Respiratory Physiology PDF
Document Details
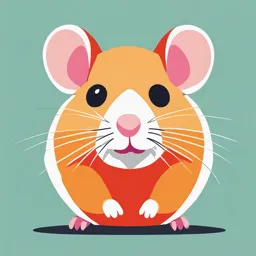
Uploaded by FriendlyMountain
University of Health and Allied Sciences
2024
Isaac Boateng
Tags
Summary
This document is a study guide on respiratory physiology for undergraduate students. It covers different themes like the functional anatomy and physiology of the respiratory system, including the mechanics of breathing and gas exchange. The document also describes the organs of the respiratory system and their roles.
Full Transcript
Respiratory Physiology Isaac Boateng 2024 Learning Objectives FUNCTIONAL ANATOMY: Describe the primary functions of the respiratory system Identify the organs of the respiratory system and describe their functions Relate the function of any portion of the res...
Respiratory Physiology Isaac Boateng 2024 Learning Objectives FUNCTIONAL ANATOMY: Describe the primary functions of the respiratory system Identify the organs of the respiratory system and describe their functions Relate the function of any portion of the respiratory tract to its gross and microscopic anatomy Describe and explain the important structural features of the respiratory membrane PHYSIOLOGY OF RESPIRATION Mechanics of breathing: Define and compare the processes of external and internal respiration Learning Objectives: cont Respiratory volumes and capacities Pulmonary ventilation Exchange of gases: Trace the flow of air from the nose to the pulmonary alveoli Summarize the physical principles governing the movement of air into the lungs and the diffusion of gases into the blood Describe how oxygen and carbon dioxide are picked up, transported and released in the blood Oxygen-Hb dissociation curve Respiration Introduction All body processes require ATP –ATP synthesis requires oxygen(O2) and gives off carbon dioxide(CO2) as byproduct –Drives the need to breathe (take in O2, and eliminate CO2) The respiratory system is a route by which the body is provided with O2 from the atmospheric air and rids it of CO2. The Respiratory system – Consists of a system of tubes that delivers air to the into and out of the lungs – Works with the cardiovascular system (cardiopulmonary system) to deliver O2 to the tissues and remove CO2 – Collaborates with the renal system to regulate the body’s acid–base balance Breathing represents life! – First breath of a newborn baby – Last gasp of a dying person The Respiratory System Nasal Copyright © The McGraw-Hill Companies, Inc. Permission required for reproduction or display. cavity Nostril Epiglottis Pharynx Larynx Esophagus Trachea Left lung Left main bronchus Lobar bronchus Right lung Segmental bronchus Pleural cavity Diaphragm Respiration is the process of exchanging gases between the atmosphere and body cells. Consists of the following events: –Pulmonary ventilation :– moving air into and out of the lungs (breathing) –External respiration :– gas exchange between the lungs and the blood –Transport :– transport of O2 and CO2 between the lungs and tissues –Internal respiration :– gas exchange between systemic blood vessels and tissues Functions: respiratory and Non-respiratory Ventilates the lungs by supplying the body with O2 and removing CO2 from the blood –moving air to and from the lungs (exchange of gases between the atmosphere and the lungs) –Providing an extensive exchange surface area for gas exchange between air (lungs) and circulating blood. Protection of respiratory surfaces from –Dehydration: humidifies and moistens air to become saturated with water vapour – environmental changes through thermoregulation (Heating and cooling of body): air is warmed or cooled to body temperature – Defends against invasion of pathogens and inhaled foreign matter air is 'cleaned' by preventing entry and removal of microorganisms Immune functions ─Airway epithelial cells secrete a variety of molecules that aid in the defense of lungs. Immunoglobulins (IgA), collectins, defensins, peptides, proteases: Act directly as antimicrobials to help keep the airway free of infection. Chemokines and cytokines recruit traditional/ mucosal innate immune cells to the site of infections. ─Mucous membranes contain mucosa- associated lymphoid/lymphatic tissue which produce WBCs-lymphocytes. ─ Immune function within the lung is mediated by alveolar macrophages engulf the particles that reach the alveoli Regulation of blood pH/ acidity Aids in the maintenance of normal acid- base balance as gaseous exchange occurs Altered by changing blood carbon dioxide levels. Metabolic Functions Synthesize and metabolize different compounds eg. surfactant Modify, activate and inactivate substances in the pulmonary circulation vasoactive substances, hormones, neuropeptides, eicosanoids, lipoprotein complexes. Neuro-endocrine cells in lower airways secrete and release chemical mediators: bradykinin, prostaglandins, serotonin, substance P, histamine and heparin (Hemostatic functions). Acts as a Reservoir of Blood The pulmonary vascular bed is a low pressure system, and can occupy a large volume of blood. In the presence of a hypovolemic state, the pulmonary vessels constrict, releasing the blood into the systemic circulation, to increase the effective circulating volume. Filtration of Blood at the Pulmonary Capillaries Venous blood entering the heart, first passes through pulmonary capillaries which are small. Large particles such as emboli, air bubbles, cell debris and fat globules get trapped in the pulmonary vessels, preventing such particles from entering the systemic circulation and obstructing an end-artery supplying a vital organs, such as the brain Production of sound (Phonation) : speech, singing and nonverbal sounds (other vocalizations) Vocal sound is created by the opening and closing of the vocal cords (vibration), caused by force of air flow from the lungs, as air moves past the vocal folds Provides the sense of smell from the olfactory epithelium in the nasal cavity to the CNS. Smell occurs when airborne molecules drawn into nasal cavity Breath-holding helps expel abdominal contents during urination, defecation, and childbirth Organization: upper and lower respiratory tracts Upper respiratory system(in head and neck) – Upper respiratory passages: Nose, nasal cavity, paranasal sinuses, pharynx – Filter and humidify incoming air, protects the more delicate surfaces of the Lower respiratory system – Cool and dehumidify the outgoing air Lower respiratory system(organs of the thorax) – Lower passageways: delicate conduction passages and alveolar exchange surfaces – Larynx, trachea, bronchi, bronchioles, alveoli Anatomy of the Upper Respiratory Tract Nasal septum: Copyright © The McGraw-Hill Companies, Inc. Permission required for reproduction or display. Frontal sinus Perpendicular plate Septal cartilage Nasal Vomer chonchae Pharynx: Nasopharynx Oropharynx Laryngopharynx Anatomy: Lower Respiratory Tract Thyroid Larynx cartilage Cricoid cartilage Trachea Carina Lobar Bronchi (20) Main bronchi Segmental Bronchi (30) Anatomy of the Larynx Epiglottis Epiglottis Epiglottic cartilage Thyroid cartilage Laryngeal Thyroid cartilage prominence Cuneiform cartilage Arytenoid cartilage Corniculate cartilage Vestibular fold