Neuroscience PDF
Document Details
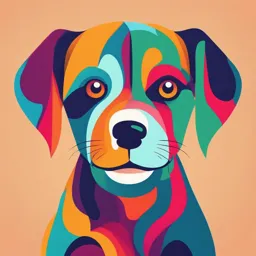
Uploaded by WorldFamousLightYear
Faculty of Medicine
Tags
Summary
This document provides an introduction to neuroscience, focusing on the organization of the nervous system and synaptic transmission. It details the functions, organization, and properties of synapses and their roles in communication within the nervous system.
Full Transcript
# Neuroscience ## Introduction The nervous system is the major controlling, regulatory, and communicating system in the body. It is the center of all mental activity including thought, learning, and memory. Together with the endocrine system, the nervous system is responsible for regulating and m...
# Neuroscience ## Introduction The nervous system is the major controlling, regulatory, and communicating system in the body. It is the center of all mental activity including thought, learning, and memory. Together with the endocrine system, the nervous system is responsible for regulating and maintaining homeostasis. Like other systems in the body, the nervous system is composed of organs, principally the brain, spinal cord, nerves, and ganglia. Together these carry out the complex activities of the nervous system. The nervous system has three broad functions: sensory input, information processing, and motor output. In the peripheral nervous system (PNS), sensory receptors keep us in touch with our environment, both external and internal. This sensory information is then processed by the central nervous system, predominantly by the brain. After information is processed, motor neurons return signals to the muscles and glands of the PNS, which responds with motor output. ## Organization of the nervous system: (Fig 1) The nervous system as a whole is divided into two subdivisions: the central nervous system (CNS) and the peripheral nervous system (PNS). ### 1- Central Nervous System (CNS): Includes the brain and spinal cord. It contains centers for integration of the input signals and for production of output signals. There are three major levels of the CNS: - **Spinal cord level:** for immediate automatic activities such as withdrawal reflexes and evacuation of bladder and rectum. - **Lower Brain Level:** for control of subconscious activities of the body, e.g. cardiovascular and respiratory functions. Also maintenance of posture and equilibrium and emotional reactions. - **Higher Brain or Cortical Level:** for control of motor and sensory functions and other higher functions including: thinking, learning, memory, and speech. ### 2- Peripheral Nervous System (PNS): Is further subdivided into: - **Sensory Division:** (Afferent Division) - Consists of the receptors, axons and cell bodies of sensory neurons. - **Motor Division:** (Efferent Division): is further subdivided into: - **Somatic division:** consists of the axons of motor neurons that innervate skeletal muscles. - **Autonomic division:** consists of the axons of motor neurons that innervate the viscera, smooth muscles, cardiac muscles and glands. ## Synaptic Transmission CNS function depends on synaptic transmission. Synaptic transmission is the foundation of neural communication and information processing in the nervous system. By transmitting impulses from one neuron to another, it allows for the integration and processing of information in the brain. ### What is a synapse? A synapse is a junction between an axon terminal of one neuron (presynaptic neuron) and a second neuron (post synaptic neuron). On average, each neuron has anywhere between a few to hundreds of thousands of synaptic connections. ### Types of synapses: (Fig 2) - **Chemical Synapse:** where the presynaptic neuron releases a chemical substance (called a neurotransmitter) to act on receptors on the membrane of the postsynaptic neuron. Most of synapses in human nervous system are chemical synapses. - **Electric Synapse:** where the neurons are communicated at the cell membrane by low-resistance gap-channel pathway which allows passage of the electric current from one neuron to the other directly. This type is present in few areas in human CNS, e.g. retina, olfactory bulb. ### Function of Synapses: - Synaptic transmission is not simply jumping of an action potential from one neuron to the other. - It is a complex process that permits grading and adjustment of neural activity necessary for integration, interpretation and processing of information at different levels of the CNS by, for example: - Facilitating transmission from one neuron to the next. - Blocking transmission from one neuron to the next. - Amplification of weak impulses. - Distribution of impulses to many