lignite is crumbly and has high moisture content, which contributes to its low heating value. Lignite coal, aka brown coal, is the lowest grade coal with the least concentration of carbon. Lignite has a low heating value and a high moisture content and is mainly used in electricity generation. the lowest level of coal, the most harmful to health, is used almost exclusively as a fuel for electric power generation. Lignite is the youngest type of coal and contains the least amount of carbon, while anthracite is the oldest and has the highest carbon content. Generally, coal with higher carbon content has a higher energy content and burns more efficiently. Coal also contains varying amounts of minerals such as silica, alumina, iron, calcium, sodium, and potassium, which can affect its combustion properties and environmental impact when burned. Chemical composition Lignite is the youngest type of coal and contains the least amount of carbon, while anthracite is the oldest and has the highest carbon content. Generally, coal with higher carbon content has a higher energy content and burns more efficiently. Coal also contains varying amounts of minerals such as silica, alumina, iron, calcium, sodium, and potassium, which can affect its combustion properties and environmental impact when burned. mainly connected in numerous chemical compounds: - carbonates (CaCO 3, MgCO 3 and in others), - carbon dioxide in air (CO 2), - in organic compounds. Coal formation The process of coal formation from organic compounds includes two distinct phases: 1- biochemical : plant material – peat – lignite 2- geochemical : phase lignite – bituminous coal – anthracite Coal chemical structure Physical properties Coal has a variety of physical properties, including: 1. Color: Coal can range in color from black to brown to grayish. 2. Hardness: Coal can range in hardness from very soft and crumbly, like graphite, to very hard, like anthracite. 3. Density: Coal has a lower density than many rocks and minerals, making it relatively lightweight. 4. Porosity (poro sity): Coal can be very porous, with small spaces between the coal particles. 5. Conchoidal fracture: Coal often fractures in a smooth, curved pattern, known as conchoidal fracture. 6. Luster: Coal has a dull to shiny luster, depending on the type of coal. 7. Streak: Coal produces a black or dark brown streak when rubbed on a white, unglazed porcelain plate. Mining and processing of coal Coal is typically extracted from underground or surface mines. Underground Mining processes which includes: 1. Room and Pillar methods include: Tunnels dug (drilled) into coal seam (layers). Leave pillars (columns) to support the roof. 2. Longwall methods include: Mine a long wall of coal in a single slice created. Roof collapses (falls) behind the mining machine. 3. Retreat Mining: Remove pillars (columns) from a previously mined area. Surface Mining processes which include: 1. Strip Mining: Remove excess material in strips to access coal. 2. Mountaintop Removal: Entire mountaintops were removed to access coal. 3. Open-Pit (hole) Mining: Create a large hole to extract coal. Processing Coal: 1. Extraction: Crushing, screening, washing remove impurities. 2. Drying: Reduce moisture content. 3. Chemical Treatment: Coal cleaning to remove sulfur and impurities. Coal Processing: 1- Extraction: Crushing, screening, washing to remove impurities. 2- Chemical Treatment: Remove sulfur and impurities. 3- Conversion: Coal may be converted to liquid or gaseous fuels. 4- Coal washing: this process includes the following: a-Preparation: Crushed coal mixed with water and chemicals. b- Slurry Formation: Creates a slurry for processing. c- separation by: - Passed through screens and cyclones. - Separates coal from impurities (rock, ash, sulfur). d- Grading: Coal graded based on size. 5- Crushing and Grinding: Makes coal suitable for burning or other uses. 6- Sulfur Removal: Processes to remove sulfur and pollutants (polo tent). 7- Carbonization: Converts coal to coke for steel-making. FOSSIL FUELS: ENVIRONMENTAL EFFECTS The National Energy Strategy in air quality emphasizes: Emission Reduction Goals: The primary objective is to reduce energy-related emissions, with a specific focus on achieving and sustaining compliance with Air Quality Standards for carbon monoxide and ozone. Control Strategies: Develop cost-effective and flexible control strategies to reduce energy-related emissions of sulfur dioxide (SO2) and nitrogen oxides (NOx). This involves implementing measures to efficiently manage and decrease these pollutants. Energy production and use pose significant environmental challenges. Policy approaches must align energy and environmental issues to ensure that economic growth and environmental protection are achieved together. The Administration is reinventing environmental protection, creating regulatory systems that are more flexible and accountable, emphasizing pollution prevention over “end-of-pipe” clean-up, and fostering the development of new energy-efficient technologies to meet both economic and environmental goals. A pollutant is a substance, often harmful, not naturally present in the environment or found in abnormally high concentrations. Principal air pollutants from fossil fuel combustion include carbon monoxide (CO2), sulfur oxides (SOx), nitrogen oxides (NOx), and particulates (fine soot and ash). Unburned hydrocarbons and lead compounds were historically contributors to air pollution, but the reduction of leaded gasoline has significantly diminished this issue. These primary pollutants can further interact with the environment to generate additional deleterious effects. Examples of these effects (secondary pollutants) are acid rain and smog, the greenhouse effect and the high ozone levels in the air we breathe. (This last effect should not be confused with the ozone layer depletion, which is also becoming an environmental problem but has no direct relationship with fossil fuel utilization.). Primary Air Pollutants (polo tents) 1- Carbon Monoxide. Carbon monoxide (CO) is a product of incomplete combustion of any fuel. It is both a highly poisonous (pois nes) gas and the principal constituent of photochemical smog (see below). Table in below summarizes the effects of CO exposure on human health. The main culprits of CO pollution are the urban automobiles and transportation vehicles in general. Un con sciousness 2- Sulfur Oxides. Sulfur oxides arise during combustion from oxidation of sulfur in sulfur containing fuels (some coals and some petroleum-based products). The principal product is sulfur dioxide (SO2): Sulfur dioxide has an annoying odor and it irritates the eyes and respiratory tract. Still, SO2 itself is not highly dangerous. However, when it is released to the atmosphere, it can react with oxygen in the air to form sulfur trioxide: Sulfur trioxide causes an irritant (irri tent) to the respiratory tract, causing coughing and choking (choo king) even at low concentrations. Sulfur trioxide dissolves in water to form sulfuric acid, a potent corrosive (corro ziv) agent (egent). Sulfur trioxide can absorb moisture from the air, forming fine droplets of sulfuric acid that, when inhaled (in held), can harm the respiratory system and lead to bronchitis (bron kytes) with chronic exposure. Additionally, it readily dissolves in raindrops, contributing to the formation of acid rain when it falls to the earth.