Cricoid Vocal cord cartilage Arytenoid cartilage Cricoid cartilage (a) Anterior (b) Posterior (c) Median Mucociliary escalator Mucus traps inhaled particles. The flagella(endothelium) causes a peristaltic wave that traps the foreign matter in the mucus Upward beating cilia drives mucus toward pharynx for swallowing/expectoration Mucus with trapped particles move mechanically upstream by constant beating of the cilia Functions of the Lower Respiratory system Warming, humidifying and filtering of air: Continues as in the nose, although air is normally saturated and at body temperature when it reaches the trachea. Cough reflex: is initiated in response to stretching and irritation to expel foreign particles entering the lower respiratory tract. Nerve endings sensitive to irritation generates nerve impulses, initiates a reflex in which a deep inspiration is followed by a sudden release of air, expelling mucus and foreign material from the mouth. Bronchial Tree Is a branching system of air tubes in each lung – From main bronchus to 65,000 terminal bronchioles – 1⁰ bronchi →2⁰ (lobular) bronchi → tertiary (segmental) bronchi → bronchioles → terminal bronchioles → respiratory bronchioles All bronchi are lined with ciliated pseudostratified columnar epithelium – Lamina propria has abundant mucous glands and lymphocyte nodules, positioned to intercept inhaled pathogens The Bronchial Tree Respiratory tract Consists of the airways that carry air to the lungs –Divided into respiratory and conducting zones Respiratory muscles – diaphragm and other muscles that promote ventilation Conducting zone Conducting zone –Includes all respiratory structures, from the entrance of the nasal cavity down through to the terminal bronchioles –Provides rigid channels for air to reach sites of gas exchange Respiratory Zone Respiratory zone –Site of gas exchange –Consists of bronchioles, alveolar ducts, and alveoli –Begins as terminal bronchioles feeding into respiratory bronchioles –Ends in ducts and sacs –Terminal bronchioles→ Respiratory bronchioles → alveolar ducts → terminal clusters of alveolar sacs → alveoli Lower respiratory tract showing conducting and respiratory zones Alveoli: Approximately 300 million alveoli: –Accounts for most of the lungs’ volume –Provides a better surface area (70 m )for gas exchange 2 Connected to circulatory system via pulmonary circuit (pulmonary artery) Surrounded by fine elastic fibers and contains open pores that: –Connect adjacent alveoli, allowing air pressure throughout the lung to be Blood supply Alveolar walls: –Consists of epithelial cells and macrophages I. Squamous (type I) alveolar cells I. Septal cells (Type II) alveolar cells II. Alveolar Macrophages Copyright © The McGraw-Hill Companies, Inc. Permission required for reproduction or display. Fluid with surfactant Type I alveolar cell Lymphocyte Erythrocyte Septal Alveolar Alveolar Pulmonary cell macrophage capillary I. Alveolar Macrophages (dust cells) Most numerous of all cells in the lung Wander the lumen and connective tissue between alveoli Patrol epithelium and engulf foreign particles Keep alveoli spaces and surfaces both sterile and free from debris by phagocytizing dust particles 100 million dust cells perish each day as they ride up the mucociliary escalator to be swallowed and digested with their load of debris II. Squamous (type I) alveolar cells Thin simple squamous epithelium with broad cells Cover 95% of alveolus surface area Broad cells allow for rapid gas exchange by simple diffusion between alveolus and bloodstream III. Septal cells(Type II) alveolar cells Round to cuboidal cells that cover the remaining 5% of alveolar surface Scattered among the membranes’ squamous cells Repair the alveolar epithelium when the squamous (type I) cells are damaged Secrete SURFACTANT into alveolar surfaces : – An oily secretion containing a mixture of phospholipids and proteins that coats the alveoli – It forms a thin surface/superficial coating over the layer of H2 O (within alveoli), interacting with the H2O molecules(converts air-fluid interface to air-surfactant interface) to reduce surface tension IMPORTANCE: Reduces surface tension and Keeps the alveoli open, helps to avoid alveolar collapse during exhalation Maintains alveolar stability preventing collapse of smaller alveoli Pressure in smaller and larger alveoli become equal preventing smaller radius alveoli emptying into larger radius alveoli Reduction in ultra-filtration Filtration of fluid across the capillary wall into the alveolar interstitium is reduced Inadequate amounts produced o Could be due to injury/genetic abnormalities o will cause alveoli collapse after each inhalation(respiratory distress syndrome) Respiratory Membrane It’s an air-blood barrier between the alveolar air and blood 0.5 to 1 m thick Has a total surface area of 50–70 m2 (40x that of the skin) Composed of Alveolar and capillary walls: –Squamous epithelium(alveolus) –Endothelial cell lining an adjacent blood capillary –and their fused basal membrane Respiratory Membrane Respiratory membrane Squamous alveolar cell Shared basement membrane Capillary endothelial cell Importance of respiratory membrane Important to prevent fluid from accumulating in alveoli –Gases diffuse too slowly through liquid to sufficiently aerate the blood –Alveoli are kept dry by absorption of excess liquid by blood capillaries –Lungs have a more extensive lymphatic drainage than any other organ in the body –Low capillary blood pressure also prevents the rupture of the delicate respiratory membrane Membrane allows the movement of 02 and CO2 by diffusion between the alveolar air spaces and blood –Diffusion is rapid because total distance separating the alveolar air from the blood is small 02 & CO2 are lipid soluble Respiratory membranes(defects): Thicken if lungs become waterlogged and edematous, whereby gas exchange is inadequate and oxygen deprivation results Decrease in surface area with emphysema (progressive respiratory disease), when walls of adjacent alveoli break –Cause: Unknown bronchial spasm, infection, irritation, or a combination can be contributory. – Occurs frequently in heavy cigarette smokers exposed to polluted air. Children with bronchitis or asthma are also susceptible. –characterized by coughing, shortness of breath, and wheezing Extreme difficulty in breathing, Sometimes results in disability and death. The Pleurae Serous membrane which folds back onto itself to form a two layered membrane structure arranged in the form of a closed invaginated sac. Normally adhered to each other Lines each lung and the wall of the chest cavity. –Visceral pleura: inner membrane that covers lungs –Parietal pleura: outer membrane that adheres to mediastinum, inner surface of the rib cage, and superior surface of the diaphragm (chest cavity Pleura: functions Serves in optimal lung function: – Allows for smooth movement of the lungs within the chest cavity. – Allows the volume of the lung to change with the volume of the thoracic cavity –Contributes to the elastic recoil of the lung and lung deflation. Protection –Provides mechanical support to the lung. –Limits lung expansion, protecting the lung –Acts as a cushion