directions. - Changing a single impulse to repetitive impulses. - Integration between different types of neurons. - Storage of information, i.e. memory. N.B. It should be noted that synapses are dynamic structures, increasing and decreasing in complexity and number with use and experience. ## Functional Anatomy of a Synapse: (Fig. 3a) ### The Presynaptic Axon Terminal:(or synaptic knob): The synaptic knobs contain important internal structures: - A large number of mitochondria which provides ATP needed for the synthesis and exocytosis of the synaptic transmitters. - A large number of synaptic vesicles which contain the synaptic_transmitters. There are three types of synaptic vesicles: - Small clear vesicles containing the rapidly acting chemical transmitters e.g. acetyl choline, glycine, glutamate and GABA. - Small granular vesicles containing catecholamines. - Large granular vesicles that contain slowly acting neuropeptides. Synaptic vesicles discharge their transmitters at areas of membrane thickening in presynaptic membrane called active zones. ### SNARE proteins: (Fig 3b): - **V- SNARE (Synaptobrevin)** on the synaptic vesicle. - **T- SNARE (Syntaxin)** on the presynaptic membrane. ## Mechanism of Synaptic Transmission: ### 1- Release of chemical transmitter: (Fig 4b) - Arrival of action potential in the presynaptic nerve opens voltage-gated Ca++ channels predominant in this area. - Ca++ enters the knob according to concentration and electric gradients. - Influx of Ca++ triggers binding of the SNARE proteins & fusion of the synaptic vesicles with the presynaptic membrane & exocytosis of their chemical transmitter into the synaptic cleft. ### 2- Binding of chemical Transmitter with its Receptors: Interaction of the chemical transmitter with its specific receptors changes the permeability of the postsynaptic membrane to one or more ions. ### 3- Generation of Postsynaptic Potential: Change in ion fluxes through postsynaptic membrane leads to change in its membrane potential (according to type of NT and/or type of receptor) which may become: - **(less negative)** causing excitatory postsynaptic potential. (EPSP). - **(more negative)** causing inhibitory postsynaptic potential. (IPSP) → EPSP and IPSP are graded potentials whose magnitude and duration depend on the amount of the released neurotransmitter. Therefore, they do not obey All or None rule. ### 4- Removal of Neurotransmitters from the Synaptic Cleft: After combining with the postsynaptic receptor, the chemical transmitter must be removed to terminate its effect, in one of following ways: - Inactivation of the transmitter by specific enzymes within the synaptic cleft, e.g. acetyl choline. - Active reuptake of the transmitter into the axon terminals, e.g. catecholamines, dopamine, serotonin. - Passive diffusion of the transmitter away from the synaptic cleft, e.g. neuropeptides. ## Types of Synaptic Potentials: ### A] Postsynaptic potentials: - **Excitatory postsynaptic potentials [EPSP]:** (Fig 5) - **Definition:** local transient state of partial depolarization of postsynaptic membrane. - **Lonic basis:** - Release of excitatory neurotransmitter and binding to its receptor → opening of ligand gated Na* (or Ca**) channels. - Influx of +ve ions makes the inside of the cell membrane less negative → nearer to the firing level → more excitable. - During this potential, the membrane is said to be facilitated i.e. needs a weaker stimulus to be excited than at rest. - **Amplitude and Duration:** A single EPSP have a mean amplitude of 1-5 mv, and reaches its peak value within 15 msec, then gradually declines due to current leakage. - **EPSP is a local excitatory state.** Up to 40-156/EPSPs have to be summated to reach threshold value needed to elicit an action potential. - **Inhibitory Postsynaptic Potential [IPSP]:** (Fig 6) - **Definition:** local transient state of hyperpolarization of postsynaptic membrane. - **Lonic basis:** - Release of inhibitory neurotransmitter will bind with its receptors → opening of ligand gated K⁺ or Cl channels or closure of Na*(or Ca**) channels. - Influx of -ve ions or efflux of +ve ions makes the inside of the cell membrane more negative → away from the firing level → less excitable. - During this potential, the membrane is said to be inhibited i.e. needs a stronger stimulus to be excited than at rest. ### Types of Summation: (Fig 7) - **Temporal (Time) summation:** Summation of PSPs originating from ONE presynaptic neuron stimulated by rapid repetitive stimuli. - **Spatial (Space) summation:** Summation of PSPs originating from MANY presynaptic neurons stimulated simultaneously. ### 3. Grand Post-Synaptic Potential: A