Pulmonary Physiology PDF
Document Details
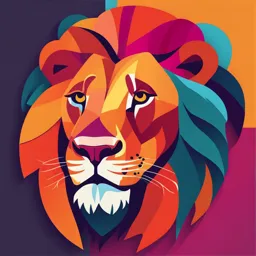
Uploaded by AlluringFoil
Đại học Quốc tế Hồng Bàng
Tags
Summary
This document discusses pulmonary physiology, including the structure of the respiratory system, lung volumes, gas exchange, and mechanics of breathing. It details concepts such as anatomic and physiologic dead space, lung compliance, and the control of breathing.
Full Transcript
have 23 divisions Conducting zone important, these beta2 receptors are activated by circulating epinephrine released from the adrenal medulla and by "2-adrenergic agonists such as isoproterenol. (2) Parasympathetic cholinergic neurons activate muscarinic receptors, which leads to contraction and con...
have 23 divisions Conducting zone important, these beta2 receptors are activated by circulating epinephrine released from the adrenal medulla and by "2-adrenergic agonists such as isoproterenol. (2) Parasympathetic cholinergic neurons activate muscarinic receptors, which leads to contraction and constriction of the airway airrways Sturcture of the respiratory system activate beta 2 receptors on bronchial smooth muscle, which leads to relaxation and dilation of the airways. Respiratory zone Pulmonary blood flow Lung capacities Volumes Capacities Anatomic dead space The anatomic dead space is the volume of the conducting airways including the nose (and/or mouth), trachea, bronchi, and bronchioles. It does not include the respiratory bronchioles and alveol physiologic dead space space. By definition, the physiologic dead space is the total volume of the lungs that does not participate in gas exchange. Physiologic dead space includes the anatomic dead space of the conducting airways plus a functional dead space in the alveoli. Dead space Lung volumes and capacities Often 150 ml In normal persons, the physiologic dead space is nearly equal to the anatomic dead space. ventilation rates alveolar ventilation equation alveolar gas equation Forced expiratory volumes FEV1/FVC in normal person is 0.8 if < 0,7 => considering patient with COPD muscles of inspiration Muscles used for breathing Muscles of expiration Some notions Transmural pressure is the pressure across a structure. For example, transpulmonary pressure is the difference between intraalveolar pressure and intrapleural pressure. (The intrapleural space lies between the lungs and the chest wall.) The compliance of the lungs and chest wall is inversely correlated with their elastic properties or elastance. The greater the amount of elastic tissue, the greater the tendency to “snap back,” and the greater the elastic recoil force, but the lower the compliance. On inspiration limb - In the initial part of the inspiration curve, at lowest lung volumes, the lung surface area is increasing faster than surfactant can be added to the liquid layer; thus surfactant density is low, surface tension is high, compliance is low, and the curve is flat. - As inflation proceeds, the surfactant density increases, which decreases surface tension, increases compliance, and increases the slope of the curve. compliance of the lungs On expiration limb - During deflation of the lung (expiration limb), lung surface area decreases faster than surfactant can be removed from the liquid lining and the density of surfactant molecules rapidly increases, which decreases surface tension and increases compliance; thus the initial portion of the expiration limb is flat. - As expiration proceeds, surfactant is removed from the liquid lining and the density of surfactant remains relatively constant, as does the compliance of the lung compliance of the chest wall This negative intrapleural pressure is created by two opposing elastic forces pulling on the intrapleural space: 1/ The lungs, with their elastic properties, tend to collapse 2/the chest wall, with its elastic properties, tends to spring out - First, without the negative intrapleural pressure to hold the lungs open, the lungs collapse. - Second, without the negative intrapleural pressure to keep the chest wall from expanding, the chest wall springs out. Volume is FRC (functional residual capacity) pressure volume curves for the lungs, chest wall, and combined lung and chest wall Volume is less than FRC Compliance there is less volume in the lungs and the collapsing (elastic) force of the lungs is smaller. The expanding force on the chest wall is greater, however, and the combined lung and chest-wall system “wants” to expand. (Notice on the graph that at volumes less than FRC, the collapsing force on the lungs is smaller than the expanding force on the chest wall and that airway pressure for the combined system is negative; thus the combined system tends to expand, as air flows into the lungs down the pressure gradient.) Subtopic 1 Volume is greater than FRC there is more volume in the lungs and the collapsing (elastic) force of the lungs is greater. The expanding force on the chest wall is smaller, however, and the combined lung and chest-wall system “wants” to collapse. (Notice on the graph that at volumes greater than FRC, the collapsing force on the lungs is greater than the expanding force on the chest wall and that airway pressure for the combined system is positive; thus the overall system tends to collapse, as air flows out of the lungs down the pressure gradient.) Emphysema Lost of elastic fiber in the lung => result, at a given volume, the collapsing (elastic recoil) force on the lungs is decreased. At the original value for FRC, the tendency of the lungs to collapse is less than the tendency of the chest wall to expand, => A patient with emphysema is said to breathe at higher lung volumes (in recognition of the higher FRC) and will have a barrel-shaped chest. Fibrosis associated with stiffening of lung tissues and decreased compliance. A decrease in lung compliance is associated with a decreased slope of the volume-versus-pressure curve for the lung. At the original FRC, the tendency of the lungs to collapse is greater than the tendency of the chest wall to expand and the opposing forces will no longer be balanced Diseases of lung compliance Mechanics of breathing Surface tension of alveoli Laplace's Law Without surfactant, the law of Laplace predicts that the small alveolus will collapse (atelectasis). With surfactant present, the same small alveolus will remain open (inflated with air) because the collapsing pressure has been reduced. Surfactant the collapsing pressure has been reduced. Surfactant is synthesized from fatty acids by type II alveolar cells. The exact composition of surfactant remains unknown, but the most important constituent is dipalmitoyl phosphatidylcholine (DPPC). Mechanism of surfactant reduce surface tension based on the amphipathic nature of the phospholipid molecules (i.e., hydrophobic on one end and hydrophilic on the other). The DPPC molecules align themselves on the alveolar surface, with their hydrophobic portions attracted to each other and their hydrophilic portions repelled. Intermolecular forces between the DPPC molecules break up the attracting forces between liquid molecules lining the alveoli (which had been responsible for the high surface tension) Surfactant provides another advantage for pulmonary function: It increases lung compliance airway resistance Poiseuille law => example, if the radius of an airway decreases by a factor of 2, resistance does not simply increase twofold, it increases by 24, or 16-fold. When resistance increases by 16-fold, air flow decreases by 16-fold, a dramatic effect. radius. However, because of their parallel arrangement, the smallest airways do not have the highest collective resistance. These effects can be simulated by muscarinic agonists (e.g., muscarine and carbachol) and can be blocked by muscarinic antagonists (e.g., atropine). Constriction of bronchial smooth muscle also occurs in asthma and in response to irritants Parasympathetic stimulation produces constriction of bronchial smooth muscle air flow, pressure, and resistance relationships Autonomic nervous system via stimulation of beta2 receptors. Relaxation of bronchial smooth muscle results in increases in airway diameter and decreases in resistance to air flow. Sympathetic stimulation produces relaxation of bronchial smooth muscle Therefore #2 agonists such as epinephrine, isoproterenol, and albuterol produce relaxation of bronchial smooth muscle, which underlies their usefulness in the treatment of asthma. changes in airway resistance Lung volume decreased lung volume causes increased airway resistance (even to the point of airway collapse) and increased lung volume causes decreased