SDS-PAGE Lab 2 Reading Material PDF
Document Details
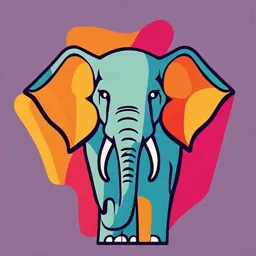
Uploaded by BrainiestLavender7620
Valdosta State University
Tags
Summary
This document provides a detailed explanation of SDS-PAGE, a technique used to separate proteins based on their molecular weights. It outlines the steps involved, from sample preparation to visualization and interpretation of results. The document also touches upon osmosis and different solutions used in the process.
Full Transcript
https://www.youtube.com/watch?v=MILiO1XnuqQ&t=230s https://www.youtube.com/watch?v=aa2OSzXpXOQ SDS-PAGE (sodium dodecyl sulfate-polyacrylamide gel electrophoresis) is a widely used technique for separating proteins based on their molecular weights. The process involves several steps: 1. Sample...
https://www.youtube.com/watch?v=MILiO1XnuqQ&t=230s https://www.youtube.com/watch?v=aa2OSzXpXOQ SDS-PAGE (sodium dodecyl sulfate-polyacrylamide gel electrophoresis) is a widely used technique for separating proteins based on their molecular weights. The process involves several steps: 1. Sample Preparation: Proteins are denatured and coated with SDS. SDS is an anionic detergent that binds to proteins and unfolds them into linear chains, masking the intrinsic charge of the proteins and providing a uniform negative charge based primarily on the mass of the polypeptide chain. 2. Loading: The denatured protein samples are loaded into the wells of a polyacrylamide gel. The gel is composed of a cross-linked matrix of polyacrylamide, which forms a porous network that allows proteins to migrate through it based on their size. 3. Electrophoresis: An electric field is applied to the gel. Because the proteins are negatively charged due to the bound SDS, they move towards the positively charged anode (opposite the direction of electron flow) in the electric field. The migration rate of the proteins depends on their molecular weight. 4. Separation by Size: Smaller proteins can migrate more quickly through the pores of the gel matrix, while larger proteins move more slowly. Therefore, during electrophoresis, the proteins separate out according to their molecular weights, with smaller proteins traveling farther from the point of application. 5. Visualization: After electrophoresis is complete, the proteins in the gel are typically stained to visualize them. Common stains include Coomassie Blue, silver stain, or fluorescent dyes that bind specifically to proteins. 6. Interpretation: The separated proteins appear as distinct bands or spots on the gel. The position of each band corresponds to the molecular weight of the protein. By comparing the migration of unknown proteins to known molecular weight markers (standard proteins of known sizes run alongside the samples), the molecular weights of the unknown proteins can be estimated. In SDS-PAGE (sodium dodecyl sulfate-polyacrylamide gel electrophoresis), the gel is typically composed of two regions: the stacking gel and the separating gel. Each of these gel regions serves a specific purpose in facilitating the separation of proteins based on their molecular weights. Here's how stacking and separating gels work in SDS-PAGE: 1. Stacking Gel: Purpose: The stacking gel is the initial region of the gel that the protein samples enter after they are loaded into the wells of the gel. Its primary function is to concentrate and focus the protein samples into a narrow, sharp zone or "stack" before they enter the separating gel. Composition: The stacking gel has a lower percentage of acrylamide compared to the separating gel, typically around 4-5%. This lower percentage creates a more porous matrix that allows proteins to migrate quickly and without significant separation based on molecular weight. Buffer System: The pH and ionic composition of the stacking gel buffer are designed to create a high-resistance environment that slows down protein movement. This leads to protein stacking at the interface between the stacking gel and the separating gel. Electric Field: When an electric field is applied during electrophoresis, proteins migrate into the stacking gel. Because of the high-resistance buffer system, all proteins are focused into a tight zone, regardless of their molecular weight. This stacking effect compresses the protein bands into a concentrated front that enters the separating gel as a sharp starting point. 2. Separating Gel: Purpose: The separating gel is the main region of the gel where protein separation based on molecular weight occurs. Proteins that have been stacked in the stacking gel enter the separating gel and begin to separate based on their size. Composition: The separating gel typically has a higher percentage of acrylamide, often ranging from 8% to 15% depending on the desired resolution for separating proteins of different sizes. Gradient Gels: In some cases, separating gels may be gradient gels, where the acrylamide concentration gradually increases from top to bottom. Gradient gels allow for improved resolution of proteins across a wider range of molecular weights. SDS-PAGE Principle: In the separating gel, SDS-bound proteins migrate through the gel matrix in response to the electric field. Smaller proteins move more quickly and travel farther through the gel, while larger proteins move more slowly and remain closer to the point of origin. Visualization: After electrophoresis is complete, proteins within the separating gel are visualized using stains or detection methods to analyze their migration pattern and estimate their molecular weights based on comparison with protein markers of known sizes. Osmosis: Osmosis is the movement of solvent molecules (usually water) across a semi-permeable membrane from an area of lower solute concentration to an area of higher solute concentration, in order to equalize the concentration on both sides of the membrane. This process occurs spontaneously and does not require energy. Hypertonic Solution: A hypertonic solution has a higher concentration of solutes (such as salts or sugars) compared to another solution. When a cell or a semi-permeable membrane is placed in a hypertonic solution, water molecules will move out of the cell or membrane, causing it to shrink or crenate. This is because water moves from an area of lower solute concentration (inside the cell or membrane) to an area of higher solute concentration (outside the cell or membrane). Example of Hypertonic Solution: When you place a red blood cell (which has a higher solute concentration inside compared to the surrounding solution) into a hypertonic saline solution, water will move out of the cell, causing it to shrink and become wrinkled. Isopropanol solutions can be hypertonic compared to the cytoplasm of RBCs, meaning they have a higher solute concentration. This hypertonic environment can drive the movement of water out of the cells, leading to cellular dehydration. Hypotonic Solution: A hypotonic solution has a lower concentration of solutes compared to another solution. When a cell or a semi-permeable membrane is placed in a hypotonic solution, water molecules will move into the cell or membrane, causing it to swell or even burst (lyse). This is because water moves from an area of higher solute concentration (outside the cell or membrane) to an area of lower solute concentration (inside the cell or membrane). Example of Hypotonic Solution: If you place a red blood cell (which has a higher solute concentration inside) into pure water (a hypotonic solution), water will rush into the cell, causing it to swell and potentially burst. Isopropanol is typically less dense than water, which means that when it mixes with water or bodily fluids, it can create a hypotonic environment. In a hypotonic solution, water moves into the red blood cells (osmosis), causing them to swell and potentially burst. Isotonic Solution: An isotonic solution has the same concentration of solutes as another solution. When a cell or a semi-permeable membrane is placed in an isotonic solution, there is no net movement of water across the membrane. The water concentration is equal on both sides, so the cell retains its normal shape and size. Example of Isotonic Solution: Normal saline (0.9% NaCl) is often used as an isotonic solution. When you place a red blood cell into normal saline, there is no net movement of water, and the cell maintains its normal shape without shrinking or swelling. In summary: Hypertonic: Higher solute concentration; causes water to move out of cells (crenation). Hypotonic: Lower solute concentration; causes water to move into cells (swelling or lysis). Isotonic: Equal solute concentration; no net movement of water, so cells maintain their shape and size.