typical neuronal cell body receives thousands of presynaptic inputs carrying sensory information from external and internal environment or from control centers in the brain. At any given time, many presynaptic neurons may be firing at the same time and thus influencing the postsynaptic neuron's level of activity. Grand postsynaptic potential (GPSP) is the sum of all EPSPs and IPSPs occurring at approximately the same time. ## Initiation of Action Potentials at the Axon Hillock: - Axon hillock has lowest threshold in the whole neuron. WHY? - Due to abundant voltage gated Na+ channels. - Any current flowing anywhere on the cell body or dendrites will be summated and spread electrotonically (by current sink) throughout the whole neuron, - Upon reaching the axon hillock, and due to presence of many low threshold voltage-gated Nat channels, it will be depolarized to the firing level thus initiating an action potential. ## Presynaptic Potentials: (Fig 8) The release of neurotransmitter from presynaptic fibers can be facilitated or inhibited by a THIRD NEURON that synapses axo-axonically with the presynaptic terminals of another neuron causing presynaptic potentials. - **Presynaptic Facilitation:** - The axon terminal of a 3rd facilitatory neuron synapses axo-axonically with axon terminal of another sensory neuron. (Fig 9). - The 3rd facilitatory neuron releases "serotonin" → increases CAMP concentration in the presynaptic terminals →phosphorylates K* channels and closes them. = prevent repolrication From occuring. - This delays repolarization and leads to prolongation of the depolarization state of the presynaptic knob, (because outflow of K⁺ is necessary for rapid recovery from the action potential). - The prolonged action potential in turn keeps the voltage gated Ca2+ channels opened for a longer period, allowing tremendous amounts of Ca²+ ions to enter the sensory terminal. - Ca++ influx increases the release of the NT from the presynaptic sensory neuron, which may continue for longer durations (up to 3 weeks). - Example: Sensitization (discussed later) - Significance: Presynaptic facilitation enhances neurotransmitter release from the sensory neuron. - **Presynaptic Inhibition:** - The axon terminal of a 3rd inhibitory neuron synapses axoaxonically with the axon terminal of another excitatory presynaptic neuron. (Fig 9). - The 3rd inhibitory neuron releases an inhibitory chemical transmitter (e.g GABA), which either opens CI channels or K+ channels. - This will hyperpolarize the presynaptic membrane leading to reduced Cat influx to the synaptic knob → reduced release of NT from the synaptic knob and reduced transmission from the presynaptic to the postsynaptic neuron. - Example: pain inhibition (discussed later) - Significance: Presynaptic inhibition permits the selective blockade of a specific synaptic connection, without altering the excitability of the postsynaptic neuron ## Properties of Synaptic Transmission: - **Forward direction:** = uni directional = oneway - Impulses are conducted at the synapse in one way directed from presynaptic to postsynaptic neurons, because the neurotransmitters are formed and released from the presynaptic neuron not the postsynaptic. - **Synaptic delay:** - It is the time taken by an impulse to be conducted through the synapse. It lasts about 0.5 m. sec. - **Synaptic Fatigue:** - Definition: decreased rate of impulses discharged from postsynaptic neuron after repetitive stimulation of presynaptic neuron. It may be due to: - Exhaustion synaptic vesicles in the presynaptic terminals. - Inactivation of postsynaptic receptors. - Significance: The benefit of synaptic fatigue is to stabilize the nervous system, e.g. stopping discharge from overexcited areas in CNS during epileptic seizures. - **Effect of changes in composition of internal environment:** - **pH of the blood:** - **PH↑ Alkalosis:** increases the excitability of neurons and facilitates synaptic transmission due to decrease in ionized Ca**. This may lead to convulsions (e.g. if an epileptic patient hyperventilates, this may precipitate a fit). - **PHTAcidosis:** decreases synaptic transmission due to increase in ionized Ca**. This may lead to coma, e.g. in diabetic Ketoacidosis. Ketone bodies (acid) - **Hypoxia:** - Hypoxia inhibits synaptic transmission. Temporary interruption of cerebral circulation for 3-5 seconds may cause unconsciousness & prolonged ischemia for minutes cause brain damage. - **Hypoglycemia:** - Glucose is the main fuel of the brain, so hypoglycemia inhibits synaptic transmission. - **Hypocalcemia:** - Decreased ionized Catt in ECF facilities synaptic transmission as it increases the