airway resistance. Air viscosity decreased air viscosity -> reduced air resistance and the opposite also true Mechanism for the stated sentence: is, alveoli tend to hold their neighbors open by radial traction or mechanical tethering. When alveoli are more inflated (higher lung volume), they pull on both adjacent alveoli and nearby bronchioles, pulling the bronchioles open and decreasing their resistance. At rest, no air is moving into or out of the lungs. Alveolar pressure equals atmospheric pressure, rest At rest, intrapleural pressure is negative, or approximately −5 cm H2O - During inspiration, the diaphragm contracts, causing the volume of the thorax to increase. As lung volume increases, the pressure in the lungs must decrease. (Boyle’s law states that P × V is constant at a given temperature.) inspiration - Halfway through inspiration, alveolar pressure falls below atmospheric pressure (−1 cm H2O). The pressure gradient between the atmosphere and the alveoli drives air flow into the lung. Air flows into the lungs until, at the end of inspiration the FRC plus one tidal volume (FRC + VT). During inspiration, intrapleural pressure becomes even more negative than at rest. There are two explanations for this effect: (1) As lung volume increases, the elastic recoil of the lungs also increases and pulls more forcefully against the intrapleural space, and (2) airway and alveolar pressures become negative Together, these two effects cause the intrapleural pressure to become more negative, or approximately −8 cm H2O at the end of inspiration. breathing cycle expiration Normally, expiration is a passive process. Alveolar pressure becomes positive (higher than atmospheric pressure) because the elastic forces of the lungs compress the greater volume of air in the alveoli. When alveolar pressure increases above atmospheric pressure air flows out of the lungs and the volume in the lungs returns to FRC is Will the lungs and airways collapse under these conditions of positive intrapleural pressure? No, as long as the transmural pressure is positive, the airways and lungs will remain open. forced expiration = During a normal forced expiration, transmural pressure across the airways is airway pressure minus intrapleural pressure, or +5 cm H2O (+25 − [+20] = +5 cm H2O); transmural pressure across the lungs is alveolar pressure minus intrapleural pressure, or +15 cm H2O (+35 − [+20] = +15 cm H2O). Therefore both the airways and the alveoli will remain open because transmural pressures are positive. In person wiith emphysema forced expiration may cause the airways to collapse. In emphysema, lung compliance increases because of loss of elastic fibers. During forced expiration, intrapleural pressure is raised to the same value as in the normal person, +20 cm H2O. However, because the structures have diminished elastic recoil, alveolar pressure and airway pressure are lower than in a normal person. The transmural pressure gradient across the lungs remains a positive expanding pressure, +5 cm H2O, and the alveoli remain open. However, the large airways collapse because the transmural pressure gradient across them reverses, becoming a negative (collapsing) transmural pressure of −5 cm H2O. general gas law Boyle's law Gas law P1.V1=P2.V2 , equal with a constant number => if P increase V will decrease and opposite Gas laws Dalton's law of partial pressures Henry's law for concentrations of dissolved gases Diffusion of gases-Fick's law forms of gases in solution Gas exchange In avep;ar air PAO2 is 100 mm Hg, which is less than in inspired air, and PACO2 is 40 mm Hg, which is greater than in inspired air. These changes occur because O2 leaves alveolar air and is added to pulmonary capillary blood, and CO2 leaves pulmonary capillary blood and enters alveolar air Blood entering the pulmonary capillaries is mixed venous blood. The PO2 is relatively low, at 40 mm Hg, because the tissues have taken up and consumed O2; the PCO2 is relatively high, at 46 mm Hg, because the tissues have produced CO2 and added it to venous blood. Diffusion limited gas exchange means that the total amount of gas transported across the alveolarcapillary barrier is limited by the diffusion process. Perfusion limited gas exchange means that the total amount of gas transported across the alveolarcapillary barrier