for the lungs –Lines the inside of the thoracic cavities. Compartmentalization –Prevents spread of infection from one organ in mediastinum to others Pleural cavity –potential space between pleurae –Normally no room between the membranes, but contains a film/small amount of slippery serous pleural fluid secreted by the pleura –Function: I. aids optimal functioning of the lungs during breathing II. transmits movements of the chest wall to the lungs, the closely apposed chest wall transmits pressures to the visceral pleural surface and hence to the lung. pleural fluid: functions Reduce friction when breathing : – Prevent friction between the lungs and the ribs – Works like a lubricant to aid in the smooth movement : I. of the lungs within the chest/thoracic cavity. II. between individual lobes of the lungs – Establishes adhesion between the layers allowing the pleurae to slide effortlessly against each other during ventilation Provides surface tension –Keeps the lung suitably close to the wall of the thorax, despite the lungs not being directly fixed to it. –Leads to close apposition of the lung surfaces with the chest wall. –Creates pressure gradient, because the closely apposed chest wall transmits pressures to the visceral pleural surface and hence to the lung. Pressure lower than atm pressure during breathing is created; allowing for greater Mechanics of breathing Expected Learning Outcomes – Name the muscles of respiration and describe their roles in breathing. – Describe the brainstem centers that control breathing and the inputs they receive from other levels of the nervous system. – Explain how pressure gradients account for the flow of air into and out of the lungs, and how those gradients are produced. – Explain the significance of anatomical dead space to alveolar ventilation. Pulmonary ventilation (breathing): Is the physical movement of air into and out of the lungs (between the environment and the lungs) From outside the body into the bronchial tree and alveoli. External respiration – Exchange of gases between the body’s interstitial fluid and the external environment (one side of the respiratory membrane) –gas exchange across the respiratory membrane in the lungs air into lungs; gas exchange by diffusion across the membrane b/n alveolar air spaces & capillaries(O load/ CO unload); 2 2 air out; Transport – respiratory gases from and back to the lungs in the blood of the alveolar capillaries are transported to capillary beds and walls of body cells and other tissues Internal respiration –gas exchange across membranes at body capillaries and metabolizing tissues –Absorption of 02 (O2 upload) and the release of CO2 (CO2 offload) between the body’s interstitial fluid and cells Cellular respiration = use of oxygen by cells to produce energy (production of CO2). Respiratory air flow Respiratory airflow is governed by I. principles of flow II. pressure III. resistance (as blood flow) –The flow of a fluid is directly proportional to the pressure difference between two points –The flow of a fluid is inversely proportional to the resistance –Boyle’s law (P=1/V): at a constant temperature, the pressure of a given quantity of gas is inversely proportional to its volume Pulmonary Ventilation Mechanisms Consists of a repetitive (respiratory) cycle of two phases –Inspiration :– air flows into the lungs –Expiration :– gases exit the lungs Movement and flow of air in and out of lung depends on: i. Pressure gradients created between air pressure within lungs and outside body ii. Volume of lungs and thoracic cavity –Breathing muscles change lung volumes in the thoracic cavity and –creates differences in pressure relative to the atmosphere resulting in the flow of gases to equalize pressure V P F (flow of gases) –Movement of air resulting from pressure changes in thoracic volume in turn causes alveolar volume to change. maintain adequate alveolar ventilation (air movement in & out of alveoli) – Boyle’s Law: pressure ↑ses with volume ↓se and vice versa (P=pressure of gas [mm Hg], V=volume [cubic mm]), (Atm pressure = 1 atm or 760mm Hg) Organs involved in ventilation: – Lung – Thoracic cavity – Respiratory muscles The Respiratory Muscles Diaphragm –Prime mover of respiration –Contraction flattens diaphragm, enlarging thoracic cavity and pulling air into lungs –Relaxation allows diaphragm to bulge upward again, compressing the lungs and expelling air –Accounts for two-thirds of airflow Internal and external intercostal muscles –Synergist to diaphragm –Between ribs –Stiffen the thoracic cage during respiration –Prevent it from caving inward when diaphragm descends –Contribute to enlargement and contraction of thoracic cage –Add about one-third of the air that ventilates the lungs 22-70 Scalenes –Synergist to diaphragm –Quiet respiration holds ribs 1 and 2 stationary Some accessory muscles Quiet respiration: while at rest, effortless, and automatic Forced respiration: deep, rapid breathing (eg. during exercise) Inspiration Major events Muscle fibres in the diaphragm contract Dome shaped diaphragm moves downwards thoracic cavity expands External intercostal (inspiratory) muscles contract raising the rib cage, expanding the thoracic cavity further. Lungs are stretched, volume increases Pressure drops below atmospheric pressure (1 mm Hg) The atmospheric pressure is greater on the outside air is forced into the respiratory tract Air fills the lungs, down its pressure gradient, until pressure equalizes Shape of thorax during and at end of inspiration Expiration Major events Normal quiet expiration is achieved by the elasticity of the lungs and thoracic cage The diaphragm and external respiratory muscles relax rib cage descends due to gravity Elastic tissues of the lungs and thoracic cage recoil to original shape and original (smaller) size of thoracic cavity Thoracic cavity & lung volume decreases Lung pressure rises above atmospheric pressure (+1 mm Hg) Air is squeezed out , down the pressure gradient until lung pressure is 0, resulting in airflow out of the lungs Expiration 79 Respiratory muscles and forced respiration Accessory muscles of respiration act mainly in forced respiration Forced inspiration –Erector spinae, sternocleidomastoid, pectoralis major, pectoralis minor, and serratus anterior muscles and scalenes –Greatly increase thoracic volume Forced expiration –Rectus abdominis, internal intercostals, and other lumbar, abdominal, and pelvic muscles –Greatly increased abdominal pressure pushes viscera up against diaphragm increasing thoracic pressure, forcing air out The Respiratory Muscles Inspiration Copyright © The McGraw-Hill Companies, Inc. Permission required for reproduction or display. Sternocleidomastoid (elevates sternum) Scalenes (fix or elevate ribs 1–2) External intercostals Forced expiration (elevate ribs 2–12, widen thoracic cavity) Internal intercostals, interosseous part Pectoralis minor (cut) (depress ribs 1–11, (elevates ribs 3–5) narrow thoracic cavity) Internal intercostals, intercartilaginous part Diaphragm (aid in elevating ribs) (ascends and reduces depth of thoracic cavity) Diaphragm (descends and Rectus abdominis increases depth (depresses lower ribs, of thoracic