Fourth Year Nervous System Lecture 1 PDF
Document Details
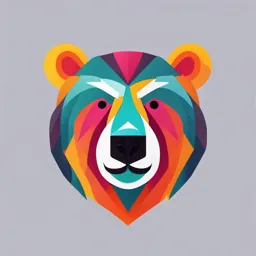
Uploaded by BoundlessSynergy559
Tags
Related
- Neurobiology of Neurons and the Neuroglia PDF
- Neuroscience 1 - Trans 1 - Nerves and Neurons PDF
- Chapter 3 PDF - Cells of the Nervous System
- ASD 3003 Human Physiology L4 Nervous System PDF
- Chapter 2: Structure and Functions of Cells of the Nervous System PDF
- BIO 203 Lecture Notes - Nervous Tissue PDF
Summary
This document provides an overview of neurons and nervous system functions for a fourth-year undergraduate neuroscience course. It explains the neuron structure and types, the different classifications of neurons, as well as synaptic transmission. The document includes diagrams, but is not formatted to be an exam paper (no question sections).
Full Transcript
The NEURON The nervous tissue composed of 2 broad classes of cells: neurons & glial cells Neuron is the functional unit of NS that performs the function of information transfer Glial cells are supportive cell for other functions There are about 1014 neurons in our NS The neurons do not r...
The NEURON The nervous tissue composed of 2 broad classes of cells: neurons & glial cells Neuron is the functional unit of NS that performs the function of information transfer Glial cells are supportive cell for other functions There are about 1014 neurons in our NS The neurons do not reproduce Most neurons contain a cell body and two types of processes— dendrites and axons. With some exceptions the cell bodies and the dendrites of the neurons are in the central nervous system while the axons are in the peripheral nervous system Structure of the Neurons The cell body (soma or perikaryon): Contains the nucleus and ribosomes and thus it controls the neuron metabolic activity and has the genetic information and machinery necessary for protein synthesis. The dendrites : Are a highly branched outgrowths of the cell that receive incoming information from other neurons The axon: Is a long single process that extends from the cell body and carries outgoing signals to its target cells. Axons range in length from a few microns to over a meter. The region of the axon that arises from the cell body is known as the axon hillock. The axon (continue) In most cases the axon hillock is the location where electrical signals (action potentials) are generated and propagated away from the cell body along the axon. The axon may have branches, called collaterals near their ends, both the axon and its collaterals undergo further branching ends in an axon terminal responsible for releasing neurotransmitters from the axon. The axons of many neurons are covered by sheaths of myelin wrapped around the axon by a supporting cells (oligodendrocytes in the central and Schwann cells in the peripheral nervous system The myelin sheet is do not cover the axon completely but in intervals. it paces between adjacent myelin are called the nodes of Ranvier where the axon’s plasma membrane is exposed to extracellular fluid. As we will see, the myelin sheath speeds up conduction of the electrical signals along the axon and conserves energy. Axonal (axoplasmic) transport A great amount of materials must be synthesized in the soma and delivered to the axon to maintain its structure and function. The proteins formed by the soma are engaged into vesicles, some of them is exported to the axon terminals and dendrites (anterograde direction) Some other materials are transported from the axon towards the soma (retrograde direction) The cell’s ability to transport proteins or other components depend on the internal cytoskeleton ( composed of microfilaments, neurofilaments, and microtubules). Axonal transport (continue) Anterograde transport: Is done by transport protein called kinesin. The transport can be slow or fast. The slow is for transporting regenerative materials to repair the axon The fast is for vesicles and organelles transported into axon terminal Retrograde transport: Is done by transport protein called dynein. This is for transport of trophic substances such as nerve growth factors across the synapse from target cells or waste products from axon terminals (vesicles membrane..etc) Functional Classification of Neurons 1) Sensory (afferent) neuron: Carries the information from the target organs back to the CNS 2) Motor (efferent) neuron: Carries the information away from the CNS to the target organs 3) Interneuron: Neurons in the brain and spinal cord that process the information within the central nervous system The Nerves The nerves are the structures that connect the neurons of the central nervous system with the target organs They form the peripheral nervous system Each nerve composed of bundles called fasciculus Each bundle