is limited by blood flow overviewfas transport in the lungs diffusion limited and perfusion limited gas exchanve O2 transport perfusion limited and diffusion limite Diffision limited distance for gases and decreasing DL. This increased diffusion distance slows the rate of diffusion of O2 and prevents equilibration of O2 between alveolar air and pulmonary capillary blood. In these cases, the partial pressure gradient for O2 is maintained along the entire length of the capillary, converting it to a diffusion-limited process Perfusion limited PAO2 is constant at 100 mm Hg. At the beginning of the capillary, PaO2 is 40 mm Hg, reflecting the composition of mixed venous blood. There is a large partial pressure gradient for O2 between alveolar air and capillary blood, which drives O2 diffusion into the capillary. As O2 is added to pulmonary capillary blood, PaO2 increase low. Equilibration of O2 occurs about onethird of the distance along the capillary, at which point PaO2 becomes equal to PAO2, and unless blood flow increases, there can be no more net diffusion of O2. Thus under normal conditions O2 transport is perfusion limited. O2 transport at high altitude instead of the normal gradient at sea level of 60 mm Hg (100 mm Hg − 40 mm Hg). This reduction of the partial pressure gradient means that diffusion of O2 will be reduced, equilibration will occur more slowly along the capillary, and complete equilibration will be achieved at a later point along the capillary Increases in Pco2 and decreases in pH CO2 increases due to metaoblic => PCO2 increase => cause increase in H+ concentration => pH decreases => low pH concentration cause hemoglobin to decrease it affinity to O2 => releashed more O2 to adapt to body's need. =>Bohr effect Dissolved O2 Forms of O2 in blood O2 bound to hemoglobin O2binding capacity and O2 content O2 hemoglobin dissociation curve O2 delivery to tissues Oxxygen transport in blood changes in the O2 hemoglobin dissociation curve Erythropoeitin Shift to the right Subtopic 1 EPO synthesis is induced in the kidney in response to hypoxia in the following steps 1. When there is decreased O2 delivery to the kidneys (hypoxia), either due to decreased hemoglobin concentration or decreased PaO2, there is increased production of the alpha subunit of hypoxia-inducible factor 1 (hypoxiainducible factor 1$). 2. Hypoxia-inducible factor 1% acts on fibroblasts in the renal cortex and medulla to cause synthesis of the messenger ribonucleic acid (mRNA) for EPO. 3. The mRNA directs increased synthesis of EPO. 4. EPO then acts to cause differentiation of proerythroblasts. 5. Proerythroblasts undergo further steps in development to form mature erythrocytes (red blood cells). These further maturation steps do not require EPO ! Increases in temperature. The increases in temperature also cause a right shift of the O2-hemoglobin dissociation curve and an increase in P50, facilitating unloading of O2 in the tissues. Considering the example of exercising skeletal muscle, this effect also is logical. ! Increases in 2,3-diphosphoglycerate (2,3DPG) concentration - 2,3-DPG is a byproduct of glycolysis in red blood cells. 2,3-DPG binds to the # chains of deoxyhemoglobin and reduces their affinity for O2. This decrease in affinity causes the O2hemoglobin dissociation curve to shift to the right and facilitates unloading of O2 in the tissues. - 2,3-DPG production increases under hypoxic conditions. For example, living at high altitude causes hypoxemia, which stimulates the production of 2,3-DPG in red blood cells. In How the kidney distinguish between lack of RBC or lack of oxygen only This distinguishing ability is based on the fact that decreased renal blood flow causes decreased glomerular filtration, which leads to decreased filtration and reabsorption of Na+. Because O2 consumption in the kidneys is strongly linked to Na+ reabsorption, decreased renal blood flow results in both decreased O2 delivery and decreased O2 consumption; thus renal O2 delivery and renal O2 consumption remain matched in that scenario and, as is appropriate, the kidney is not alerted to a need for more erythrocytes. If there is decreased O2 content of arterial blood, then the kidney is alerted to a need for more erythrocytes. Dissolved Co2 Carbaminohemoglobin Carbon dioxide transport in blood Forms of CO2 in blood HCO3- Sinh lý phổi pulmonary blood flow, pressure, and resistance relationships 1. In the tissues, CO2 is produced from aerobic metabolism. CO2 then diffuses across the cell membranes and across the capillary wall, into the red blood cells. The transport of CO2 across each of these membranes occurs by simple diffusion, driven by the partial pressure gradient for CO2. 