Respiratory Physiology PDF
Document Details
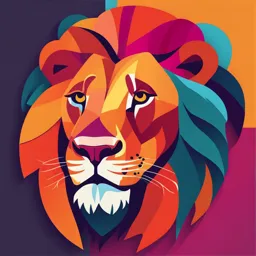
Uploaded by HappyCobalt
Stellenbosch University
Tags
Summary
This document provides an introduction to respiratory physiology, including gas laws, Boyle's Law, Charles' Law, and respiratory mechanics. It covers topics like pressure and volume, and factors affecting gas exchange. It's designed to be a concise overview.
Full Transcript
Pressure & Volume GAS LAWS The atmosphere is a mixture of gases: Nitrogen Oxygen Water vapour Gases move down a pressure gradient - from high to low pressure. 1. Dalton’s Law - Total pressure = sum of partial pressures of individual gases....
Pressure & Volume GAS LAWS The atmosphere is a mixture of gases: Nitrogen Oxygen Water vapour Gases move down a pressure gradient - from high to low pressure. 1. Dalton’s Law - Total pressure = sum of partial pressures of individual gases. - In dry air, water vapour is not present ∴ does not dilute the total Partial pressure: individual gas pressure. pressure in a mixture represented - In humid air, water vapour dilutes the contribution of other gases to as Pgas the total pressure. Dry air Humid air Pgas = Patm x percentage gas in atmosphere Pgas = Patm - PH2O x % gas in atmosphere 2. Boyle’s Law ➔ At any constant temperature the pressure exerted by a gas varies inversely with the volume of the gas. i.e. Inverse relationship between pressure and volume ∴ pressure increases as volume decreases & vice versa. P1V1 = P2V2 3. Charles’ Law ➔ Gas volume is directly proportional to temperature when pressure is constant. ∴ When gas temperature increases, the volume of gas also increases. When gas temperature decreases, the volume of gas also decreases. ∴ Air warmed in conducting part of respiratory system will increase in volume. RESPIRATORY MECHANICS Air moves in & out of lungs from an area of high to low pressure. Pulmonary ventilation: breathing (inspiration & expiration) Alveolar ventilation: gaseous exchange between alveoli and external environment. Respiratory cycle: one inspiration and one expiration. PRESSURE Atmospheric pressure: Pressure exerted by weight of air on earth’s surface = 760mmHg at sea level. Intra-alveolar pressure: Pressure within alveoli. - For inspiration to occur, atmospheric pressure and intra-alveolar pressure need to differ. - At completion of inspiration & expiration Patm = PIAP Intrapleural pressure: Pressure within the pleural sac. - Usually lower than atmospheric pressure. - Gravity & posture affect intrapleural pressure. - Standing upright, gravity pulls the lungs downward away from the apex. - This leads to low intrapleural pressure in apex & high intrapleural pressure in base. ↪ Subatmospheric Intrapleural pressure Elasticity of lungs & alveoli surface tension pull lungs inward towards a collapse. However, the elasticity of the chest wall pulls the thoracic cage outwards. This leads to the expansion of the pleural cavity and subsequent increase in volume. According to Boyle’s Law, as the volume increases the pressure decreases. Transmural pressure gradient: Difference in intra-alveolar and intrapleural pressure. Enables lung stretch. Inspiration ↪ Airflow into the lungs occur when pressure inside the lungs is lower than atmospheric pressure. How does the body increase thoracic volume so that intra-alveolar pressure decreases (Boyle’s Law)? Thoracic volume increases by contraction of skeletal muscles & diaphragm. 60-75% of volume change is due to the diaphragm contracting & flattening. Inspiratory muscles: - Phrenic nerve stimulates the diaphragm to contract causing External intercostals abdominal muscles to bulge outward. Sternocleidomastoids 25-40% of volume change is due to rib cage movement. Anterior serrati - Intercostal nerves stimulate external intercostal muscles to Scalenes contract, moving the ribs & sternum up and outward. 1. Intra-alveolar pressure & intrapleural pressure decreases. - Transmural pressure gradient ensures that lungs expand to maximum capacity. 2. Air flows into lungs. Air flow stops when PIAP = Patm Expiration ↪ A passive process during quiet breathing. 1. Decrease in thoracic volume by the relaxation of inspiratory muscles at the end of inspiration. - Diaphragm returns to a dome-shaped position. - Rib cage falls due to gravity. - Chest wall & lungs recoil due to elastin fibres. 