Sensory Systems Physiology Notes W16 PDF
Document Details
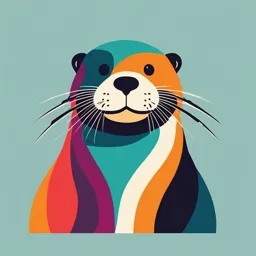
Uploaded by RobustPoisson
Tags
Summary
These notes cover sensory systems, including pain, touch, taste, smell, vision, and hearing in humans. They discuss the signals detected by each system, the peripheral receptors, afferent pathways, central processing, efferent pathways, and what happens when the sensory system is disrupted. The study notes also compare different characteristics of sensory receptors and cover the mechanisms of the muscle stretch reflex and the Golgi tendon organ reflex.
Full Transcript
**SENSORY SYSTEMS\_W16** For each modality we cover (pain, touch, taste, smell vision, hearing, and proprioception), think **SPACED**: - **S-** what **signals** does this system detect? - **P-** what are the **peripheral **receptors (cells [and] organs) that detect the signal and convert...
**SENSORY SYSTEMS\_W16** For each modality we cover (pain, touch, taste, smell vision, hearing, and proprioception), think **SPACED**: - **S-** what **signals** does this system detect? - **P-** what are the **peripheral **receptors (cells [and] organs) that detect the signal and convert it into a neural code? - **A-** what are the **afferent **pathways that carry the information to the central nervous system, including the primary, secondary, and tertiary neurons, whether and where the pathway crosses the midline, and the major collateral projections? - **C**- what is the **central **processing of the signal? How does the signal reach the spinal cord and brain, and what CNS areas are involved in processing the signal? - **E- **what **efferent** pathways modify detection of the signal? - **D-** what happens when the system is **disrupted**? Does the pathway degrade with age? **1. Describe the purpose of sensory input** Sensory input allows organisms to perceive and interact with their environment. It is essential for survival, providing information about potential dangers, food sources, and social interactions. Sensory information helps maintain homeostasis by monitoring internal and external conditions. **2. Compare and contrast the different characteristics of sensory receptors** **Characteristics of Sensory Receptors:** **Modality Specificity:** Different receptors are specialized for specific types of stimuli (e.g., mechanoreceptors for touch, photoreceptors for light). **Receptive Field Size:** The area over which a receptor can detect stimuli. Smaller receptive fields allow for finer discrimination (e.g., fingertips vs. back). **Adaptation:** Receptors can be classified as rapidly adapting (phasic) or slowly adapting (tonic), influencing how they respond to continuous stimuli. **Conduction Velocity:** Myelinated fibers (A-alpha, A-beta) conduct signals faster than unmyelinated fibers (C fibers), affecting the speed of sensory information transmission. **3. Understand the organization of the gustatory (taste) and olfactory (smell) systems** **Gustatory System:** Taste receptors are located on taste buds within papillae on the tongue. Five basic tastes: sweet, sour, bitter, salty, umami. Taste information is transmitted via cranial nerves VII (facial), IX (glossopharyngeal), and X (vagus) to the nucleus of the solitary tract, then to the thalamus, and finally to the gustatory cortex. **Olfactory System:** Olfactory receptors are located in the olfactory epithelium in the nasal cavity. Each receptor neuron has cilia that bind odorant molecules. Olfactory information is transmitted via the olfactory bulb to the olfactory cortex, bypassing the thalamus initially. Olfactory projections reach the amygdala, piriform cortex, and entorhinal cortex, linking smell to memory and emotion. **4. Identify the major types of receptors for the chemical (olfactory and gustatory) senses** **Gustatory Receptors:** Type I cells: Glial-like cells, potentially involved in salty taste. Type II cells: Receptors for sweet, umami, and bitter tastes, use G-protein-coupled receptors. Type III cells: Receptors for sour taste, use ion channels. **Olfactory Receptors:** Bipolar neurons with cilia containing receptor proteins. About 350 functional olfactory receptor genes in humans, allowing detection of a wide range of odorants. **5. Recognize how information is transformed in the afferent pathways between receptor and brain** **Transformation of Sensory Information:** **Primary Afferent Neurons:** Carry information from receptors to the spinal cord or brainstem. **Secondary Neurons:** Transmit signals from the spinal cord or brainstem to the thalamus. **Tertiary Neurons:** Relay information from the thalamus to the appropriate sensory cortex (somatosensory, gustatory, olfactory). **6. Differentiate the different types of afferent fibers in the somatosensory system** **Types of Afferent Fibers:** **A-alpha Fibers:** Large diameter, myelinated, fast conduction, involved in proprioception and muscle sense. **A-beta Fibers:** Medium diameter, myelinated, conduct fine touch and pressure. **A-delta Fibers:** Small diameter, lightly myelinated, conduct sharp pain and temperature. **C Fibers:** Small diameter, unmyelinated, conduct dull pain, temperature, and crude touch. **7. Differentiate between discriminative and non-discriminative touch sensations** **Discriminative Touch:** Involves fine, precise touch information. Carried by A-beta fibers. Ascends via the dorsal column-medial lemniscus pathway to the somatosensory cortex. **Non-Discriminative Touch:** Involves crude touch, pain, and temperature. Carried by A-delta and C fibers. Ascends via the spinothalamic tract to the somatosensory cortex. **8. Understand how conduction velocity affects sensation of stimuli at the skin surface** **Conduction Velocity and Sensation:** **Myelination and Diameter:** Larger diameter and myelinated fibers (A-alpha, A-beta) conduct signals faster, resulting in quicker sensation. **Unmyelinated Fibers:** C fibers conduct more slowly, leading to delayed sensation of dull pain and temperature changes. **9. Describe the mechanisms of the muscle stretch reflex (tendon reflex) and the golgi tendon organ reflex** **Muscle Stretch Reflex:** Involves