2. Carbonic anhydrase is found in high oncentration in red blood cells. It catalyzes the hydration of CO2 to form H2CO3. In red blood cells, the reactions are driven to the right by mass action because CO2 is being supplied from the tissue. 3. In the red blood cells, H2CO3 dissociates into H+ and HCO3 −. The H+ remains in the red blood cells, where it will be buffered by deoxyhemoglobin, and the HCO3 − is transported into the plasma in exchange for Cl− (chloride). 4. If the H+ produced from these reactions remained free in solution, it would acidify the red blood cells and the venous blood. Therefore H+ must be buffered so that the pH of the red blood cells (and the blood) remains within the physiologic range. The H+ is buffered in the red blood cells by deoxyhemoglobin and is carried in the venous blood in this form 5. The HCO3 − produced from these reactions is exchanged for Cl− across the red blood cell membrane (to maintain charge balance), and the HCO3 − is carried to the lungs in the plasma of venous blood. Cl−-HCO3 − exchange, or the Cl− shift, is accomplished by an anion exchange protein called band three protein Subtopic 1 All of the reactions previously described occur in reverse in the lungs Pulmonary blood flow is directly proportional to the pressure gradient between the ulmonary artery and the left atrium and is inversely proportional to the resistance of the pulmonary vasculature Hypoxic vasoconstriction By far, the major factor regulating pulmonary blood flow is the partial pressure of O2 in alveolar gas, PAO2. Decreases in PAO2 produce pulmonary vasoconstriction hypoxic vasoconstriction occurs as an adaptive mechanism, reducing pulmonary blood flow to poorly ventilated areas where the blood flow would be “wasted.” Thus pulmonary blood flow is directed away from poorly ventilated regions of the lung, Hypoxic vasoconstriciton mechanism Not well understood At high altitude mixture, PAO2 is reduced throughout the lungs, not just in one region. The low PAO2 produces global vasoconstriction of pulmonary arterioles and an increase in pulmonary vascular resistance.=> In response to the increase in resistance, pulmonary arterial pressure increases. In chronic hypoxia, the increased pulmonary arterial pressure causes hypertrophy of the right ventricle, which must pump against an increased afterload. Fetal circulation is another example of global hypoxic vasoconstriction. Because the fetus does not breathe, PAO2 is much lower in the fetus than in the mother, producing vasoconstriction in the fetal lungs. This vasoconstriction increases pulmonary vascular resistance and, accordingly, decreases pulmonary blood flow to approximately 15% of the cardiac output. At birth, the neonate’s first breath increases PAO2 to 100 mm Hg, hypoxic vasoconstriction is reduced, pulmonary vascular resistance decreases, and pulmonary blood flow increases and eventually equals cardiac output of the left side of the heart (as in the adult). regulation of pulmonary blood flow Thromboxane A2 produce by macrophages, leukocytes and endothelium. cause vasoconstriction Other vasoactive subtances Prostacyclin (prostaglandin I2) and leukotrienes cause airway constriction Lung volume Pulmonary blood vessels include alveolar vessels (i.e., capillaries) that are surrounded by alveoli and extraalveolar vessels (e.g., arteries and veins) that are not. Increased lung volume affects the two types of vessels differently: It crushes the alveolar vessels, increasing their resistance, but it pulls open the extraalveolar vessels, decreasing their resistance. Because total pulmonary vascular resistance is the sum of alveolar and extra-alveolar resistances Pulmonary blood flow distribution of pulmonary blood flow At low lung volumes, the effect on extra-alveolar vessels dominates; as lung volume increases, the extraalveolar vessels are pulled open (as intrapleural pressure becomes more negative) and their resistance