cavity) pushes diaphragm upward by compressing abdominal organs) External abdominal oblique (same effects as rectus abdominis) Mechanisms of Pulmonary Ventilation Pressures within the respiratory surfaces Intrapulmonary / intra-alveolar/ alveolar pressure: pressure of air measured inside the respiratory tract at the alveoli Intrapleural /pleural pressure: pressure of the fluid in the thin space between parietal (chest wall pleura) and visceral pleura(lung pleura). Transpulmonary pressure: difference between the alveolar pressure(intrapulmonary) and the pleural(intrapleural) pressure :(P – P ) alv ip the Pressure difference between that in the alveoli and that on the outer surfaces of the lungs Pleural Pressure changes During ventilation Inspiration An active phase of respiration as compared to expiration. Expansion of lungs in inspiration is brought about by: I. Intrapleural pressure II. Charles law I. Intrapleural pressure: The cohesive attraction of the two pleural layers to each other, and their connections to the lungs and their lining of the rib cage bring about inspiration – When the ribs swing upward and outward during inspiration, the parietal pleura follows them – The visceral pleura clings to it by the cohesion of water and it follows the parietal pleura – It stretches the alveoli within the lungs – The entire lung expands along the thoracic cage – As it increases in volume, its internal Intrapleural pressure is about −3 to −4 mm Hg before the onset of inspiration Drops to −6 mm Hg during inspiration as parietal pleura pulls away Some of this pressure change transfers to the interior of the lungs causing a change (drop) in the intrapulmonary pressure – Pressure gradient from 760 mm Hg in the atmosphere to 757 mm Hg in alveoli allows air (500ml) to flow into the lungs ii. Charles’s law (law of volumes): –Inhaled air is warmed to 37°C (99°F) by the time it reaches the alveoli –Inhaled volume of 500 mL will expand to 536 mL and this thermal expansion will contribute to the inflation of the lungs –Eg. On a cool day, 16°C (60°F) air will increase its temperature by 21°C (39°F) during inspiration In inspiration, the thoracic cavity expands laterally, vertically, and anteriorly; intrapulmonary pressure drops 3 mm Hg below atmospheric pressure, and air flows into the lungs. Expiration During normal relaxed breathing, expiration is a passive process achieved mainly by the elastic recoil of the thoracic cage –Recoil compresses the lungs –Volume of thoracic cavity decreases –Increases pleural pressure –Raises intrapulmonary pressure –Air flows down the pressure gradient and out of the lungs In expiration, the thoracic cavity contracts in all three directions; intrapulmonary pressure rises 3 mm Hg above atmospheric pressure, and air flows out of the lungs. Alveolar Pressure changes During ventilation Alveolar volume changes cause changes in pressure resulting in ventilation Volume change (collapse and expansion) is caused by – Lung recoil – Pleural pressure 1. Lung recoil (collapse): – Results from: a. elastic recoil by elastic fibres in alveolar wall. b. Surface tension of the film of fluid lining the alveolar 2. Pleural pressure (expansion): – Pressure in the pleural cavity – When lesser (more –ve) than alveolar pressure, lungs expand – The greater the pressure difference, the greater the force of expansion – When sufficiently lower than alveolar pressure, lung recoil is overcome Inspiration: Before the start of breath (end of expiration), pressures inside and out side the thoracic cavity are identical ,no air moves into or out of lungs (fig 1) During inspiration (fig 2): Thoracic cavity enlargens (vol ↑ses), the pleural cavities and lungs expands to fill the additional space created. Expansion lowers alveolar pressure (−3 mm Hg) inside the lungs, air enters Air continues to enter until volume stops increasing and (alveolar) Pinside is the same as (atm)Poutside End of inspiration (fig 3) Thoracic cavity stops expanding, volume ↓ses alveoli also stops expanding, alveolar pressure = atm pressure No air movement, but larger lung volume as compared to end of expiration During expiration (fig 4): Thoracic volume ↓ses, thorax & lungs recoil alveolar volume ↓ses , pressure ↑ses (+3 mm Hg) over atm pressure, air is forced out of the tract PB=Palv Expiration Relaxed breathing –Intrapulmonary pressure increases to about +3 mm Hg –Air flows down the pressure gradient and out of the lungs Forced breathing –Accessory muscles raise intrapulmonary pressure as high as +30 mmHg –Massive amounts of air moves out of the lungs Pressure Relationships Intrapulmonary pressure and intrapleural pressure fluctuate with the phases of breathing Intrapleural pressure is always less than intrapulmonary and atm pressure Lung Collapse: intrapleural = intrapulmonary Intrapulmonary pressure always eventually equalizes itself with atmospheric pressure (determines direction of air flow) Pressure Relationships Respiratory volumes and capacities A spirometer is an apparatus for measuring the volume of air inspired and expired by the lungs (ventilation): Respiratory flow different types use a number of different methods for measurement (pressure transducers, ultrasonic, water gauge). The spirogram will identify two different types of abnormal ventilation patterns: obstructive and restrictive Main piece of equipment used for – basic Pulmonary Function Tests (PFTs), – Lung diseases, – finding the cause for shortness of breath, – assessing the effects of contaminants (eg. Chemicals) on lung functions, – assessing effect of medication, – Measuring progress for disease treatment and – checking lung function before someone has surgery Total volume of the lungs at the end of a maximal inspiration can be categorized into volumes and capacities – Can be represented in graphical readings (spirogram) – These values can be experimentally determined and are useful in diagnosing problems with pulmonary ventilation Relates respiratory performance to volume – Values differ by gender Respiratory volumes Subdivision of the total amount of air that can be contained in the lungs Alveolar volume –Amount of air reaching the alveoli each minute Tidal volume (TV) / (V ) :– T –Amount of air inhaled (moves into ) and (moves out) exhaled with each breath ie a single cycle of inhalation and exhalation Resting tidal volume: TV under resting conditions (approximately 500 ml in both females & males) Respiratory rate(f) : no of breaths taken per minute –Normal rate of a resting adult is 12 -18 breaths/min –For children:18-20 breaths/min Residual volume (RV) –Air remaining in lungs after strenuous or maximum expiration (1200 ml-males, 1100ml- females) Respiratory minute volume (VE) :amount of air moved each minute –Respiratory rate(f) X tidal volume (VT) –Eg. (VE) at rest at 12 breaths / min is 6L / min VE = f x VT =12 x 500ml/ min =6000 ml/ min =6.0L / min Also known as Minute respiratory volume (MRV) Inspiratory reserve volume (IRV) – air that can be inspired forcibly beyond/ in excess of the tidal volume (about 2100–3200 ml):1900ml-females, 3300ml-males approx. Expiratory reserve volume (ERV) – air that can be evacuated from the lungs after a tidal expiration Amount of air that can be voluntarily with maximum effort expelled after a complete normal quiet respiratory cycle (1000–1200 Respiratory Capacities 2/more volumes together Inspiratory capacity (IC) – total amount of air that can be inspired after a tidal expiration/ complete quiet respiratory cycle. (IRV + VT ) Functional residual capacity (FRC) – amount of air remaining in the lungs after a tidal expiration Sum of expiratory reserve volume + residual volume (RV + ERV) Vital capacity (VC) : the total amount of exchangeable air –TV + IRV + ERV –4800-males, 3100-females Total lung capacity (TLC) : maximum amount of air the lungs can contain – sum/ total of all lung volumes TLC = RV + VC (6,000 mL) –(approximately 6000 ml-males, 4200- females) Respiratory Volumes and Capacities Copyright © The McGraw-Hill Companies, Inc. Permission required for reproduction or display. 