muscle spindles detecting stretch. Afferent signals sent via A-alpha fibers to the spinal cord. Efferent signals via alpha motor neurons cause muscle contraction. **Golgi Tendon Organ Reflex:** Detects tension in tendons. Afferent signals sent via A-alpha fibers to the spinal cord. Inhibitory interneurons reduce muscle contraction to prevent damage. **10. Describe the main discriminative ascending pathways from sensory receptor to cortex** **Discriminative Ascending Pathways:** **Dorsal Column-Medial Lemniscus Pathway:** Carries fine touch and proprioception. Ascends ipsilaterally in the dorsal columns, decussates in the medulla, then projects to the thalamus and somatosensory cortex. **11. Describe the role of the thalamus in sensory processing** **Role of the Thalamus:** Acts as a relay station for sensory information. Filters and modulates signals before transmitting them to the cortex. Involved in sensory integration and attention. **12. Identify the parts of the cortex involved and describe their roles in somatosensory processing** **Parts of the Cortex:** **Primary Somatosensory Cortex (S1):** Receives and processes sensory information from the body. **Secondary Somatosensory Cortex (S2):** Further processes sensory information and integrates it with other modalities. **13. Apply knowledge of sensory systems to clinical conditions associated with lesions of the ascending pathways** **Clinical Conditions:** **Lesions in the Dorsal Column-Medial Lemniscus Pathway:** Result in loss of fine touch and proprioception on the [ipsilateral] side. **Lesions in the Spinothalamic Tract:** Result in loss of pain and temperature sensation on the [contralateral] side. **Thalamic Lesions:** Can lead to sensory deficits and pain syndromes. **Cortical Lesions:** Result in impaired sensory perception and integration, such as astereognosis (inability to recognize objects by touch). **LO1. Describe the purpose of sensory input** Sensory input allows organisms to perceive and interact with their environment. It is essential for survival, providing information about potential dangers, food sources, and social interactions. Sensory information helps maintain homeostasis by monitoring internal and external conditions. **Discriminative Somatic Sensation** Discriminative somatic sensation refers to sensations of fine touch and joint proprioception. **Somatosensory Homunculus:** A 3D representation of sensory information distribution on the skin.\ \ **Areas with high sensitivity:** Hands (especially fingertips), lips, tongue, and lower face. **Areas with low sensitivity:** Torso, shoulders. **TYPES OF TOUCH:** **Discriminative Touch (Fine Touch):** **Includes:** Precise touch information (especially on the non-hairy surface of the hands and feet and along the feet) and proprioception (joint position and movement). **Receptors:** Low-threshold mechanoreceptors. **Nerve Fibers:** Densely myelinated, thick, fast-conducting (A-alpha and A-beta fibres). **Pathway:** Ascending fibres concentrated in the Dorsal column-medial lemniscus system. **Non-Discriminative Touch (Crude Touch):** **Includes:** Pain, temperature (hot/cold), and light touch (affective/emotional that is not restricted in terms of space or pressure). **Receptors:** Free nerve endings. **Nerve Fibers:** Lightly myelinated or unmyelinated, **slow-conducting** (A-delta and C-fibres). **Pathway:** Ascending fibres concentrated in the spinothalamic tract. **3. Pathways** **Discriminative Pathway (Fine Touch):** **Primary Afferents:** A-alpha and A-beta fibres. **Course:** Enter spinal cord, ascend ipsilaterally to the medulla. **Synapse:** On second-order neurons in the medulla, cross midline, ascend to thalamus where they synapse on third-order thalamocortical neurons which project to primary somatosensory cortex. **Non-Discriminative Pathway (Crude Touch):** **Primary Afferents:** A-delta (lightly myelinated) and C-fibres (unmyelinated) **Course:** Enter spinal cord, synapse on second-order neurons in gray matter either at the same level that they enter the spinal cord or one-two levels above or below it. The second order neurons then cross the midline, and ascend to thalamus, synapse on third-order neurons, and project to the cerebral cortex. **KEY DIFFERENCES:\ **-Where the pathways cross the mid-line and where the second-order motor neuron cell bodies are located. **4. Fiber Types**  - **A-alpha Fibers:** Large diameter meaning low internal resistance meaning they carry information along quite quickly, thick myelination, fast conduction, uses Saltatory conduction. **A-beta Fibers:** Slightly narrower than A-alpha, strong myelination, rapid conduction. **A-delta Fibers:** Narrower, lightly myelinated, slow conduction. **C-fibres:** Smallest diameter meaning high internal resistance and limited conduction capacity along its length, unmyelinated, very slow conduction. No saltatory conduction. **Proprioception as the first element of discriminative touch that we will discuss:\ ** **Definition:** Ability to sense limb and body position in space. This is a function of the angle of the joint and the amount of stretch or load on a given muscle. **Proprioception Receptors:** Muscle spindles (embedded in skeletal muscles). Muscle spindles act as sensors for determining 'how stretched a muscle is' OR 'the overall muscle length'. Intrafusal muscle fibres are contained within the muscle spindle, and they have three segments: -**A central non-contractile portion** bounded by a contractile muscle portion on either side of the non-contractile centre. In the centre, there is a large 1A or A-alpha sensory fibre that terminates in what are called annulo-spiral endings. The annulo-spiral endings wrap around the intrafusal muscle fibre, cling very tightly to it, and act as the mechanoreceptors for deformation or stretch of the muscle fibre. -**The contractile portions on either end** receive innervation from gamma motor fibres. The gamma motor fibres are narrower, have a smaller myelination, and proportionately conduct much more slowly than the alpha motor neurons. **-The large extrafusal skeletal muscle** fibres are innervated by alpha motor neurons (large conducting or large myelinated fast conducting motor neurons). Therefore, the fusimotor systems sets the "gain" of the motor system. **Intrafusal Fibre Types:** Nuclear chain fibres and nuclear bag fibres. **Innervation:** **Central Non-Contractile Portion:** A-alpha sensory fibers (annulo-spiral endings). **Contractile Portions:** Gamma motor fibers. In the extrafusal muscle fibres, there are alpha motor neurons. In the contractile portion of the intrafusal fibres, there are gamma motor neurons. **Function:** Encode muscle stretch and length, providing proprioceptive feedback.  \ If we look at the membrane that forms the terminus of the nerve fibre, you will see ion channels connected together by a web of cytoskeletal strands. These strands are actin fibers which connecting the individual channels within the membrane. The actin cytoskeleton web allows deformation of the membrane to pull on the sides of each channel and resultingly open the channels. This results in a graded receptor potential. On the bottom graph, B, you can see the stretch of muscle fibres (in micrometers), and in B, there is a proportional change in graded potential to the membrane associated with each stretch. As long as the membrane is deformed, there will be current flowing through these channels. When the deformation is removed, the current returns to its normal baseline level. Overall, the muscle spindle is sensitive to muscle stretch; it does not add any contractile force to the muscle but instead responds to changes in muscle length, either through contraction or when the muscle is loaded.  As the muscle length changes, there is an increase in discharge of this somatosensory or sensory fibre that is wrapping around the interfusal fibres. The sensory fibre is active throughout the dynamic phase of the change in muscle length, and then it returns to a steady state, and there is a return to a normal firing pattern. Without the gamma motor system (without this efferent contractile portion of the motor fibre) you could not encode stretch of the muscle throughout its length. If we stimulate the alpha motor neuron and drive a contraction in this muscle, we simultaneously cause stimulation of the gamma motor neuron, which results in the contractile portions of the interfusal fibre contracting and allows for the sensory portion of the muscle spindle to encode the muscle length throughout this contraction. **CUTANEOUS MECHANOSENSATION OR SKIN SENSATION:** **Mechanoreceptors in the Skin** **Non-Discriminative Receptors:** **Free Nerve Endings:** Respond to pain and temperature. **Hair Follicle Receptors:** Respond to deformation of hair. **4** **Discriminative Receptors (Low-Threshold Cutaneous Mechanoreceptors carrying fine touch information):** **Meissner's Corpuscle:** Small receptive field, fast adapting. **Merkel's Disc:** Small receptive field, slow adapting. **Ruffini Ending:** Large receptive field, slow adapting, embedded in the deep layers of the skin. **Pacinian Corpuscle:** Large receptive field, fast adapting, embedded in the deep layers of the skin. Importantly, these low-threshold cutaneous mechanoreceptors take mechanical energy/stimulus and transform it into action potentials. **Characterising the Low-Threshold Cutaneous Mechanoreceptors:** Uses three characteristics: receptive field size, adaptation and innervation density. **Receptive Field Size:** the area of skin surfaced that when changed or pressed or stimulated, will activate a response in mechanoreceptors. **Small Receptive Field Size:** Meissner's corpuscle, Merkel's disc. **Large Receptive Field Size:** Ruffini ending, Pacinian corpuscle. **Adaptation:** **Fast Adapting (do not encode the entire phase of a stimulation, are responsive at the beginning of a stimulation, rapidly adjust to the steady state and then they respond at the end of the stimulation):** Meissner's corpuscle, Pacinian corpuscle. ** Slow Adapting (encode the change in pressure on the skin throughout the phase of stimulation, leading to a steady-state response):** Merkel's disc, Ruffini ending. **Innervation Density:** ** High Density:** Meissner's corpuscle, Merkel's disc (especially in fingertips,\ palmar surfaces). ** Low Density:** Ruffini ending, Pacinian corpuscle (one or two will encode the entire surface of larger areas like the hand). \ \ **USING THE EXAMPLE OF THE PACINIAN CORPUSCLE:** - Fast adapting receptor - If a steady stimulus is applied, and the corpuscle is depressed, an on-off response occurs. - The on-off response is encoded by connective tissue laminae/lamellae around the free nerve ending, which leads to an Alpha-beta type fibre. When the stimulus is applied and the onion-like structure is deformed, fluid gets squeezed onto these membranes and these membranes then deform the ion channels in the free nerve ending. This allows the nerve to encode, as action potentials, the degree of deformation of this particular structure. - If a changing stimulus is applied, such as vibration, there will be a response to each cycle of the vibration, which allows the low threshold mechanoreceptors to encode not just the presence or absence of the stimulus, but whether or not there is a vibratory component. Vibration is information about whether or not a particular object is living or moving and this information is useful in terms of survival for the organism in contact with the external world. Low threshold mechanoreceptors are much more common in the skin of the hands and feet and of the lips and tongue than they are of the body surface or the upper legs or upper arms and so on. This can be measured through use of two-point discrimination. In the thumb and lips, two-point discrimination, is quite precise. In the case of thigh, upper back, etc, stimuli that are closer than 40mm are interpreted as a single point or pressure source. **8. Central Nervous System Pathways** The red line shows the system for fine touch. The blue line shows the system for crude touch. **Fine Touch (Discriminative Touch) System:\ ** **Primary Afferent Pathway:** Dorsal column nuclei in the medulla, cross midline, ascend via medial lemniscus to the thalamus. **Thalamic Relay:** VPM (face and head) and VPL (body surface).  **THE CNS PATHWAY:** Ascending white matter tracts that carry the fine touch information are called the dorsal columns (enlargements on the dorsal surface of the spinal cord) terminate in the dorsal column nuclei of the medulla. There are two dorsal columns. There\'s the **gracile funiculus** and the **cuneate funiculus.** The gracile funiculus is the more medial one. The cuneate is the more lateral one. The gracile carries information from the lower limbs, and the cuneate carries information primarily from fine touch receptors on the upper limbs. The second-order neurons that project to the thalamus are **generally** contralateral in both fine touch and crude touch. Second-order neurons cross at what are called decussations or crossing points where the fibers cross the midline. At the level of the thalamus in the VPM, the ventral posterior medial, and VPL, ventral posterior lateral thalamus, information from these second-order neurons synapses onto third-order neurons, which project to the somatosensory cortex. The VPM carries information from the face and head, whereas the VPL carries information from the body surface. **CONSIDERING THE SPINAL CORD AND ITS STRUCTURES:** The spinal cord is composed of white matter and grey matter tracts that extend along the entire vertebral column.\ \ In the case of the spinal nerves, which exit the spinal cord in the dorsal part and the ventral part and form spinal nerves that project out to the various receptors and muscular targets, we have the dorsal portion above and the ventral portion below in the diagram. The dorsal portion that forms the spinal nerve has these enlargements called the **dorsal root ganglia** which is where the cell bodies of A-alpha and A-beta fibres can be found. These A-alpha and A-beta fibres are the ones that carry proprioceptive and fine touch mechanoreception information. These neurons have long axons -- they extend from the receptor in the periphery, all the way up to the cell body here, before bypassing the cell body. Thus, they form continuous axons that go up into the dorsal columns and ascend to the level of the medulla. These axons can be quite large and often meters long.\ \ Note that sensory axons also have collaterals which terminate in the dorsal gray matter of the spinal cord (purple dotted lines in the diagram above). These are axon collaterals from the same axon that allow information about muscle stretch in particular to be represented at the level of the spinal cord while the main axon then projects onto the brain and the medulla and brain. **HISTOLOGY OF THE DORSAL COLUMNS:**  The **gracile funiculus** or **gracile dorsal column** extends from the fifth sacral vertebrae to the seventh thoracic vertebra, carrying information from the legs and feet. The arrangement of information from the fine touch receptors in the hands and feet is such that the feet is medial, the hands are lateral. The way to remember this is that we walk on grass, so the gracile funiculus is more medial and carries information from the lower limbs. At C5, there is the cuneate funiculus and gracile funiculus. At L2, only the gracile funiculus is present because we are now below the level of the upper limb and that\'s where the cuneate information terminates. Thus, the spinal cord morphology changes along its length as a function of the presence or absence of information from the dorsal columns. **MEDIAL LEMNISCUS:** **First-Order Neurons**: First-order afferent neurons carry fine touch and proprioception information from the peripheral nervous system to the central nervous system. The first-order neurons' axons travel through the spinal cord in the **dorsal columns**. **Second-Order Neurons**: Second-order neurons are located in the **dorsal column nuclei** (specifically, the gracile nucleus and the cuneate nucleus) in the medulla oblongata. First-order neurons terminate on the second-order neurons. At this point, the pathway changes its name from the **dorsal columns** **pathway** to the **medial lemniscus pathway**. The term "medial lemniscus" refers to the same fibre bundle, but it is now running along the medial portion of the medulla and through the pons. **Crossing the Midline**: The fibers of the medial lemniscus **cross the midline** (decussate) at the level of the medulla. This means that the sensory information from the right side of the body is processed by the left side of the brain, and vice versa. 4\. **Third-Order Neurons**: - The medial lemniscus fibers terminate on the third-order neurons located in the **thalamus**. The third-order neurons for fine touch information and the third-order neurons for crude touch information originate in the **VPM** **and the VPL of the thalamus.** - The thalamus is the relay nucleus for sensory information before it reaches the cerebral cortex. Information from the thalamus is projected to primary somatosensory cortex in the cerebral cortex.  **Crude Touch (pain and temperature) System:** **Primary Afferent Pathway:** Synapse at spinal cord level, cross midline, ascend to thalamus. **Thalamic Relay:** VPM and VPL. **CNS STRUCTURES AND SENSORY PATHWAYS, WITH REFERENCE TO THE THALAMUS AND CORTEX:** 1. **Thalamus and Ventricles**: The **thalamus** is a relay station for sensory information. - **VPM (ventral posteromedial nucleus)**: Processes sensory information from the face. - **VPL (ventral posterolateral nucleus)**: Processes sensory information from the rest of the body. - The **third ventricle** and **lateral ventricles** are fluid-filled cavities in the brain. 2\. **Primary Somatosensory Cortex**: Located in the **post-central gyrus**, just behind the **central sulcus**. The **central sulcus** is a prominent groove that runs from the middle of the brain (medial) to the side (lateral). This area contains the cell bodies for the primary somatosensory cortex, which processes sensory information from the body. 3\. **Internal Capsule**: A bundle of white matter fibers that carries information between the thalamus and the cortex. **Mapping the Body on the Brain (Homunculus)** 1\. **Somatotopic Map**: The primary somatosensory cortex is organized as a map of the body, called the **homunculus**. **Medial portion**: Represents the legs and feet. **Central portion**: Represents the arm and hand. **Lateral portion**: Represents the face and oropharynx (tongue and roof of the mouth). 