decreases At high lung volumes the effect on alveolar vessels dominates; as lung volume increases further, the alveolar vessels are crushed by the inflated alveoli and their resistance increases sharply. Subtopic 1 Subtopic 1 Right to left shunts Shunts Shunting of blood from the right heart to the left heart can occur if there is a defect in the wall between the right and left ventricles ! Left-to-right shunts. Left-to-right shunts are more common and do not cause hypoxemia. left to right shunts normal value for V/Q The normal value for V̇ /Q̇ is 0.8. distribution of V/Q in the lung regional variations in pulmonary blood flow, or perfusion, are caused by gravitational effects: Zone 1 has the lowest perfusion, and zone 3 the highest. Alveolar ventilation also varies in the same direction among the zones of the lung. Ventilation is lower in zone 1 and higher in zone 3, again due to gravitational effects in the upright lung Among the causes of left-to-right shunts are patent ductus arteriosus and traumatic injury. If blood is shunted from the left side of the heart to the right side of the heart, =>. In effect, oxygenated blood that has just returned from the lungs is added directly to the right heart without being delivered to the systemic tissues. => the PO2 in blood on the right side of the heart will be elevated. Ventilation/perfusion relationships ventilation/perfusions ratios Subtopic 1 Dead space Dead space is ventilation of lung regions that are not perfused. This ventilation is wasted, or “dead.” No gas exchange is possible in dead space because there is no blood flow to receive O2 from alveolar gas or add CO2 to alveolar gas. Dead space is illustrated by pulmonary embolism HIgh V/Q High V̇ /Q̇. Regions of high V̇ /Q̇ have high ventilation relative to perfusion, usually because blood flow is decreased. Unlike dead space, which has no perfusion, high V̇ /Q̇ regions have some blood flow. Because ventilation is high relative to perfusion, pulmonary capillary blood from these regions has a high PO2 and a low PCO2 Low V/Q Regions of low V̇ /Q̇ have low ventilation relative to perfusion, usually because ventilation is decreased. Unlike shunt, which has no ventilation, low V̇ /Q̇ regions have some ventilation. Because ventilation is low relative to perfusion, pulmonary capillary blood from these regions has a low PO2 and high PCO2. Ventilation/Perfusion defects Subtopic 1 Subtopic 1 Right to left shunt (V/Q=0) Right-to-left shunt is perfusion of lung regions that are not ventilated. No gas exchange is possible in regions of shunt because there is no ventilation to deliver O2 to the blood or carry away CO2 from the blood. Shunt is illustrated by airway obstruction and right-to-left cardiac shunts. Because no gas exchange can occur with a shunt, pulmonary capillary blood from these regions has the same composition as mixed venous blood: PaO2 is 40 mm Hg, and PaCO2 is 46 mm Hg Inspiratory center The inspiratory center is located in the dorsal respiratory group (DRG) of neurons and controls the basic rhythm for breathing by setting the frequency of inspiration. This group of neurons receives sensory input from peripheral chemoreceptors via the glossopharyngeal (cranial nerve [CN] IX) and vagus (CN X) nerves and from mechanoreceptors in the lung via the vagus nerve. The inspiratory center sends its motor output to the diaphragm via the phrenic nerve. Expiratory center located in the ventral respiratory neurons and is responsible primarily for expiration. Because expiration is normally a passive process, these neurons are inactive during quiet breathing. However, during exercise when expiration becomes active, this center is activated. medullary respiratory center brain stem control of breathing apneustic center Apneusis is an abnormal breathing pattern with prolonged inspiratory gasps, followed by brief expiratory movement. Stimulation of the apneustic center in the lower pons produces this breathing pattern in experimental subjects. Stimulation of these neurons apparently excites the inspiratory center in the medulla, prolonging the period of action potentials in the phrenic nerve, and thereby prolonging the contraction of the diaphragm. pneumotaxic center The pneumotaxic center turns off inspiration, limiting the burst of action potentials in the phrenic nerve. In