excitability of the post synaptic membrane leading to tetanic convulsions. - **Effect of Drugs:** - Most of the drugs, which act on the CNS alter synaptic mechanism, e.g.: - Theophylline, theobromine, and caffeine facilitate synaptic transmission by depolarizing the postsynaptic membrane. ## Synaptic Plasticity Synaptic plasticity means: the ability of neurons to modify the strength of their connections on the basis of past experience. So, the synaptic transmission. can be strengthened or weakened, for short or long duration. These changes occur in parts of the brain concerned with memory and learning particularly the hippocampus. Memory can be classified into short term (lasting seconds to few minutes), intermediate term (lasting minutes, hours, days up to 3 weeks), long term (lasting days, months, years or even lifetime). Short term memories may be transformed to long term in the hippocampus by a process called consolidation, then stored in the neocortex. ### Molecular mechanisms of Synaptic Plasticity #### 1. Short term: Post tetanic potentiation (PTP): - **Definition:** Transient enhancement of synaptic transmission following application of brief tetanizing train of stimuli. - **Lonic basis:** PTP is caused by accumulation (build up) of Ca2+ in the presynaptic neuron after rapid repetitive stimulation → continuous release of neurotransmitter & enhanced EPSPs until the Ca2+ pump is able to remove it. - **Duration:** It may last for few seconds to minutes. - **Example:** it is the basic mechanism of immediate memory for a few facts, words, telephone numbers (7-10 digits). #### 2. Intermediate term: Habituation & Sensitization - **Habituation:** - **Definition:** Gradual decline (or loss) of responsiveness to a harmless (or insignificant) stimulus after repeated exposure to that stimulus. - **Lonic basis:** Habituation is caused by gradual inactivation of Ca2+ channels in the presynaptic terminal →↓ intracellular Ca2+ → ↓ release of neurotransmitter → synaptic transmission becomes gradually weaker - **Duration:** may last up to 3 weeks - **Example:** Neglecting unimportant stimuli e.g. getting used to noises - **Importance:** provides an important mechanism for filtering sensory information, as it allows filtering out irrelevant stimuli and thereby focusing on important stimuli - **Sensitization:** - **Definition:** Augmented responsiveness to stimuli (to which one has become habituated) after being paired with a harmful (noxious) stimulus - **Lonic basis:** (presynaptic facilitation): - A noxious stimulus activates a 3rd facilitator neuron (Which synapse axoaxonically with a sensory neuron) → secreting serotonin CAMP → closure of K⁺ channels → prolonged presynaptic depolarization → prolonged Ca2+ influx → excess neurotransmitter release → enhanced synaptic transmission. - **Duration:** may last up to 3 weeks - **Example:** Walk through the woods. At first you are alert to noises then you habituate. Then a squirrel jumps on you - You immediately become alert and hyper responsive to any voice or movement. - **Importance:** Defensive response; to avoid injury or discomfort. #### 3. Long term: Long term potentiation & depression: Long term memory last from days, weeks, years up to life time. It requires permanent structural changes in the synapse, i.e. protein synthesis and growth of presynaptic and/ or postsynaptic neurons and their connections. - **Long term potentiation (LTP):** - **Definition:** Persistent increase in synaptic strength after application of strong intensity, high frequency stimuli. - **Importance:** it contributes to long term memory storage → learning and long-term memory - **Long term depression (LTD):** - **Definition:** Persistent decrease in synaptic strength after application of weak intensity, low frequency stimuli. - **Importance:** Unlike LTP, it contributes to long term memory (decay). ### lonic basis of LTP and LTD: (Fig 11) - Glutamate released from stimulation of presynaptic neuron binds to AMPA and NMDA receptors in the postsynaptic membrane. Activation of AMPA receptors causes Nat inflow which depolarizes the postsynaptic membrane and thus, relieves the Mg++ block in the NMDA receptor channel, Ca++ enters the neuron. - High frequency stimuli cause a rapid increase in cytoplasmic Ca** which activates a signaling cascade (involving Calmodulin Kinase II) that moves more AMPA receptors into the postsynaptic membrane. The insertion of additional AMPA receptors strengthens the synapse → LTP. - Low frequency stimuli cause a slow increase in cytoplasmic Ca++ which activates a different signaling cascade (involving Calcineurin phosphatase I), which results in the removal of AMPA