composed of neuronal axons Classification of the nerves The nerves can be classified in different ways: According to the structure: myelinated or non myelinated According to their distribution : - Somatic: to the skeletal muscles - Autonomic to smooth muscles and glands According to their origin: - Cranial nerves from the brain - Spinal nerves from spinal cord According to the transmitters they secretes: - Cholenrgic nerves - Adrenergic nerves Classification of the nerves According to their function: - Sensory nerves - Motor nerves - Mixed sensory and motor According to their diameter and velocity: Note that the thickest the nerve fibers the highest is the velocity Normally all the fibers are myelinated except type c nerve fibers Central nervous system synapse and synaptic transmission Information is transmitted in the central nervous system mainly in the form of nerve action potentials “nerve impulses” through a succession of neurons The impulse pass from one neuron to the other through a functional contact called a “synapse” Each neuron has average of 1,000 to 10,000 synaptic interactions with other cells At the synapse the impulse may be: (1) Block transmission from one neuron to the next (2) May be changed from a single impulse into repetitive impulses (3) May be integrated with impulses from other neurons to cause highly complicated patterns of impulses in successive neurons. Types of Synaptic Transmission There are two types of synapses: - Chemical synapse -most common - Electrical synapse Electrical synapse: Only a few examples of gap junctions have been found in the central nervous system Characterized by direct open fluid channels (gap junctions) that conduct electricity from one cell to the next. These synapses allow the passage of the signals in both directions Gap junctions are more common in the embryonic nervous system Chemical synapse: Almost all the synapses used for signal transmission in the central nervous system of the human being are chemical synapses. The structure of a chemical synapse includes the axon terminal of the presynaptic neuron, a synaptic cleft and a group of receptors in the postsynaptic cell membrane In this type, the first neuron (presynaptic) a neurotransmitter and this transmitter in turn acts on receptor of the next neuron (post synaptic) to excite it, inhibit it, or modify its sensitivity in some other way Chemical synapse always transmit the signals in one direction from pre to post synaptic neurons. It allows signals to be directed toward specific goals. The most known transmitters are: acetylcholine, norepinephrine, epinephrine, histamine, gamma-aminobutyric acid (GABA), glycine, serotonin, and glutamate. The resting membrane potential and action potential Generally speaking, the cells has a difference in ion concentration and electrical discharge across their membrane At rest, the inside of the cell membrane is more negative while the outside is more positive. This difference is called resting membrane potential The resting membrane potential in the nerve average -70mv The resting potential results from the diffusion of ions most important are Na+ and K+ K+ efflux is more than Na+ influx, that’s why more negative charges found inside The action potential is a temporary conversion of the potential inside the membrane into positive. Membrane potential (continue): For the action potential to occur: 1- There must be a stimulus to open Na+ channels 2- Na+ influx must move the membrane towards positive direction till reach the threshold (-55mv), where high number of voltage gated Na+ channels open wide High Na+ influx drive the membrane towards positive value (+35) (depolarization) At this point, the Na+ channels closed and the K+ channels open wide leading to K+ efflux K+ efflux drive the membrane potential again towards the negative value (repolarization) In some conditions the repolarization assume a value more than the resting value, this is called hyperpolarization Generation of nerve action potential The neuro transmitter from presynaptic neuron attached to receptors on post synaptic neuron but the receptors can be excitatory or inhibitory, so the transmitter either excite or inhibit the post synaptic neuron Excitatory Postsynaptic Potential (EPSP): The transmitter acts on the dendrites or the soma excitatory receptor (ligand gated Na+ channels) to increase the permeability to Na+ The rapid influx of positively charged sodium ions to the interior changes the negative resting membrane potential positive This increase in voltage above the normal resting potential is called the excitatory postsynaptic potential (or EPSP) Excitatory Postsynaptic Potential (EPSP) (continue): EPSP is a graded potential not an action potential, means it can be increased