effect, the pneumotaxic center, located in the upper pons, limits the size of the tidal volume, and secondarily, it regulates the respiratory rate. A normal breathing rhythm persists in the absence of this center. Commands from the cerebral cortex can temporarily override the automatic brain stem centers. cerebral cortex For example, a person can voluntarily hyperventilate (i.e., increase breathing frequency and volume). The consequence of hyperventilation is a decrease in PaCO2, which causes arterial pH to increase. hyperventilation is self-limiting, however, because the decrease in PaCO2 will produce unconsciousness and the person will revert to a normal breathing pattern. Although more difficult, a person may voluntarily hypoventilate (i.e., breath-holding). Hypoventilation causes a decrease in PaO2 and an increase in PaCO2, both of which are strong drives for ventilation. A period of prior hyperventilation can prolong the duration of breath-holding. control of breathing These chemoreceptors are located on the ventral surface of the medulla, near the point of exit of the glossopharyngeal (CN IX) and vagus (CN X) nerves and only a short distance from the DRG in the medulla. = > Thus central chemoreceptors communicate directly with the inspiratory center. central chemoreceptors The brain stem chemoreceptors are exquisitely sensitive to changes in the pH of cerebrospinal fluid (CSF). => Decreases in the pH of CSF produce increases in breathing rate (hyperventilation), and increases in the pH of CSF produce decreases in breathing rate (hypoventilation). The medullary chemoreceptors respond directly to changes in the pH of CSF and indirectly to changes in arterial PCO2 Mechanism 1. In the blood, CO2 combines reversibly with H2O to form H+ and HCO3 − by the familiar reactions. Because the blood-brain barrier is relatively impermeable to H+ and HCO3−, these ions are trapped in the vascular compartment and do not enter the brain. CO2, however, is quite permeable across the blood-brain barrier and enters the extracellular fluid of the brain. 2. CO2 also is permeable across the brain-CSF barrier and enters the CSF. 3. In the CSF, CO2 is converted to H+ and HCO3−. Thus increases in arterial PCO2 produce increases in the PCO2 of CSF, which results in an increase in H+ concentration of CSF (decrease in pH). 4 and 5. The central chemoreceptors are in close proximity to CSF and detect the decrease in pH. A decrease in pH then signals the inspiratory center to increase the breathing rate (hyperventilation). Subtopic 1 There are peripheral chemoreceptors for O2, CO2, and H+ in the carotid bodies located at the bifurcation of the common carotid arteries and in the aortic bodies above and below the aortic arch. Information about arterial PO2, PCO2, and pH is relayed to the DRG via CN IX and CN X, which orchestrates an appropriate change in breathing rate. Decreases in arterial PO2 The most important responsibility of the peripheral chemoreceptors is to detect changes in arterial PO2. Surprisingly, however, the peripheral chemoreceptors are relatively insensitive to changes in PO2: They respond dramatically when PO2 decreases to less than 60 mm Hg. - Thus if arterial PO2 is between 100 mm Hg and 60 mm Hg, the breathing rate is virtually constant. - However, if arterial PO2 is less than 60 mm Hg, the breathing rate increases in a steep and linear fashion. In this range of PO2, chemoreceptors are exquisitely sensitive to O2; Increase in arterial PCO2 The peripheral chemoreceptors also detect increases in PCO2, but the effect is less important than their response to decreases in PO2. Detection of changes in PCO2 by the peripheral chemoreceptors also is less important than detection of changes in PCO2 by the central chemoreceptors Decreases in arterial pH Decreases in arterial pH cause an increase in ventilation, mediated by peripheral chemoreceptors for H+. This effect is independent of changes in the arterial PCO2 and is mediated only by chemoreceptors in the carotid bodies (not by those in the aortic bodies). Thus metabolic acidosis, in which there is decreased arterial pH, the peripheral chemoreceptors are stimulated directly to increase the ventilation rate Lung strech receptors mechanoreceptors initiate a reflex decrease in breathing rate called the Hering-Breuer reflex. The