receptors from the postsynaptic membrane thereby decreasing the synaptic strength → LTD. ## Chemical Transmitters (Neuro-Transmitters) ### What is a chemical transmitter? A substance that mediates chemical signaling between neurons. - Neurotransmitters are endogenous chemical substances released from presynaptic neurons that transmit signals between neurons across a synapse. - A substance can act as a neurotransmitter in one region of the brain while serving as a hormone elsewhere. ### Types of Neurotransmitters: More than 100 different chemical substances function as synaptic transmitters. In general, there are two different groups of synaptic transmitters: - **A. Small molecule, rapidly acting transmitters:** - They are synthesized in the cytosol of the presynaptic knobs, then stored in small vesicles. - The vesicles are continually recycled and reused in the presynaptic terminal. - They are released rapidly in large amounts. - They stimulate ionotropic receptors leading to rapid activation of ion channels. - They cause most of the acute responses of nervous system e.g. transmission of sensory signals to the brain and motor signals back to the muscles. - **B. Large molecules, slowly acting neuropeptides:** - They are synthesized in the soma of the neuron, then transported all the way to the terminal knobs to be stored in large granular vesicless. - After exocytosis, these vesicles are autolyzed and not reused. - They are released at a slower rate and in smaller amounts than small molecule neurotransmitters, however, they are a thousand times more potent. - They often stimulate metabotropic receptors leading to activation of 2nd messenger system. - They often cause more prolonged actions such as, long term opening or closure of ion channels, changes in number of receptors, synapses or genes. ## Organization of Neurons for Processing of Signals in Neuronal Pools The CNS is composed of thousands to millions of neuronal pools. A neuronal pool is a collection of neurons carrying the same function in CNS e.g. cortical neuronal pool, cerebellar neuronal pool and so on. Although each neuronal pool has its own arrangement of neuronal circuits, they all share certain similarities of organization. ### These similar organizations are, for example: - **Divergence:** (Fig 12) - **Definition:** ONE neuron stimulates MANY neurons in a neuronal pool. - **Functions:** - **Divergence in the same direction:** allows Amplification of the signal. Example: one motor neuron in the cortex supplies many muscle fibers. - **Divergence in different directions:** allows Distribution of the signals. Example: a painful stimulus i) stimulates anterior horn cells of muscles on the same and opposite sides, ii) ascends to stimulate cells in the brain stem, and sensory cortex. - **Convergence:** (Fig 12) - **Definition:** MANY neuronal inputs stimulate ONE neuron in a neuronal pool. - **Functions:** Convergence of input signals (excitatory & inhibitory) from different sources: allows summation and integration of information from different sources, and the resulting response is a summated effect of all different types of information. - **Example:** a motor neuron receives different input fibers from a) peripheral sensory fibers, b) propriospinal fibers, c) corticospinal tract from the motor cortex. The signals converge on anterior motor neurons to control muscle function. ### Excitation field: (Fig 13) - **Definition:** It is the number of neurons, with which one afferent neuron synapses. - **The Discharge (excited) zone:** - Neurons that lie in the center of the field receive large numbers of knobs. When the afferent nerve is stimulated, these cells are stimulated enough to reach to the threshold value and discharge nerve impulses. - **The Facilitated zone (subliminal or subthreshold zone).** - Neurons that lie in the periphery of the field receive few numbers of knobs. - The area of discharge zone and subliminal fringe depends on the strength of the stimulus: the stronger the stimulus the wider the discharge zone This arrangement leads to the phenomenon of **Facilitation:** (Fig 14) - **Definition:** If the excitation fields of two input neurons overlap at the peripheral facilitation zones (subliminal fringe), , the outcome of the two pools when stimulated simultaneously is more than the sum of the outcome of both pools, when each neuron is stimulated separately. - **Function:** the two neighboring neurons facilitate each other - **Example:** Referred pain ### Inhibitory Circuits Inhibitory circuits act as a mechanism for stabilizing the nervous system by help preventing excessive spread of signals in widespread areas of the