6,000 Maximum possible inspiration 5,000 Inspiratory Inspiratory reserve volume Vital capacity capacity Lung volume (mL) 4,000 Tidal volume 3,000 Total lung capacity 2,000 Expiratory reserve volume Functional residual 1,000 Maximum voluntary Residual capacity expiration volume 0 Respiratory Volumes and Capacities Respiratory Diseases that can be diagnosed with evaluations of the spirometer Restrictive Disease: –Makes it more difficult to get air in to the lungs. –They “restrict” inspiration. –Decreased VC; Decreased TLC, RV, FRC –Includes: Fibrosis Sarcoidosis Muscular diseases Chest wall deformities Obstructive Disease – Make it more difficult to get air out of the lungs. – Decrease VC; Increased TLC, RV, and FRC – Includes: Emphysema Chronic bronchitis Asthma Alveolar Ventilation The amount of air that reaches the alveoli and is available for gas exchange with the blood per unit time. The volume of air that ventilates all the perfused alveoli The volume of air entering and leaving the alveoli per minute Not all inhaled air gets to the alveoli (dead space), only air that enters the alveoli is available for gas exchange Equal to total ventilation minus dead space ventilation. –(Tidal volume – physiologic dead space) Dead space ventilation Volume of airways and lungs that does NOT participate in gas exchange (Alveoli that are ventilated but not perfused). Anatomical dead space: Conducting division of airway where there is no gas exchange: Volume of conducting airways Is the physical structures that are not involved in the gas exchange process: nose, larynx, pharynx, trachea, bronchi, and bronchioles to the edge of the alveolus. Fixed Alveolar dead space: Alveolus not perfused, so no gas exchange Physiological dead space: the anatomical plus any alveoli that do not take part in gas exchange for whatever reason – Anatomic + alveolar (any pathological alveolar dead space) A person inhales 500 mL of air, and 150 mL stays in anatomical dead space, then 350 mL reaches alveoli Alveolar ventilation rate (AVR) – Air that ventilates alveoli (350 mL) X respiratory rate (12 bpm) = 4,200 mL/min. – Of all the measurements, this one is most directly relevant to the body’s ability to get oxygen to the tissues and dispose of carbon dioxide Gas exchange and transport Expected Learning Outcomes Contrast the composition of inspired and alveolar air. Discuss how partial pressure affects gas transport by the blood. Describe the mechanism of transporting O2 and CO2. Describe the factors that govern gas exchange in the lungs and systemic capillaries. Explain how gas exchange is adjusted to the metabolic needs of different tissues. Discuss the effect of blood gases and pH on Composition of air – 78.6% nitrogen, 20.9% oxygen, 0.04% carbon dioxide, 0% to 4% water vapor, depending on temperature and humidity, and minor gases argon, neon, helium, methane, and ozone – At sea level 1 atm of pressure = 760 mm Hg PN = 78.6% x 760 mm Hg = 597 mm Hg 2 PO = 20.9% x 760 mm Hg = 159 mm 2 Hg PH O = 0.5% x 760 mm Hg = 3.7 mm 2 Hg PCO = 0.04% x 760 mm Hg = 0.3 mm 2 Hg Composition of inspired air and alveolar air is different because of three influences –Air is humidified by contact with mucous membranes in the conducting pathways Alveolar PH2O(water vapour) is more than 10 times higher than inhaled air –Freshly inspired air mixes with residual air left from previous respiratory cycle Oxygen is diluted and it is enriched with CO2 –Gas exchanges in the lungs. Alveolar air exchanges O2 for CO2 from the blood contain more CO2 Gas exchange Involves: – External respiration(Alveolar gas exchange) – Internal Respiration(systemic gas exchange) Systemic gas exchange: – Gas exchange between systemic capillaries and tissue cells – The loading of O and unloading of CO by 2 2 tissues – unloading of O and loading of CO at the 2 2 systemic capillaries Alveolar gas exchange: –The loading of O2 and unloading of CO2 by arterial blood at the lungs –back-and-forth traffic of O2 and CO2 across the respiratory membrane. Alveoli are supplied with O2 that is transported to the blood stream CO2 arriving from the blood. For exchange to occur, gases in the alveoli air or blood stream, either – must dissolve in the film of water covering the alveolar epithelium – Pass through the respiratory membrane separating the air from the bloodstream Exchange is governed by Henry’s law: at the air–water interface, for a given temperature, the amount of gas that dissolves in the water is determined by its solubility in water and its partial pressure in air Gas exchange (diffusion) is determined by: i. Lipid-solubility of gases: –O and CO diffuse readily through the 2 2 surfactant layer, alveolar & endothelial cell membranes –CO is 20x as soluble as O 2 2 Equal amounts of O and CO are 2 2 exchanged across the respiratory membrane because CO is much more 2 soluble and diffuses more rapidly – O is twice as soluble as N 2 2 ii. Small diffusion distance: –Fusion of capillary and alveolar basement membranes reduce distance for gaseous exchange to about 0.5 um iii. Membrane surface area –Due to the large surface area of all alveoli and their capillaries, 100 mL blood spread thinly over 70 m2 iv. Coordination of blood flow and airflow: – Gas exchange requires both good ventilation of alveolus and good perfusion of the capillaries – Good coordination improves both pulmonary ventilation & circulation – Blood flow is greatest around the alveoli with highest PO2values, where O2uptake can start with maximum efficiency – Ventilation–perfusion coupling: the ability to match ventilation and perfusion to each other – Ventilation–perfusion ratio of 0.8 is a flow of 4.2 L of air and 5.5 L of blood per minute at rest Fig. 22.23 Reduced PO2 in Elevated PO2 in Decreased Increased blood vessels blood vessels airflow airflow Response to Result: Response reduced Blood flow to increased ventilation matches airflow ventilation Vasodilation of Vasoconstriction of pulmonary vessels pulmonary vessels Decreased Increased blood flow blood flow (a) Perfusion adjusted to changes in ventilation Reduced PCO2 Decreased Increased Elevated PCO2in in alveoli blood flow blood flow alveoli Response Result: Response to reduced Airflow matches to increased perfusion blood flow perfusion Dilation of Constriction of bronchioles