2\. **In Vivo Intercortical Recordings**: By using electrodes to stimulate or record activity in different parts of the cortex, researchers can determine which body part is represented in each region. **Medial stimulation**: Activates neurons corresponding to the legs. **Central stimulation**: Activates neurons corresponding to the mouth. **Lateral stimulation**: Activates neurons corresponding to the oropharynx. **Dynamic Nature of the Homunculus** 1\. **Plasticity of the Somatosensory Cortex**: The homunculus is not static; it can change throughout life. The homunculus is a dynamic representation from the body. If sensory input from a body part is lost (e.g., due to injury or amputation), adjacent areas in the brain can take over the representation of that body part. **Example:** If the hand's sensory input is lost, the brain regions that represent the face and other adjacent areas may expand to cover the hand's representation. 2\. **Phantom Limb Sensation**: After losing a limb, individuals may still feel sensations in the missing limb, known as **phantom limb sensation**. This occurs because the brain's representation of the limb still exists and can be activated by stimulating adjacent areas on the cortex. It is not a perfect expansion -- in the case of the reorganised system, there will be sensation associated with the fingers and hands along the face even though the forelimb has been de-afferented. This can be described as residual sensation. Example: Stimulating the face in someone who has lost a hand may cause them to feel sensations as though they are being felt by the hand even though the hand is missing. 3\. **Mirror Box Therapy**: **Phantom limb pain**: Patients may feel pain or discomfort in a missing limb. **Mirror box therapy**: A mirror is used to create a visual illusion of the missing limb. By moving their intact hand while looking at its reflection, patients can feel like they are moving their missing limb, which can reduce pain and discomfort. 4\. **Rubber Hand Illusion**: If a person sees a fake hand being stroked while their own hand is simultaneously stroked but hidden, they can start to feel that the fake hand is part of their body. This shows how the brain can integrate visual and tactile information to update the body map. 5\. **Illusory Third Arm**: When a fake hand is convincingly integrated into a person's body map, they may react to threats to the fake hand as if it were their own. This reaction disappears if the fake limb is placed in an unnatural position (e.g., a foot instead of a hand). **Summary** **Thalamus**: Processes sensory information (VPM for face, VPL for body). **Ventricles**: Fluid-filled cavities (third and lateral ventricles). **Primary somatosensory cortex**: Located in the post-central gyrus, organized as a body map (homunculus). **Central sulcus**: Landmark dividing the frontal and parietal lobes. **Internal capsule**: White matter connecting thalamus and cortex. **Homunculus**: A dynamic, plastic map of the body in the brain. Representation of body parts can change based on sensory input. **Phantom limb sensation**: Sensations in missing limbs due to reorganization of the brain's sensory map. **Mirror box therapy**: Visual feedback can help reduce phantom limb pain. **Rubber hand illusion**: The brain can adopt a fake hand into its body map.  **Illusory third arm**: Shows the brain's ability to update the body map in real time. **REFLEXES\_VIDEO 2\_W16:** **Major Clinical Reflexes tested using information from the proprioceptive system** 1\. **Myotactic (Stretch) Reflexes**: **Examples**: [Patellar tendon reflex] and ankle tendon reflex. **Characteristics**: IMPORTANT TO KNOW **Monosynaptic**: Involves only one synapse between the sensory neuron and the motor neuron in the spinal cord. **Autogenic**: The muscle that is stretched (stimulated) is the same muscle that contracts. **Ipsilateral**: The reflex action occurs on the same side of the body that is stimulated by the reflex hammer. **Mechanism** of the patellar tendon reflex: **1.** **Stimulus**: Tapping the patella with a reflex hammer stretches the quadriceps muscle. **2.** **Muscle Spindle Activation**: When the muscle stretches, the muscle spindle detects changes in muscle length and is activated. The muscle spindle sends a signal via **type 1A** sensory neurons to the spinal cord. **3. Signal Pathway**: The sensory neuron splits (collateralizes). One (main) part goes to the medulla, specifically to the gracile nucleus in the case of the patellar tendon reflex (which is activated from the lower limb). The (collateral) other part of the axon synapses directly onto an alpha motor neuron in the anterior horn grey matter of the spinal cord. 4\. **Motor Response**: The alpha motor neuron sends a signal back to the quadriceps muscle, causing it to contract (resulting in the leg kick). 5\. **Important Points**: The alpha motor neuron does not involve the gamma motor neuron system in this reflex. There is no need for a spinal cord interneuron for this reflex. **THINGS TO NOTE:** Type 1A sensory axon is the first instance that we\'ve seen of axon collateralization. It is capable of synapsing at multiple places, even though there\'s still only a single fibre coming out of the muscle spindle.\ \ In this reflex, the antagonist muscle, in this case, the hamstring, may be inhibited by a very powerful reflexive response. 2. **Golgi Tendon Organ Reflex**: responds to muscle load **Characteristics**: **Polysynaptic**: Involves multiple synapses including an interneuron in the spinal cord. **Heterogenic**: The response can occur in **multiple** muscles. **Ipsilateral**: The reflex action occurs on the same side of the body as the stimulus. **Mechanism**: 1\. **Stimulus**: [Overloading] the muscle, which activates the Golgi tendon organ. 