brain. - **Lateral inhibition:** (Fig 15) - **Definition:** Central neuron is stimulated while the neurons at the periphery are inhibited by one excitatory input through inhibitory interneuron. - **Function:** Sharpening of the sensation - **Example:** 1-Skin: (tactile localization), 2-Retina: horizontal cells inhibit peripheral bipolar cells to prevent blurring and sharpen the vision. - **Negative feedback inhibition:** (Fig 16) - **Definition:** When a motor neuron is stimulated it gives off recurrent collateral that synapse with Inhibitory Interneuron (Renshaw cell) which inhibits the motor neuron itself and its surrounding neurons. - **Functions:** Allows focusing & sharpening of the effect. - **Example:** Dampens activity of motor neurons and prevents over excitation - **Reciprocal innervation:** (Fig 17) - **Definition:** It is the stimulation of one muscle and inhibition of its antagonist by excitation of one nerve. This is carried through inhibitory interneurons. - **Function:** Enables contracted muscle to carry its function unopposed ### Activating Circuits: (After Discharge) - **Continued output discharge after stoppage of stimulation of input:** - It may be caused by one of two arrangements. - **Parallel after-discharge circuits:** (Fig 18). - **Definition:** In a parallel circuit, a neuron inputs to several chains of neurons. Each chain is made up of a different number of neurons but their signals converge onto one output neuron. - **Function:** prolongation of signal discharge after the input signal is over. - **Example:** When looking at an image, you will not notice when you blink. - **Reverberatory (Oscillatory = closed circuits):** (Fig 19). - **Definition:** Positive feedback within the neuronal circuit that feeds back to re-excite the input of the same circuit. - **Function:** One of the most important of all circuits in the entire CNS which may continue to discharge for a long period even throughout the whole life. - **Example:** It is the base of wakefulness and sleep and other tonically discharging centers, e.g. respiratory centers. - **Duration of reverberatory circuits is determined by:** - Number of synapses in the circuit. - Fatigue of the synapses. - Facilitatory or inhibitory impulses entering the circuit. ## The "sensory system" is part of nervous system that consist of: 1. Sensory receptors: receive stimuli from the external or internal environment, 2. Sensory afferents: carry information from the receptors to CNS 3. Sensory pathways: conduct information to the brain 4. Sensory cortex: Part of the brain that deal with processing the information. Information processed by the sensory system may or may not lead to conscious awareness of the stimulus. If the information does reach consciousness, it can be called a sensation. A person's understanding of the sensation's meaning is called perception. ### Sensations are classified into: unconsciousness. - Special senses: Vision, hearing, taste and smell. - Somatic sensations: arise from different parts of the body: ## Mechanoreceptive Sensations ### 1.Touch - **Crude Touch** - **Fine Touch:** - Tactile localization - Texture detection - Tactile discrimination - **Pressure** - **Vibration** - **Stereognosis** - **Graphasthesia** ### 2. Position - **Static position sense:** conscious awareness of angle of joints - **Kinetic position sense:** awareness of rate of movement ### Cortical Sensations - **Fine pressure:** Ability to Discriminate between weights - **Stereognosis:** Ability to recognize objects in hand with eyes closed by touch. - **Graphasthesia:** Ability to recognize symbols traced on skin. - **Tactile Localization:** Ability to localize the exact site of tactile stimuli -Ability to detect two touched points applied to the skin as two separate points. - **Two point discrimination:** Measured by threshold distance (shortest at lips & finger tips and largest at back) - **Texture Recognition:** Ability to recognize texture of materials ## Thermoceptive Sensations - **Definition:** conscious perception of different grades of temperature. - **Receptors:** Thermoreceptors are Free nerve endings. - **Afferents:** C fibers (for warm) and As fibers (for cold). - **Pathway:** Lateral Spinothalamic tract ### Types of thermoreceptors: - **Warm receptors:** respond from 30-45°C. - **Cold receptors:** respond from 10 - 40°C. - **Warm pain receptors:** start to be stimulated >45 °C. - **Cold pain receptors:** start to be stimulated <10°C. - At zero C, no receptors discharge and a state of anesthesia occurs. - At area of overlap (between 30°C and 40°C) both cold and warm receptors discharge and are very sensitive to fine gradations of temperature