bronchioles Decreased Increased air flow airflow (b) Ventilation adjusted to changes in perfusion v. Differences/gradient in partial pressure of the gases (alveolar and systemic exchange) – The greater the difference in partial pressure, the faster the rate of gas diffusion Partial pressures in the pulmonary circuit: – PO2= 104 mm Hg in alveolar air versus 40 mm Hg in blood – PCO2= 46 mm Hg in blood arriving versus 40 mm Hg in alveolar air Partial Pressures in the systemic circuit: – The partial pressures and diffusion gradients are reversed – PO2in tissue is always lower than in systemic arterial blood supplying them – PO2and PCO2 of venous blood draining tissues are 40 and 45 mm Hg respectively – PO2in the pulmonary veins that enters the systemic circuit are the same as blood reaches the peripheral capillaries – CO2diffuses into blood entering the systemic circuit because peripheral tissues have a higher PCO2(45mm Hg) than blood coming into the systemic circuit (40mm Hg) – Normal interstitial fluid has a PO2of 40mmHg, O2diffuses out of the capillaries into tissues, and CO2diffuses in, until the capillary partial pressures are the same as those in the adjacent tissues Partial Pressure Gradients Changes in Gases Expired air Inspired air PO2 116 mm Hg PO2 159 mm Hg PCO2 32 mm Hg PCO2 0.3 mm Hg Alveolar gas exchange Alveolar air O2 loading PO2 104 mm Hg CO2 unloading CO2 PCO2 40 mm Hg O2 Gas transport O2 carried Pulmonary circuit from alveoli to systemic Deoxygenated Oxygenated blood tissues blood PO2 104-95 mm Hg PO2 40 mm Hg CO2 carried PCO2 40 mm Hg from systemic PCO2 46 mm Hg tissues to alveoli Systemic circuit Systemic gas CO2 O2 exchange O2 unloading CO2 loading Tissue fluid PO2 40 mm Hg PCO2 46 mm Hg Diseases that alter the determinants of gas exchange Certain conditions & fluid build up increases diffusion distance and impairs alveolar gas exchange. When respiratory membrane increases in thickness , gases have to farther to travel between blood and air and cannot equilibrate fast enough to keep up with blood flow Some conditions that thickening membrane: – Inflammation of lung tissue – Pulmonary edema in left ventricular failure that causes edema – Pneumonia Damage to alveolar surfaces reduces available surface area and efficiency of gas transfer – Emphysema – lung cancer, and – tuberculosis decrease surface area for gas exchange Gas Transport It is the process of carrying gases from the alveoli to the systemic tissues and from the systemic tissues back to the alveoli. Involves – Oxygen transport – Carbon dioxide transport O2 transport Molecular oxygen is carried in the blood bound to hemoglobin (Hb) within RBCs and as being dissolved in plasma. – 98.5% bound to hemoglobin, 1.5% dissolved in plasma – Hb is a molecule specialized in oxygen transport with four protein (globin) portions, each with a heme group (non-protein) which binds one O2 to the ferrous ion (Fe2+) – Each Hb molecule binds 4 oxygen in a rapid and reversible process – The hemoglobin-oxygen combination is called oxyhemoglobin (HbO2) – Saturated hemoglobin – when all 4 hemes of the molecule are bound to oxygen 100% saturation Hb with 4 O2 molecules – Partially saturated hemoglobin – when one to three hemes are bound to oxygen 50% saturation Hb with 2 O2 molecules – Hemoglobin that has released oxygen is called reduced hemoglobin (HHb) – Deoxyhemoglobin (HHb)—hemoglobin with no O2 Oxygen Transport Hemoglobin Saturation Curve Oxygen-hemoglobin dissociation curve: presented as a graph that relates the O2 saturation: SO2 (saturation of hemoglobin) to PO2 –the percent O2 Hb saturation plotted against PO2 It is determined by Hb's affinity for O2 : how readily Hb acquires O2 molecules and releases it to its surrounding tissue. –Hb molecule changes shape anytime it binds with 02 affecting its ability to bind to another 02 The Oxygen-Hemoglobin Saturation Curve (Oxyhemoglobin Dissociation Curve) Slope of the curve is gradual until the 1st binds to Hb, then the slope rises rapidly with a plateau near 100% saturation Hemoglobin is almost completely saturated at a PO2 of 70 mm Hg Further increases in PO2 produce only small increases in oxygen binding Oxygen loading and delivery to tissue is adequate when PO2 is below normal levels in the tissues If oxygen levels in tissues drop: –More oxygen dissociates from Hb and is used by cells –Respiratory rate or cardiac output need not increase If oxygen levels increase: –Saturation goes on and Hb stores more oxygen Factors Influencing Hemoglobin Saturation Hemoglobin unloads O2 to match metabolic needs of different states of activity of the tissues Adjustments occur to satisfy the metabolic needs of individual tissues The rate in which hemoglobin binds and releases oxygen is regulated by: –PO2 –blood pH (through the Bohr effect) –temperature –concentration of 2,3-biphosphoglycerate (BPG) – PCO 2 These parameters are all high (except pH) in systemic capillaries where oxygen unloading is the goal. Mentioned parameters affect the up loading and offloading by: –Modifying the structure of hemoglobin and alter its affinity for oxygen –Increased parameters: Decrease hemoglobin’s affinity for oxygen Enhance oxygen unloading from the blood Control decrease increase factors Temperature left shift right shift 2,3-BPG left shift right shift p(CO2) left shift right shift pH (Bohr right shift left shift effect) (acidosis) (alkalosis) Control factors Change Shift of curve ↑ → Temperature ↓ ← ↑ → 2,3-BPG ↓ ← ↑ → pCO2 ↓ ← ↑ → Acidity [H+] ↓ ← Temperature Metabolizing cells(active tissues) produce heat as a byproduct. Active tissues have temp; promotes O2 unloading temp ↑se more O2 release Temp ↓se Hb holds O2 more tightly Effects of Temperature on Oxyhemoglobin Dissociation Copyright © The McGraw-Hill Companies, Inc. Permission required for reproduction or display. 100 10ºC 90 20ºC Percentage saturation of hemoglobin 80 38ºC 43ºC 70 60 50 Normal body temperature 40 30 20 10 0 0 20 40 60 80 10 PO2 (mm Hg) Effect of temperature CO 2 Glucose metabolizing cells release CO into 2 capillary blood, increasing : –PCO 2 –H+ (end prdt of H CO ) in blood 2 3 Changes in the PCO can modify blood pH 2 –The relationship between PCO and blood 2 pH is mediated by Carbonic Anhydrase which converts gaseous CO to carbonic 2 acid that in turn releases a free hydrogen ion, thus reducing the local pH of blood. Effects of CO2 on Oxyhemoglobin Dissociation BPG Is a glycolytic intermediate/ organophosphate produced within the RBC, during glycolysis Is a charged ion that cannot permeate the RBC membrane. Affects the affinity of Hb for oxygen by binding preferentially to deoxyhemoglobin binds to Hb and O2 is unloaded Temperature ↑se and glycolysis(RBC) increase BPG synthesis Irrespective of ↑PO , high conc of BPG 2 causes more O release and less pickup 2 by Hb body temp (fever), thyroxine, growth hormone, testosterone, and epinephrine all raise BPG and cause O unloading 2 pH Declining pH of the Interstitial fluid (acidosis) – (caused by diffusion of H out of RBC) + the shape of hemoglobin changes and weakens the hemoglobin-oxygen bond – Bohr effect: effect of declining pH on the hemoglobin saturation curve Active tissue has CO2, which lowers pH of blood; promoting O2 unloading CO2 is the 1⁰ cpd responsible for the Bohr effect – PCO2 ↑se, plasma/blood pH ↓ses, resulting in a lower affinity of Hb for O2. – PCO2 ↓ses, plasma pH ↑ses, higher affinity of Hb for O2 Net effect of the increase in PCO2 and declining pH(increased H+ conc): saturation Effects of pH on Oxyhemoglobin Dissociation 100 Copyright © The McGraw-Hill Companies, Inc. Permission required for reproduction or display. 