2. Intra-alveolar pressure increases. Air flows out of lungs from high intra-alveolar pressure to lower atmospheric pressure. 3. Air flow stops when PIAP = Patm ACTIVE RESPIRATION/FORCED EXPIRATION 1. Accessory expiratory muscles contract to increase intra-alveolar pressure. - Abdominal muscles exert upward force on the diaphragm into the thoracic cavity. - Ribs are pulled downward & flattens chest wall. 2. Air pushed out of lungs. - Intra-alveolar pressure increases but does not collapse due to transmural pressure gradients. PNEUMOTHORAX Caused by a puncture in the pleural membrane. Air flows down the pressure gradient into the cavity. PIAP & PIPP = Patm Transmural pressure gradient does not exist. No force to stretch the lung ∴ lung collapses & thoracic wall extends outwards. Single inspiration Single expiration 1. Momentary pause between breaths 1. Maximum lung volume - PIAP = Patm PIAP = Patm 2. Start of inspiration 2. Start of expiration - Thoracic volume increases. Thoracic volume decreases - PIAP lowers by 1mmHg. PIAP & PIPP increases - PIPP lowers as the thoracic cage 3. Air flows out of lung expands. PIAP increases above Patm 3. Air flows into lung 4. Pressures equalise - PIAP reaches the lowest value. PIAP = Patm 4. Pressures equalise End of respiratory cycle PIAP = Patm Lung volume at maximum. Pulmonary elasticity Compliance Elastic recoil Effort used to stretch lungs. 1. Elastic connective tissue Stimulated by transmural pressure gradient. Loss of elastic tissue results in a high Low compliance requires large transmural compliance lung. Tissue does not return its gradients upon inspiration to stretch. This resting volume once force is released. happens by vigorous contraction of inspiratory Caused by: muscles. Inflammatory response by macrophages High compliance means the lung stretches too secrete elastase enzyme which breaks down easily. elastin fibres. Decreased compliance is indicative of 2. Alveolar surface tension restrictive pulmonary disease. Caused by: Water found on the surface of the alveoli - Inflammatory response by exchange surface exerts inward forces macrophages secrete factors that (towards collapse) aiding in elastic recoil. stimulate fibroblasts to make inelastic H2O mols are more attracted to other H2O collagen fibres. mols than to air. The cohesiveness of hydrogen binding makes it difficult to stretch. This resists any force that increases surface area and opposes alveolus expansion. Surface tension reduces lung compliance and promotes lung collapse. Breakdown of alveolar walls leads to decreased surface tension. Law of LaPlace ↪ Pressure created towards the centre of the bubble. ↪ If two bubbles have the same surface tension, the smaller one will have a higher pressure P = 2T/r ∴ More work needed to expand smaller alveoli. Alveolar stability ➔ Surfactant Lowers surface tension by disrupting cohesive H2O mol forces. Decreases the resistance of the lungs to stretch. Decreases surface tension to a greater degree in smaller alveoli. Made up of phospholipids (DPCC) & proteins (ABCD) Possess amphiphilic properties. ➔ Alveolar interdependence Alveoli are interconnected which contributes to elastic recoil & prevention of alveolar collapse. VENTILATION-PERFUSION RATIO Ventilation (V) = tidal volume x respiratory rate Perfusion (Q) = total volume of blood reaching pulmonary capillaries per unit time V/Qapex is higher than V/Qbase Ventilation exceeds perfusion in apex. Perfusion exceeds ventilation in the base. However, ventilation per unit lung volume is greater at the base of the lung than at apex. Intrapleural pressure is less negative at base. Transmural gradient is less at base. At rest, base is less expanded ∴ more compliant. This is due to gravity. Gas Exchange FACTORS AFFECTING GAS EXCHANGE: 1. Airway resistance - Determined by airway diameter: Wider airways have less resistance. - Follow Poiseuille’s Law: 𝑛𝐿 𝑅 = 4 where: 𝑟 R= resistance n = viscosity of substance (constant) L = length of system (constant) r = tube radius (determinant) - Increased airway resistance requires more work to be done. It is an indication of pathology e.g. COPD: chronic bronchitis, emphysema, asthma. 1. Airflow & resistance - AIrflow is affected by airway resistance therefore there is a flow-resistance relationship. 