2\. **Golgi Tendon Organ Activation**: The Golgi tendon organ, located at the muscle-tendon junction, sends a signal via **type 1B** sensory neurons to the spinal cord. 3\. **Signal Pathway**: The sensory neuron collateralizes and synapses onto an **inhibitory interneuron** in the spinal cord. 4\. **Motor Response**: The inhibitory interneuron suppresses the activation of the alpha motor neuron, stopping the contraction of the stimulated muscle. There is also a projection to the antagonist muscle, facilitating its contraction to control the load. The Golgi Tendon Organ Reflex protects the muscle from application of too much force. When the load is too great, the muscle will **STOP** contracting and in this muscle reflex, the antagonist muscles are activated. Therefore, the receptor is the Golgi Tendon Organ located in the interface between the muscle and the tendon. **\ \ \ \ \ \ \ \ \ \ \ \ \ ** **TASTE AND SMELL: THE CHEMICAL SENSES\_W16** **Taste System (Gustatory System)** **Basic Taste Sensations** **Five Basic Tastes**: 1\. **Sweet**: Indicates energy-rich nutrients (carbohydrates and proteins). Sweet is affectively pleasant as we are driven to take in foods that are calorically dense. 2\. **Sour**: Detects acidity, often indicating spoiled or unripe food. 3\. **Salty**: Essential for maintaining osmolality and electrolyte balance. 4\. **Bitter**: Generally an aversive stimulus, warning against potential toxins. 5\. **Umami**: Indicates protein-rich food, especially from amino acids like glutamate. Umami is driven by the presence of monosodium glutamate, giving the sensation of meatiness and savouriness experienced from read meats and fermented foods. At low concentration, sour and bitter can be pleasant BUT at high concentration, sour and bitter are unpleasant and capable of inducing nausea and vomiting. The unpleasantness of highly bitter foods saves us from intoxication. The sourness of highly sour foods saves us from eating unripe fruits or spoiled foods. **Saliva's Role in mouth function and taste perception**: Solubilises food compounds. Distributes tastants across the taste receptors in the mouth. Facilitates swallowing and mouthfeel. **Taste Receptors and Papillae** Taste sensations are widely distributed across the tongue and the roof and back of the mouth. Papillae of the tongue are small, nipple-like projections on the surface of the tongue that have various functions, primarily related to taste and texture sensation. **Types of Papillae**: 1\. **Filiform**: Most numerous, no taste buds. 2\. **Fungiform**: Mushroom-shaped, scattered across the tongue, contain taste buds. 3\. **Circumvallate**: Large, located at the back of the tongue, contain taste buds. 4\. **Foliate**: Located on the sides of the tongue, contain taste buds. **Taste Buds**: - Onion-like organs consisting of 50 to 150 taste receptor cells. Taste buds are located - The taste buds are located on the sides of the circumvallate and foliate papillae. The - The filiform papillae do not contain any taste buds. The filiform papillae is the most - Contain 50-150 taste receptor cells. - Taste cells have microvilli that extend into the taste pore.  **TASTE CELL STRUCTURES:** Taste cells are elongated cells with two poles, making them BI-POLAR cells. One pole reaches the surface of the tongue by an opening in the taste bud called the taste pore. Small processes, called microvilli, extend from the surface of the taste cell and are exposed to tastants in the saliva on the tongue. It is here that sensory transduction takes place. At the opponent pole, taste cells transmit their information through synaptic contacts to innervating nerves that convey the information to the brain. Taste cells secrete ATP as a primary signal molecule and neurotransmitter. Taste receptors are quite frequently replaced. Therefore, each taste bud has a population of stem cells associated with it to allow new receptors to form on the epithelium. **TRANSMISSION PROCESS WITHIN TASTE CELLS:** 1. Food is consumed. 2. Tastant is solubilised and exposed to the microvilli of the taste cell. 3. The taste cell undergoes depolarisation which spreads along the cell body and There are **three** major types of taste cells. The receptors expressed on each type of taste cell gives rise to the different sensations that each taste cell type can elicit. **Types of Taste Cells**: 1. **Type I (Glial-like)**: Partly responsible for the salty taste through ion channels that process sodium directly.\ -Include K+ channels that allow K+ secretion into the extracellular space. -NTPDase2, a membrane-expressed ATPase that allows processing of secreted ATP as a transmitter signal between cells. 2. **Type II (Receptor Cells)**: Most common type. Detect sweet, bitter, and umami tastes through G-protein coupled receptors.\ -Panx family of receptors form membrane channels which allow ATP to be secreted into the extracellular space. Main means by which ATP is released into the extracellular environment. -Receptors on the membrane surface that are themselves sensitive to ATP (P2X and P2Y) type proteins. -TRP (transient receptor type channels play a big role in sensing environment signals throughout the body). -Internal depolarisation of the cell via calcium. In the Type II cells, calcium stimulates ATP release. **3.** **Type III (Presynaptic Cells)**: Most neuronal-like in that their activation of sensory neurons is directly through transmitters. Release neurotransmitters like serotonin, CCK and NPY in response to sour tastants. -Internal, direct depolarisation of the cell via calcium AND depolarisation of the membrane via ATP secreted from Type II cells. -In the Type III cells, calcium stimulates neurotransmitter release in concert with the activation of the P2Y channels which are responsive to the ATP that is secreted by the Type 2 cells. **Transduction Pathways for tastant types:** **Salty and Sour**: Use direct ion channels. In the case of salty sensation, it is thought that this sensation is mediated via direct activation of a sodium channel. In the case of the sour taste receptor, it is an ion channel permissive for both sodium and hydrogen ions that permits the sour sensation. **Sweet, Bitter, Umami**: Use G-protein coupled receptors, leading to cascade involving IP3 and calcium release. In the case of sweet and umami, the proteins expressed at the extracellular surface of the membrane are responsible for the different sensations, and these are a function of the combination of the T1R1-3 types of protein domains.