90 pH 7.60 Percentage saturation of hemoglobin 80 pH 7.40 70 (normal blood pH 60 pH 7.20 50 40 30 20 10 0 20 40 60 80 0 PO2 (mm Hg) Effect of pH The Bohr Effect refers to the observation that increases the carbon dioxide partial pressure of blood or decreases in Blood pH result in a lower affinity of Hemoglobin for oxygen. This manifests as a right-ward shift in the Oxygen-Hemoglobin Dissociation Curve described in Oxygen Transport and yields enhanced unloading of oxygen by hemoglobin. Regulation of Blood pH The carbonic acid–bicarbonate buffer system resists blood pH changes –Increase in H+ concentrations is removed by combining with HCO3– –With a drop in H+ concentrations , carbonic acid dissociates, releasing H+ Changes in respiratory rate can also: –Alter blood pH –Provide a fast-acting system to adjust pH when it is disturbed by metabolic factors Fetal and Adult Hemoglobin Structure fetal Hb is different from adult Hb causing it to have a higher affinity for O 2 Maternal blood arriving at placenta has a PO b/n 2 35-50mm Hg whiles fetal blood has abt 20mm Hg But at 20mm Hg, due a higher affinity saturation is still abt 58% O enters fetal bloodstream after diffusion b/n 2 fetal and maternal blood until PO is 30mmHg 2 At PO of 30mmHg maternal Hb is 80 % saturated. Steep slope for fetal Hb indicates release of large amounts of O in response to small 2 changes in PO 2 A Functional Comparison of Fetal and Adult Hb CO2 transport CO2 is transported in the blood in 3 forms: –Dissolved in plasma: 7-10% –Chemically bound to hemoglobin: 20 -23% –Transported as bicarbonate (HCO3–) ion in plasma :70% – CO2 + H2O → H2CO3 → HCO3- + H+ Hb binding Bind to the amino groups of plasma proteins and hemoglobin within RBCs to form carbamino compounds CO2 chemically binds to the globular protein portions) within RBC by attaching to exposed amino groups (- NH2) of Hb molecules (carbaminohemoglobin: Hb.CO2) –CO2 + HbNH2↔ HbNHCOOH or Transported as bicarbonate (HCO ) – 3 The bicarbonate ions obtained from the catalytic reaction quickly diffuses from RBCs into the plasma (countertransport mechanism) To counterbalance the outrush of HCO , the 3 – chloride shift occurs The chloride shift is the mass movement of chloride from the plasma into the RBC in exchange for bicarbonate Occurs to maintain electrical balance or neutrality within the RBC – Since the exit of HCO makes the inside 3 – of the RBC positively charged Note: Carbon dioxide does not compete with oxygen They bind to different moieties on the hemoglobin molecule Hemoglobin can transport O and CO 2 2 simultaneously Blood offloads dissolved CO gas and CO 2 2 from the carbamino compounds more easily than CO in bicarbonate 2 CO2 from the tissues CO2 Transport to the lungs Factors that influence binding, transport and offloading of CO 2 Rate of CO2 loading is adjusted to varying needs of various tissues: The amount of CO2 transported is markedly affected by the PO2 – Low levels of oxyhemoglobin enables the blood to transport more CO2 At the lungs, – the lower the PO and Hb saturation with O , 2 2 the more CO can be carried in the blood 2 » achieved by the Haldane effect – binding of O with Hb displaces CO from 2 2 the blood increasing/ promoting CO 2 transport How does the haldane effect achieve increasing CO transport? 2 Haldane effect results from the combination of O with Hb at the lungs causing the Hb ( to 2 be more acidic – Displaces CO2 from the blood and into the alveoli in 2 ways: I. Highly acidic Hb has less tendency to combine with CO2 to form HCO2, displacing much CO2 from the blood II. Increased acidity of Hb causes it to release excess H+ which bind with HCO3– to form H2CO3 that dissociate into H2O and CO2 (released from blood to alveoli to be exhaled) Haldane Effect At the tissues, as more CO2 enters the blood: –More O2 dissociates from Hb (Bohr effect) –More CO2 combines with Hb, and more HCO3– ions are formed This situation is reversed in pulmonary circulation Gas exchange: Systemic and alveolar gas exchange Systemic Gas Exchange CO2 loading –CO2 diffuses into the blood –Carbonic anhydrase in RBC catalyzes CO2 + H2O H2CO3 HCO3− + H+ –Chloride shift Keeps reaction proceeding, exchanges HCO3− for Cl− H+ remain bound to hemoglobin Oxygen unloading –H+ bound to HbO2 reduces its affinity for O2 Tends to make hemoglobin release oxygen HbO2 arrives at systemic capillaries 97% saturated, leaves 75% saturated –Venous reserve: oxygen remaining in the blood after it passes through the capillary beds Systemic Gas Exchange Respiring tissue Capillary blood CO2 7% Dissolved CO2 gas CO2 + plasma Carbamino compounds protein 23% CO2 CO2+Hb HbCO2 Chloride shift Cl– HCO– 70% HCO3 – – Cl– CAH Cl – CO2+H2O H2CO3 + antiport CO2 HCO3– + H 98.5% O2+HHb HbO2+H+ O2 1.5% Dissolved O2 gas O2 Key Hb Hemoglobin HbCO 2 Carbaminohemoglobin HbO 2 Oxyhemoglobin HHb Deoxyhemoglobin CAH Carbonic anhydrase Figure 23.22a Alveolar Gas Exchange Reactions that occur in the lungs are reverse of systemic gas exchange CO2 unloading –As Hb loads O2 its affinity for H+ decreases, H+ dissociates from Hb and binds with HCO3− CO2 + H2O H2CO3 HCO3− + H+ –Reverse chloride shift HCO3− diffuses back into RBC in exchange for Cl−, free CO2 generated diffuses into alveolus to be exhaled Alveolar Gas Exchange Alveolar air Respiratory membrance Capillary blood 7% Dissolved CO2 gas CO2 CO2 + plasma Carbamino compounds protein Chloride shift 23% Cl– CO2 CO2+Hb HbCO2 HCO3 – HCO3– – Cl– Cl– 70% CAH antiport CO2 CO2+H2O H2 CO3 HCO3– + H+ 98.5% O2 O2+HHb HbO2+H+ O2 1.5% Dissolved O2 gas Key Hb Hemoglobin HbCO 2 Carbaminohemoglobin HbO 2 Oxyhemoglobin HHb Deoxyhemoglobin CAH Carbonic anhydrase Control of Respiration Why regulation??????? Rate and depth of breathing are precisely regulated in order to maintain normal levels of partial O and CO 2 2 pressures. Basic elements of the respiratory control system are (1) strategically placed sensors (2) central controller (3) respiratory muscles. Control is by….. I. CNS II. Regulators that modify respiration: Lung sensory nerve signals Chemical control – Peripheral & central chemoreceptor system Voluntary control Interrelation between chemical control and CNS – During respiratory adjustments: Exercise, diving, at high altitudes III. Others: – Irritant receptors: airways – “J receptors”: lungs – Brain edema – anesthesia CNS Breathing (automatic) depends on repetitive stimuli of skeletal muscles, from brain: determined by how frequently the respiratory muscles are stimulated. Basic rhythm of breathing, the rate and depth of respiration is controlled by a groups of nerve cells forming the respiratory center (central controller) located in the brain stem Known as Brainstem Respiratory Centers (RC) – Medullary