4 𝑟 Δ𝑃 𝐹 = 𝑛𝐿 - NUmber of airways affects airway resistance. - More airways increase paths for air to flow and decrease resistance. - Terminal bronchioles are small but have great numbers. Therefore, they have less total resistance than bronchi. 2. Collapsibility of bronchioles - Bronchoconstriction: Increased resistance to airflow. Stimulated by parasympathetic system & histamine release. - Bronchodilation: Decreased resistance to airflow. Stimulated by adrenaline (B2-receptors) or high CO2. CONTROL OVER AIRWAY RESISTANCE & PERFUSION IN MAINTAINING ADEQUATE GAS EXCHANGE. When the rate at which the heart supplies deoxygenated blood to the lungs is greater than the rate of gaseous exchange, the following occurs concurrently: Airflow increase: Decreased perfusion: - Smooth muscle relaxation. - Vasoconstriction of pulmonary arterioles. - Decreased airway resistance. - Stimulated by local decrease in alveoli O2. - Stimulated by local CO2 increase in alveoli. This occurs due to gravitational differences in ventilation & perfusion of apex & base of lung. GAS EXCHANGE & TRANSPORT 1. Gas enters the bloodstream at the alveolar-capillary interface. 2. O2 transported via blood (diffused in plasma or bound to Hb in RBCs) 3. O2 diffuses into cells. 4. CO2 diffuses out of cells. 5. CO2 transported via blood (dissolved, bound to Hb or as HCO3) 6. CO2 enters alveoli at the alveolar-capillary interface. NORMAL BLOOD VALUES: PO2 95mmHg arterial 40mmHg venous PCO2 40mmHg arterial 46mmHg venous pH 7.4 arterial 7.37 venous DEFINITIONS: Hypoxia: too little oxygen Hypercapnia: high CO2 concentration ➔ Regulation of O2, CO2 & pH is essential to avoid hypoxia & hypercapnia. Hypoxic hypoxia - Low arterial PO2. Anaemic hypoxia - Decreased total amount of O2 bound to haemoglobin. Ischemic hypoxia - Reduced blood flow. Histotoxic hypoxia - Failure of cells to use O2 because cells have been poisoned. GAS EXCHANGE BETWEEN ALVEOLI & BLOOD PO2 alveolar > PO2 blood PCO2 blood > PCO2 alveoli GAS EXCHANGE BETWEEN BLOOD & TISSUES PO2 blood > PO2 tissue PCO2 tissue > PCO2 blood FACTORS AFFECTING EFFICIENCY OF ALVEOLAR GAS EXCHANGE 1. Adequate oxygen must reach alveoli Inadequacy could be due to - Change in atmospheric composition due to altitude or humidity. - Low alveolar ventilation by decreased lung compliance, increased airway resistance or central nervous system depression. 2. Transfer of gases between alveoli & pulmonary capillaries i.e. effective diffusion. Affected by: - Surface area - Diffusion distance - Barrier permeability DIFFUSION Diffusion rate ∝surface area x concentration gradient x barrier permeability. Diffusion rate ∝1/distance - Diffusion is most rapid over short distances. - Diffusion distance, surface area & barrier permeability are mostly constant. Thus, concentration gradient is the primary factor affecting gas exchange in healthy people. TRANSPORT OF GAS IN BLOOD Gases dissolve in plasma, are carried by Hb or converted to other molecules. OXYGEN TRANSPORT - 2% Oxygen dissolved in plasma - 98% Oxygen carried by Hb - Total O2 = dissolved O2 + O2 bound to Hb ★ Fick equation - estimated oxygen consumption per O2 blood flow. Q(O2) = Cardiac output x (Arterial O2 - Venous O2) Combination of mass flow equation (amount of oxygen taken up by cells) & mass balance equation (total flow of oxygen) ★ Haemoglobin Hb + O2 → HbO2 Made up of 4 globular protein chains Centred around a heme group with an iron atom. One iron atom binds with one O2 molecule. 1 heme molecule can bind 4 O2 molecules. Hb binding increases oxygen transport → it can carry 197 ml O2/L blood Oxygen binding follows the equation. As the concentration of O2 rises, O2 binds to Hb shifting the equation to the right. If O2 concentration decreases, O2 will unbind from HbO2 shifting the equation to the left. This occurs until the reaction equilibrates. Factors affecting O2-Hb binding 1. PO2 in plasma determines % saturation of Hb. ➔ Composition of inspired air. ➔ Alveolar ventilation rate. ➔ Efficacy of gas exchange. 