changes (Fig 30) ### Distribution of thermal receptors: - Thermal receptors are located immediately under the skin, thus they respond to the temperature of the subcutaneous tissue surrounding them and not to the environmental temperature. - They are numerous in the lips then finger tips and least in the trunk. - Cold receptors are 4-10 times more numerous than warm receptors. - They are widely separated. So, to differentiate between different degrees of temperature, a wide area of skin has to be exposed to allow spatial summation. ### Adaptation of thermal receptors: - Adaptation occurs between skin temperature 20-40°C, and warm receptors adapt faster than cold receptors. - Both receptors adapt rapidly at first (phasic component) then continue to generate impulses at low frequency (tonic component). ### Mechanism of stimulation: - Thermoreceptors are stimulated chemically by changes in the concentration of metabolites accumulated due to change in metabolic rate (each 100C increase, doubles the metabolite concentration). - When activated they open membrane ion channels known as "Temperature-activated Transient Receptor Potential" (TRP) ion channels. - They respond markedly to changing temperatures rather than steady states of temperature: - Warm receptors increase their firing when temperature is increasing. - Cold receptors increase their firing when temperature is decreasing. - Sensation produced by a transient change in temperature is relative, i.e. depends upon the original skin temperature. Thus, a stimulus of 35°C will feel warm if the skin is at 30°C and will feel cold if the skin is at 40°C. ## Pain Sensation Pain is an unpleasant sensory and emotional experience for body protection. - It occurs whenever there is physical or potential tissue damage. - It causes the person to react to remove the pain stimulus or seek medical advice. ### Pain Receptors - **Types of Pain Receptors: (Nociceptors)** Pain receptors are Free Nerve Endings attached to Ad and C fibers. - **They are classified according to the mode of stimulation into:** - **Mechanical pain receptors:** stimulated by mechanical injurious stimuli, e.g. cuts, bruises. - **Thermal pain receptors:** respond to extremes of temperature and capsaicin (substance present in hot chilli). - **Chemical pain receptors:** stimulated by chemical injurious elements or chemicals produced from tissue damage. - **Polymodal pain receptors:** respond to all types of stimuli. ### Distribution of Pain receptors: - They are most numerous in superficial layers of the skin. - They are also numerous in periosteum, peritoneum, pleura, joints, arterial walls, dura and tentorium of the cranial cavity. - They are less distributed in deep tissues and very few in internal viscera. - They are absent in liver parenchyma, lung alveoli, and brain tissue (pain insensitive structures). ### Adaptation: Slowly or non-adaptive receptors. ### Pain Transduction by Nociceptors: - Noxious stimuli open transduction ion channels in pain receptors which increase membrane permeability to Na+ &/or Ca++ → receptor potential → action potential. - Mechanical stimuli open specific "degenerin" ion channels. - Thermal stimuli open specific "temperature sensing TRP" ion channels. - Chemical stimuli open specific "acid sensing" ion channels. ### Threshold of Pain: - Threshold of pain is the same for all people but reaction to pain differs from one person to another. - Pain starts to be felt when the skin temperature reaches 45°C, this is considered as average threshold of pain. ### Pain Sensitizers (OR Chemical mediators of pain): (Fig 31) - After the nociceptors are stimulated, the damaged tissues and the surrounding blood vessels release a number of pain and inflammation producing chemical substances that are normally inside the cells, into the ECF. - These substances include: histamine, serotonin, K+, ATP, Substance P, bradykinin, and prostaglandins. - These chemicals sensitize the nociceptors, lowering their pain threshold, and producing the primary hyperalgesia that often accompanies pain. N.B: Salicylates and other non-steroidal anti-inflammatory analgesics (NSAIDs) reduce pain by inhibiting prostaglandin synthesis, which points to the importance of these mediators in the promotion of pain, fever and inflammation. ### Hyperalgesia: - **Definition:** Abnormally increased sensitivity to pain leading to exaggerated pain response. - **Importance:** Hypersensitivity following an injury is an important self-preservation mechanism, which allows the injured tissue to heal and to continuously warn/remind the brain to avoid further injury to this area. ### Types: Divided into two subtypes: (Fig 32) - **Primary hyperalgesia:** - Occurs at the site of injury e.g. sunburned skin - Caused by peripheral sensitization of nociceptors by inflammatory mediators. - There is lowered threshold of pain. - **Secondary hyperalgesia:** - Occurs in uninjured tissue surrounding the site of injury e.g. area surrounding sunburned skin - Caused by central sensitization of neighboring pain neurons by the sensitized nociceptos. - Usually accompanied by **Allodynia** (exaggerated pain response to non-painful stimuli), caused by central sensitization of neighboring tactile neurons. ### Types of pain sensation: - **According to site of origin of pain:** (discussed later) - Cutaneous pain. - Deep pain. - Visceral pain. - Neuropathic pain. - **According to quality of pain:** Single painful stimuli evoke 2 successive and qualitatively different sensations (Table 5) - **Fast pain:** also called sharp, immediate or first pain. (Ad fibers) - **Slow pain:** also called dull aching, delayed or second pain. ( C fibers) N.B. The two types of pain can be dissociated from each other by: - **Moderate compression of a nerve trunk will block the Aδ-fibers leaving the C-fibers. Fast pain is lost while Slow pain remains.** - **Low concentration of local anesthetic blocks the C-fibers leaving the Aδ-fibers. The slow pain is lost while the fast type remains.** ## Characters of fast pain and slow pain | Quality | Fast Pain | Slow pain | |---|---|---| | Quality | Sharp, pricking, acute | Dull aching, Burning, throbbing | | Onset | Immediate | Delayed | | Duration | Short duration | Long duration; increase with time | | Localization | Well localized | Poorly localized (diffuse) | | Stimulated Receptor | Mechanical & thermal | All types of pain receptors | | Felt in | Skin and Parietal surfaces | All tissues (Skin, deep tissues, viscera) | | Carried by | Ad fibers | C fibers | | Blocked mainly by | Hypoxia & compression | Local anaesthesia (e.g. cocaine) | | Neurotransmitter | Glutamate | Substance P | | Pathway | Neospinothalamic Tract | Paleo-spinothalamic Tract | | Relay of 2nd order neurons | VBN of thalamus | Reticular Formation → intralaminar thalamic N → whole cortex | | Termination of fibers | sensory cortex | | | Pain perception | In Thalamus and Sensory Cortex | Mainly In Thalamus | | Motor reflexes | Flexor Withdrawal reflex | Guarding: hypertonia of overlying muscles | | Autonomic reactions | Pressor response: (↑BP & HR) | Depressor response: (↓BP & HR) Nausea and vomiting | | Emotional Reactions | Anxiety | Depression | ## Ascending Pain Pathway - **Afferents:** Αδ (carrying fast pain) and C fibers (carrying slow pain) - **First order neurons:** dorsal root ganglia (DRG). - **Second order neurons:** Posterior horn cells (PHCs) on the same side - Type Ad fibers release glutamate and synapse with PHCs in lamina I and V, - Type C fibers release Substance P and synapse with PHCs in lamina II and III (i.e. substantia gelatinosa of Rolando or SGR). - Pain fibers then cross and ascend in the lateral spinothalamic tract in the opposite side of the spinal cord. - **Fast pain fibers ascend as Neospinothalamic tract (Fig 33-A)** - **Slow pain fibers ascend as Paleospinothalamic tract. (Fig 33-B)** ### Third order neurons: - **Fast pain fibers (Neospinothalamic Tract)** end in the ventrobasal nuclei of the thalamus (VBN), then project to end in the somatic sensory cortex. - **Slow pain fibers (Paleospinothalamic Tract)** also known as spino-reticulo-thalamic tract, reach the brain stem, where: - 10% of the fibers join the neospinothalamic tract to synapse in the VBN of the thalamus then end in the somatic sensory cortex. - 90% of the fibers project bilaterally to neurons of the reticular formation, then synapse with the intralaminar (non specific) nuclei of the thalamus (spino-reticulo-thalamic), which project to activate the whole cortex. ### Reaction to Pain: Along their way upwards, the pain fibers give tributaries to: - Anterior horn cells in the spinal cord for accompanying motor reflexes. - Reticular Activating System for cortical activation and arousal reactions. - Hypothalamus for accompanying autonomic reactions. - Limbic system for emotional reactions. ### Arousal reaction to pain signal: - The intralaminar (non-specific) nuclei of the thalamus and reticular formation of the brain stem have a strong arousal effect on nervous activities through the reticular activating system (RAS) of the brain. - This explains why a person with severe pain is strongly aroused. ### Function of cerebral cortex in Perception of pain signals: - Cerebral cortex is not essential for perception (conscious awareness) of pain. - Pain perception can