respiratory center – Pontine respiratory group I. Medullary centers consist of respiratory nuclei called – Dorsal respiratory group (DRG) – Ventral respiratory group (VRG) ii. Pons – Apneustic and pneumotaxic centers Innervations: –Medulla contains inspiratory and expiratory neurons –RC receives controlling signals of neural, chemical and hormonal nature – Motor impulses leaving the RC pass through Fibers of phrenic nerve to supply the diaphragm (DRG) Intercostal nerves to supply intercostal muscles (VRG) RC and innervation Medullary Respiratory center (MRC) It is the pacesetting respiratory center DRG/inspiratory center: –Plays the most fundamental role in control of breathing (respiratory rhythm) –Causes inspiration –Excites the inspiratory muscles and sets eupnea (12-15 breaths/minute) –Becomes dormant during expiration VRG/expiratory center: –Neurons remain almost inactive during normal quiet breathing –Does not participate in rhythmical oscillation control of respiration –Involved in forced inspiration and expiration contributes to extra respiratory drive for increased pulmonary ventilation Stimulation of neurons cause expiration (important in providing power expiratory signals to abdominal muscles during heavy expiration when high levels of pulmonary ventilation are required) Pontine respiratory group (PRG) Considered not essential for the generation of respiratory rhythm Influence and modify activity of MRC Smooth out transitions of inspiration to expiration and vice versa(switching between inspiration and expiration) Adapts and modifies breathing (ventilation) to special circumstances such as during sleep, exercise, vocalization, and emotional responses Apneustic center: –continuously stimulates the medullary inspiration center. –nerve impulses from here provides constant stimulus and influence, preventing inspiratory neurons from being switched off. –without constant influence of this center respiration becomes shallow and irregular. Pneumotaxic center: –continuously inhibits the inspiration center Controls the switch off point of the inspiratory ramp Strong pneumotaxic signal-inspiration is shortened Weak pneumotaxic signal-inspiration is lengthened –Limits inspiration, ↑sing breathing rate Shorter inspiration, less air fills lungs, breathing rate ↑ses, expiration occurs faster and is shortened Longer inspiration, more air, expiration is slower to occur, breathing rate ↓ses Medullary & Pons Respiratory Centers Hering-Breuer Inflation reflex: –inhibitory impulses from receptors are sent to the RC to prevent over inflation – Stretch receptors activates Feedback protective mechanism (response) that switches off “the inspiratory ramp”. – Stops further inspiration and allow expiration – Increases rate of respiration (as signals from pneumotaxic center) 218 Chemical control The respiratory system maintains blood O and CO and blood pH 2 2 concentrations (concs.) within normal values. Chemical control is to maintain proper concs Respiratory activity is highly responsive and controlled by changes of O , CO 2 2 and H concs. in the tissues (blood & + brain). –Any deviation from their normal range has a marked influence on respiratory movements. Chemoreceptors are receptors that respond to changes in the partial pressures of O2, CO2 and H+ concs. in the blood and cerebrospinal fluid. They are located centrally and peripherally. –Central chemoreceptors: located in the chemosensitive area of the medulla oblongata, and connected to the RC. Moniter CO2 and pH (H+ concs) –Peripheral chemoreceptors are found in the carotid and aortic bodies. Moniter O2, CO2 and pH 223 CO is major regulator but the most potent 2 stimulus for breathing is pH, then CO , and 2 least significant is O2 – Blood O levels affect respiration when a 50% 2 or greater decrease from normal levels exists In controlling respiration, – Excess CO and H act directly/indirectly on 2 + RC: increases strength of inspiratory and expiratory motor signals to respiratory muscles – O : Does not have significant direct effect on 2 RC Excess H + Response to Blood pH Excess CO 2 Effect of PCO2 Changes The Chemoreceptor Response to PCO 2 Changes O 2 Stimulates peripheral chemoreceptors which in turn transmits appropriate nervous signals to the RC: indirect effect Chemoreceptors are strongly stimulated when arterial PO falls 2 below normal (50 % decrease produces significant and large stimulatory effect on respiratory movements. Respiratory Centers and Reflex Controls Some respiratory conditions HYPOXIA Hypoxia is decreased supply of oxygen to the tissues. Hypoxemia is defined as a decrease in arterial PO2. Hypoxia is caused by A reduction in partial pressure of oxygen Inadequate oxygen transport The inability of the tissues to use oxygen There are four general types of hypoxia: these are Hypoxic hypoxia Anemic hypoxia Stagnant/ischemic/circulatory hypoxia Histotoxic hypoxia Hypoxic hypoxia It is reduction of O2 entering the Blood. It is the most common type of hypoxia and it is characterized by low arterial PO2 and accompanied by inadequate Hb saturation Causes include: reduction of PAO2 and/or CaO2 reduced gas exchange area lung diseases Anemic hypoxia It is the reduction in the O-carrying capacity 2 of the blood. It is caused by a decrease in circulating rbcs inadequate amounts of Hb in rbcs CO poisoning Stagnant hypoxia is a to deficiency in O due 2 to poor circulation Histotoxic hypoxia the inability of tissue to utilize O. Causes include cyanide poisoning 2 CYANOSIS Cyanosis is a bluish or purplish tinge to the skin and mucous membranes caused by accumulation of more than 5 g/dL of reduced /unoxygenated hemoglobin in the capillaries. Cyanosis may be central or peripheral Central cyanosis There is decreased arterial O2 saturation or an abnormal Hb derivative. Both skin and mucous membranes are affected. Decreased arterial O2 saturation may arise as a result of Decreased atmospheric pressure eg high altitude Peripheral cyanosis It is due to the slowing of blood flow to an area and abnormally great extraction of normally saturated arterial blood. Occurs at fingers and toes. Causes include a. Reduced cardiac output b. Cold exposure c. Redistribution of blood flow from extremities d. Arterial obstruction e. Venous obstruction Chronic Obstructive Pulmonary Disease (COPD) Exemplified by chronic bronchitis and obstructive emphysema Patients have a history of: – Smoking – Dyspnea, where labored breathing occurs and gets progressively worse – Coughing and frequent pulmonary infections COPD victims develop respiratory failure accompanied by hypoxemia, carbon dioxide retention, and respiratory acidosis Asthma Characterized by dyspnea, wheezing, and chest tightness Active inflammation of the airways precedes bronchospasms Airway inflammation is an immune response caused by release of IL-4 and IL -5, which stimulate IgE and recruit inflammatory cells Airways thickened with inflammatory exudates magnify the effect of bronchospasms Tuberculosis Infectious disease caused by the bacterium Mycobacterium tuberculosis Symptoms include fever, night sweats, weight loss, a racking cough, and splitting headache Treatment entails a 12-month course of antibiotics