2. Number of Hb binding sites. ➔ Hb molecules count in RBCs. ➔ RBC count. Oxyhaemoglobin saturation or dissociation curves: Normal arterial PO2 = 98% Hb bound to O2. Person at rest PO2 blood = 40mmHg. Only ¼ O2 released at rest. 75% Hb saturation = reserve capacity of O2. Foetal Hb = enhanced binding of O2 in a low O2 environment. Factors stimulating the release of O2 from haemoglobin (Bohr effect) 1. High acidity (low pH) - Anaerobic metabolism releases H+ into cytoplasm. - Increased CO2 & lactic acid. 2. Temperature increase 3. Increased 2.3 - BPG levels. - Chronic hypoxia - High altitude - Anaemia CARBON DIOXIDE TRANSPORT → removal of CO2 is essential to prevent acidosis, pH changes and depression of the central nervous system. 1. Dissolved in plasma (7%) 2. Bound to Hb (23%) CO2 + Hb → HbCO2 (carbamino haemoglobin) 3. Converted to bicarbonate (70%) CO2 + H2O ↔ H2CO3 ↔ H+ + HCO3 - CO2 is first catalysed by carbonic anhydrase to form H2CO3. - H+ binds to Hb in RBCs. - HCO3 moves into plasma. As dissolved CO2 moves into the alveoli, it decreases local plasma O2. Decreased CO2 levels stimulate the production of CO2, thus H+ unbinds from Hb + HCO3 moves into RBC for CO2 production. (Haldane effect - stimulation of the release of CO2 from Hb) REFLEX CONTROL OF VENTILATION - Medulla controls inspiratory & expiratory muscles. DRG via phrenic & intercostal nerves → Inspiratory muscles - Pons integrates sensory information & influences ventilation. Chemo- & mechanoreceptors via vagus & glossopharyngeal → PRG - VRG controls the larynx, pharynx & tongue. Houses Pre-Botzinger complex which controls the rhythm of breathing. - Ventilation is modulated by receptor linked eflexes & higher brain centres. FACTORS STIMULATING CHEMORECEPTORS Peripheral Central Low PO2 in arterial blood Stimulates when PO2 < 60mmHg Depresses CNS when PO2 < 60mmHg High PCO2 in arterial blood Weakly stimulates Dominant stimulant. Depresses chemoreceptors when PCO2 > 70 - 80mmHg High H+ in arterial blood Stimulates No effect OTHER FACTORS AFFECTING VENTILATION CONTROL Bronchoconstriction Stimulated by inhaled irritants. Hering-Breur reflex High tidal volume stimulating termination of inspiration & start of expiration. Emotional state Stimulated/mediated by the limbic system. Lung Volumes & Capacities Determined by pulmonary elasticity (compliance & elastic recoil) With less compliant lungs, there is greater elastic recoil. Lung volume intake is low but transpulmonary pressure (relative pressure between alveoli & intrapleural space) is high. With more compliant lungs, there is less elastic recoil. Lung volume is high but transpulmonary pressure is low. ASSESSING PULMONARY FUNCTION Spirometer measures first how much air moves during quiet breathing & then with maximum effort. Lung volumes Tidal volume (Vt): 500ml Volume of air that moves during single inspiration or expiration. Inspiratory reserve volume (IRV): 300ml Additional intake of air at the end of quiet inspiration. Expiratory reserve volume (ERV): 1100ml Additional air expelled after normal expiration Residual volume (RV): 1200ml Volume of air in the system after maximum exhalation. Lung capacities Lung capacities formulae Vital capacity (VC); 4600ml VC = Vt + IRV + ERV Maximum air voluntarily moved into and out of lungs TLC = VC + RV with a single breath following maximal inspiration. IC = Vt + IRV Total lung capacity (TLC): 5800ml FRC = ERV + RV Maximum volume of air that lungs can hold. Inspiratory capacity (IC): 3500ml Maximum volume of air that can be inspired. Functional residual capacity (FRC): 5800ml Volume of air in the lungs at the end of normal inspiration. EFFICACY OF VENTILATION Total pulmonary ventilation = volume of air in & out of lungs per minute = ventilation rate x tidal volume - Not all inspired air reaches exchange surface: 1. Anatomic dead space: air remaining in conducting airways roughly 150ml 2. Alveolar dead space: low perfused alveoli (high ventilation-perfusion ratio) Dead space ventilation = ventilation rate x dead space volume Alveolar ventilation = ventilation rate x (tidal volume - dead space volume) FORCED VITAL CAPACITY TEST ➔ Assesses respiratory function & static lung volumes. Forced expiratory volume in 1 second (FEV1) = volume of air leaving airway in the first second. - For restrictive lung diseases total lung capacity, inspiratory capacity & vital capacity are reduced. - However, FEV1 remains normal. FEV1/FVC ratio provides an indication of pulmonary diseases. Normal ratio values are 80% or more, whereas for obstructive diseases it is lower than 80%. Restrictive diseases will present a normal ratio.