\ \ -In the case of the sweet, a combination of the R2 and R3 subunits facilitate sweet sensation, and therefore when this G-protein system is activated, the sweet sensation is elicited. -In the case of umami, the combination of these extracellular surface proteins are slightly different in that there are T1R1 and T1R3 extracellular membrane domains expressed, and as a result, when this particular G-protein system is activated, the umami sensation is elicited. **DOWNSTREAM 2^nd^ MESSENEGER CHEMICAL PATHWAY:** **G-Protein-Mediated Taste Pathway** 1\. **Activation of G-Protein-Coupled Receptor (GPCR):** A tastant binds to a GPCR on the taste cell membrane. This activates the G-protein, causing its subunits (alpha, beta, gamma) to interact with phospholipase C beta 2 (PLCβ2) in the PLCβ2 pathway. 2\. **PLCβ2 Pathway:** PLCβ2 converts PIP2 into diacylglycerol (DAG) and inositol triphosphate (IP3). IP3 levels increase, leading to the activation of the ryanodine receptor and the release of intracellular calcium stores. 3\. **Calcium-Mediated Channel Activation:** The rise in intracellular calcium activates the TRPM5 channel (additional channels associated with the taste). This also opens the pannexin-1 channel (the ATP secreting channel), allowing ATP to be released into the extracellular environment. 4\. **ATP Release and Stimulation:** Released ATP can stimulate the same type 2 receptor or adjacent type 1 and type 3 receptors as well. This cascade is crucial for generating the taste sensation.  **Ion Channel Mechanisms for Salt and Sour Tastes: simple ion mechanisms** 1\. **Sour Taste Mechanism:** Sour tastants (e.g., vinegar, citric acid) provide hydrogen ions (H+). H+ binds to proton-sensitive channels, causing them to open and allow sodium ions (Na+) to enter, resulting in depolarization. 2\. **Salt Taste Mechanism:** Salt tastants open ENaC (epithelial sodium channels), permitting Na+ entry through the membrane. The increase in sodium results in local depolarisation. High salt concentrations may open other, less well-understood channels. Not yet clear what the other channel is. A diagram of a cell Description automatically generated **\ Sensory Pathway to the Brain** 1\. **Transmission to Central Nervous System:** Once the afferent neurons are stimulated, the taste information is carried by three cranial nerves VII (facial), IX (glossopharyngeal), and X (vagus) to the nucleus of the solitary tract (NTS) in the brainstem. VII and IX encode taste information gathered along the dorsal surface and underside of the tongue. X encodes taste sensation that are received at the back of the mouth and the pharynx. All three cranial nerves converge onto the NTS which has a horseshoe shaped gustatory area that is located directly underneath the cerebellum. From the NTS, signals by second-order neurons are relayed to the ventral posterior medial nucleus of the thalamus and then to the gustatory cortex (near the anterior insula and frontal operculum in the region shown in purple on the side-view of the brain). This is underneath the lateral fissure so this region is behind the temporal lobe. 2\. **Information Transformation at each step along the pathway:** In the NTS, neurons can respond to a wide variety of stimulants, including water, salt, bitter (quinine), sweet (glucose), and acidic solutions. Different stimulants result in different responses. At the gustatory cortex (orbitofrontal cortex), this information is transformed. Some neurons are more specific for specific tastants. Therefore, in the graph shown below, the response to some stimuli is eliminated at the level of the gustatory cortex. This transformation allows for degrees of specificity in the responses and sensations expressed at the neocortex. **Smell System (Olfactory System)** **Role of Smell in Taste** Smell is encoded through receptors in the back of the nose and oral cavity (retronasal olfaction), significantly enhancing taste perception.\ \  **Smell and Taste Interaction** **Flavour Perception:** Combination of taste and smell. Olfactory (smell) and gustatory (taste) senses interact to create the perception of flavour. **Olfactory Anatomy** 1\. **Olfactory Epithelium and Bulb:** Located at the back of the nose, on the cribriform plate. Olfactory bulb contains neurons forming the olfactory tract\ (cranial nerve I). 2\. **Anatomy of the Nasal Cavity:** Contains structures called Turbinates. Turbinates create airflow turbulence to expose odorants to the olfactory epithelium. The Turbinates direct airflow across the ethmoid bone and expose the odorants to the olfactory epithelium. Turbinates also secondarily humidify and warm the air that is going to the lungs. **Olfactory Receptors** 1. **Structure:** Olfactory receptors are true neurons with bipolar structure. Olfactory receptors send a single dendrite to the surface of the epithelium, where it ends in a knob. The knob gives rise to several fine processes, the olfactory cilia. The cilia are embedded in the mucus and cover the epithelium with a dense meshwork. The cilia are the site of sensory transduction. At the basal pole, olfactory neurons form an axon. Up to 1,000 axons are clustered in bundles that leave the nasal cavity through a thin bone to the enter the **adjacent olfactory** bulb, a phylogenetically old region of the brain.\ \ \ Dendrites branch into cilia within the mucus layer, where odorant transduction occurs. The cilia form Axons bundle and pass through the cribriform plate to form cranial nerve I. 2. **Regeneration:** Like taste cells, olfactory neurons are being generated and replaced throughout life. This means that every done, some 100,000 axons have to find their way from the nasal cavity to the bulb and have to establish new synaptic contacts with their target neurons. Overall, olfactory neurons regenerate throughout life. Regeneration is a focus of research in nerve and spinal cord regeneration. **Odour Detection Mechanism** **1.