by stronger stimuli EPSP causes depolarization locally this depolarization conducted on the soma membrane passively for a limited time and distance. If the graded potential is strong enough to reach the axon hillock it stimulates the opening of Na+ voltage channels which are abundant in the hillock and more Na+ inter the cell and the membrane become more positive If the positivity reach the threshold level (-55) an action potential started in the hillock and propagated along the axon to the terminal The action potential is not graded it follows the rule of all or none Note that action potential do not created on the soma because it has limited number of voltage gated channels on the dendrites and soma membrane Inhibitory Postsynaptic Potential (IPSP): The transmitter acts on the dendrites or the soma inhibitory receptor (ligand gated Cl- channels) to increase the permeability to Cl- The transmitter also may increase the membrane’s permeability to k+ ions leading to efflux of K+ to outside the membrane Both chloride influx and potassium efflux increase the degree of intracellular negativity, which is called hyperpolarization. This inhibits the neuron because the membrane potential is even more negative than the normal so it cancels any excitatory graded potential Impulse Processing: The Neuronal Circuits The central nervous system is composed of thousands to millions of neuronal pools contains mainly interneurons Some of these pools contain few neurons, while others have large numbers Each pool receives input from afferent nerve fiber and processes the information according to the special characteristics of the pool then generates output signals to other neurons The pool of interneurons make synaptic connections with each other Processing of the information includes: - Impulse divergence - Impulse convergence - Impulse Prolongation and Repetitive discharge - Both excitation and inhibition within the circuit Signal Divergence Divergence is when one neuron enter a neuronal pool send signals to excite large numbers of neurons leaving the pool. (one input and multiple output) By this it can amplify the impulse Two major types of divergence occur: 1) Amplifying divergence in the same tract: Is when an input signal spreads to an increasing number of neurons as it passes through successive orders of neurons. 2) Divergence into multiple tracts: In this case, the signal is transmitted into different directions to different areas from the pool. Signal Convergence Convergence means signals from multiple inputs excite a single neuron (one output) Action potentials converging on the neuron from multiple terminals provide enough summation to bring the neuron to the threshold required for discharge Convergence also occurs when multiple terminals from one input neuron converge on one output neuron Convergence cause summation of the signals Prolongation of a Signals The after discharge means that the signals continue to excite the output neuron after stop of the stimulus. By this it prolongs the excitation for example: holding of the hand after a reflex movement (moving the hand away from a source of fire) The signals can be prolonged by two ways: 1) Reverberatory (Oscillatory) Circuit 2) Synaptic After-discharge circuit Reverberatory (Oscillatory) Circuit Such circuits are caused by positive feedback within the neuronal circuit that feeds back to re-excite the out put or even the input of the same circuit. Consequently, once stimulated, the circuit may discharge repetitively for a long time. e.g rhythmic movements like breathing and walking Synaptic after discharge This is seen in some complex functions of the brain The circuits involve divergence followed by convergence The signals from different circuit arrive at the output at different times, so each time one is arrived and excite the output neuron Input output Neuronal Circuit with Both Excitatory and Inhibitory Output Signals Sometimes an input signal to a neuronal pool causes an output excitatory signal going in one direction and at the same time an inhibitory signal going to other output neuron. This involves an inhibitory interneuron Propagation of action potential Once started at any point of the nerve, the action potential spread and propagates along the whole length of the nerve This the all or none principle The velocity of conduction of action potential depends on the diameter of the nerve and whether it is myelinated or not The larger the nerve, the higher the velocity The propagation in the myelinated nerve is much faster called saltatory conduction Saltatory conduction In myelinated nerves, the action potential occurs only at the node of Ranvier So the potential jump from one node to another increasing the velocity and preserving the energy