reflex decreases breathing rate by prolonging expiratory time. Joint and muscle receptors Mechanoreceptors located in the joints and muscles detect the movement of limbs and instruct the inspiratory center to increase the breathing rate. Irritant receptors Irritant receptors for noxious chemicals and particles are located between epithelial cells lining the airways. Information from these receptors travels to the medulla via CN X and causes a reflex constriction of bronchial smooth muscle and an increase in breathing rate. J receptors J receptors. Juxtacapillary (J) receptors are located in the alveolar walls and therefore are near the capillaries. Engorgement of pulmonary capillaries with blood and increases in interstitial fluid volume may activate these receptors and produce an increase in the breathing rate. For example, in left-sided heart failure, blood “backs up” in the pulmonary circulation and J receptors mediate a change in breathing pattern, including rapid shallow breathing and dyspnea (difficulty in breathing). Remarkably, mean values for arterial PO2 and PCO2 do not change during exercise. An increased ventilation rate and increased efficiency of gas exchange ensure that there is neither a decrease in arterial PO2 nor an increase in arterial PCO2 chemoreceptors peripheral chemoreceptors Other receptors arterial Po2 and Pco2 Venous PCO2 Muscle and joint receptors send signals to the lung to hyperventilate cardiac output and pulmonary blood flow cardiac output of the right heart, pulmonary blood flow increases. There is a decrease in pulmonary resistance associated with perfusion of more pulmonary capillary beds, which also improves gas exchange. As a result, pulmonary blood flow becomes more evenly distributed throughout the lungs, and the V̇ /Q̇ ratio becomes more “even,” producing a decrease in the hysiologic dead space. O2 hemoglobin dissociation curve During exercise, the O2-hemoglobin dissociation curve shifts to the right (see Fig. 5.22). There are multiple reasons for this shift, including increased tissue PCO2, decreased tissue pH, and increased temperature hyperventilation High altitude decreases air partial pressure if alveolar PO2 is 60 mm Hg, then arterial blood will have a PO2 of 60 mm Hg, in which case the hypoxemia is severe enough to stimulate peripheral chemoreceptors in the carotid and aortic bodies => chemoreceptors instruct the medullary inspiratory center to increase the breathing rate. A consequence of the hyperventilation is that “extra” CO2 is expired by the lungs and arterial PCO2 decreases, producing respiratory alkalosis reponses to excercise integrative functions Summary Ascent to high altitude produces an increase in red blood cell concentration (polycythemia) and, as a consequence, an increase in hemoglobin concentration. Polycythemia viscosity. The increased concentration of red blood cells increases blood viscosity, which increases resistance to blood flow The stimulus for polycythemia is hypoxia, which increases the synthesis of erythropoietin (EPO) in the kidney. EPO acts on bone marrow to stimulate red blood cell production. Adaption to high altitude hyppoxemia and hypoxia 2,3-DPG and O2 hemoglobin dissocitaion curve concentration of 2,3-DPG causes the O2hemoglobin dissociation curve to shift to the right. This right shift is advantageous in the tissues because it is associated with increased P50, decreased affinity, and increased unloading of O2. pulmonary vasoconstriction At high altitude, alveolar gas has a low Po2, which has a direct vasoconstricting effect on the pulmonary vasculature (i.e., hypoxic vasoconstriction). As pulmonary vascular resistance increases, pulmonary arterial pressure also must increase to maintain a constant blood flow. The right ventricle must pump against this higher pulmonary arterial pressure and may hypertrophy in response to the increased afterload. acute altitude sickness The initial phase of ascent to high altitude is associated with a constellation of complaints including headache, fatigue, dizziness, nausea, palpitations, and insomnia. The symptoms are attributable to the initial hypoxia and respiratory alkalosis, which abate when the adaptive responses are established. Hypoxemia is a decrease in arterial Po2 hypoxia Is decreased O2 delivery to the tissue Summary hypotheisis