** **Olfactory Cilia:** Cilia in the mucus layer are structured to increase odorant detection chances. Each olfactory neuron expresses specific receptor proteins encoded by about 350 genes out of 1,000 available. Each olfactory neuron is sending its hairlike projections into the mucus layer at one pole. At the opponent pole, each of the receptor cells/neurons send their axons to the olfactory nerve where they combined together.  In the genome, there are 1000 genes that encode for olfactory receptor proteins (ORPs), all of which are G-protein coupled receptor. Yet, most of the genes are pseudogenes and do not yield functional information. Probably, the high number of pseudogenes indicates that the sense of smell, once necessary for survival in the early evolution of mankind, has become less important in more recent times. The ORPs differ in the molecular properties of their odorant binding pocket (OBP). It is thought that they are only activated by a family of odorants that fit into this pocket. Odorants can be coded based on which combination of receptors are encoded. **FROM RECEPTOR POTENTIAL TO ACTION POTENTIAL:\ ** 1\. **Olfactory Receptor Neurons (ORNs):** Located in the ciliated layer of the mucosa in the nasal cavity. True neurons, unlike taste receptor cells. *In the ciliated meshwork, there are receptors which are themselves cAMP-dependent receptors that open K+, Na+ and Ca+ channels.* 2\. **Cyclic AMP-Dependent Receptors:** ORNs have receptors that respond to cyclic AMP (cAMP). When an odorant binds to these receptors, cAMP levels increase. 3\. **Ion Channels Activation:** Increased cAMP opens ion channels permeable to sodium (Na+), potassium (K+), and calcium (Ca2+). Ca2+ entry is crucial for the next steps in the signalling process. 4\. **Chloride Channel Activation:** Ca2+ influx activates chloride (Cl-) channels. ORNs actively pump Cl- into the cell, creating a high internal Cl- concentration. 5\. **Chloride Efflux and Depolarization:** The high internal Cl- concentration causes Cl- to move out of the cell when channels open. This outward movement of Cl- depolarizes the cell membrane (makes it more positive inside). 6\. **Action Potential Generation:** Depolarization leads to the generation of a generator potential (graded potential). If this generator potential is strong enough, it triggers action potentials. Action potentials are then propagated down the axon to transmit the signal to the brain. A diagram of a cell Description automatically generated At the level of the receptor surface, the Olfactory Receptor Neuron Membrane change in response to an odorant is due to changes in intracellular chloride concentration. This in turn is due to intracellular calcium concentration. This is somewhat different to other types of sensory receptors where they have a more classical profile of sodium entering the cell **Neural Pathway to the Brain** Each neuron expressing a particular receptor projects to a specific glomerulus within the main olfactory bulb (MOB). The olfactory bulb neurons include mitral cells and tufted cells. This establishes an organisational map at the level of the 2^nd^-order neurons (i.e. red and light green ORNs are scattered randomly throughout the main olfactory epithelium but their axons segregate into different glomeruli). 1\. **From Olfactory Neurons to Bulb:** Olfactory receptor neurons project to the olfactory bulb. Two main cell types in the bulb: mitral and tufted cells. Inputs to the bulb form a spatial map based on odorant types. 2\. **Olfactory Bulb Mapping:** Segregated inputs from the olfactory receptor neurons create a labelled line code (olfactory bulb map). Mitral and tufted cells project to various brain regions, including the amygdala, piriform cortex, and entorhinal cortex. From the diagram below, we can see that aliphatic esters, for example, are projected to the surface of the olfactory bulb in a very systematic way by the olfactory receptor neurons.\ \ **Olfactory Pathway and Memory**  1\. **Direct Projections:** Unlike other sensory systems, olfactory signals bypass the thalamus initially. Direct projections to limbic cortex and primary olfactory cortex. 2\. **Memory Association:** Olfactory signals can strongly trigger long-term memories. Smells can prompt memory recall even in individuals with memory loss. **Disorders of Olfaction** 1\. **Impact on Taste Perception:** Many taste disorders are actually olfactory disorders. Conditions include anosmia (loss of smell) and dysosmia (distorted smell). 2\. **Causes and Effects:** Environmental toxins, diseases, and aging can cause olfactory disorders. These disorders affect quality of life and safety (e.g., detecting fire or fumes). **Odorant Transduction** Odorant molecules bind to receptors on the cilia, activating a G-protein (Golf). This leads to the production of cAMP, opening ion channels and causing depolarization. Chloride efflux further depolarizes the cell, generating action potentials. **Central Pathways** Olfactory receptor neurons project to glomeruli in the olfactory bulb. Mitral and tufted cells in the olfactory bulb send projections to: Piriform cortex (primary olfactory cortex). Amygdala (emotional processing). Entorhinal cortex (memory processing). **Olfactory Coding** Each type of olfactory receptor neuron projects to specific glomeruli, creating a spatial map of odorant types. This map is used to differentiate between thousands of odorants. **Integration and Clinical Relevance** **Integration**: Taste and smell are closely linked, contributing to the perception of flavor. Most disorders of "taste" are actually olfactory (80% approximately). Olfactory input enhances taste perception through retronasal olfaction. **Clinical Conditions**: **Anosmia**: Loss of smell. **Hyposmia**: Reduced ability to smell. **Dysosmia**: Distorted smell perception. **Ageusia**: Loss of taste. **Hypogeusia**: Reduced ability to taste. **Dysgeusia**: Distorted taste perception. These conditions can significantly impact quality of life, highlighting the importance of the chemical senses in everyday functioning and health.