REPP Module 3 Wind Energy and Biomass PDF
Document Details
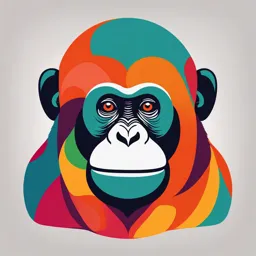
Uploaded by JoyfulMermaid7354
RNS Institute of Technology
Tags
Summary
This document is a module on renewable energy, focusing on wind energy and biomass energy. It covers topics such as wind turbine types, wind power, energy plantation, biogas production, and photosynthesis. It's intended for an undergraduate engineering course.
Full Transcript
Renewable Energy Power Plants (21ME652) Module 3 Module 3 Wind Energy: Properties of wind, availability of wind energy in India, wind velocity and...
Renewable Energy Power Plants (21ME652) Module 3 Module 3 Wind Energy: Properties of wind, availability of wind energy in India, wind velocity and power from wind; major problems associated with wind power, wind machines; Types of wind machines and their characteristics, horizontal and vertical axis windmills, elementary design principles; coefficient of performance of a windmill rotor, design aspects, numerical examples. Energy from Biomass: Energy plantation, biogas production from organic wastes by anaerobic fermentation, description of bio-gas plants, transportation of biogas, problems associated with bio-gas production, application of biogas, application of biogas in engines, cogeneration plant, advantages & disadvantages. Contents 1 Wind Energy.............................................................................................................................. 3 1.1 Introduction, Properties of wind...................................................................................... 3 1.2 Availability of wind energy in India................................................................................ 4 1.3 Basic Principles of Wind Energy Conversion................................................................. 6 1.3.1 The Nature of the Wind............................................................................................ 6 1.3.2 The Power in the Wind............................................................................................. 6 1.4 Major problems associated with wind power.................................................................. 8 1.5 Aerodynamic consideration in wind turbine................................................................... 9 1.5.1 Terms Associated with Aerofoil.............................................................................. 9 1.5.2 Aerodynamic forces on aerofoil blades................................................................ 10 1.6 Types of wind machines and their characteristics........................................................ 11 1.6.1 Horizontal Axis Turbines....................................................................................... 11 1.6.2 Vertical Axis Wind-Mill........................................................................................ 13 1.7 Advantages and disadvantages of wind energy............................................................ 14 1.7.1 Advantages.............................................................................................................. 14 1.7.2 Disadvantages......................................................................................................... 15 1.8 Problems in operating large wind power generators.................................................... 15 2 Energy from Biomass.............................................................................................................. 17 Department of Mechanical Engineering, RNS Institute of Technology. Page 1 of 34 Renewable Energy Power Plants (21ME652) Module 3 2.1 Photosynthesis................................................................................................................. 19 2.1.1 Photosynthetic oxygen production........................................................................ 19 2.2 Energy Plantation............................................................................................................ 20 2.3 Biogas generation............................................................................................................ 21 2.3.1 Anaerobic digestion................................................................................................ 21 2.4 Classification of biogas plants........................................................................................ 22 2.4.1 Continuous and batch type..................................................................................... 23 2.4.2 The dome and the drum types................................................................................ 23 2.4.3 Different variations in the drum type.................................................................... 24 2.5 Types of Biogas plant..................................................................................................... 24 2.6 Problems involved with Biogas production.................................................................. 26 2.7 Advantages and disadvantages of Biomass................................................................... 27 2.8 Factors affecting biogas generation............................................................................... 27 2.9 Thermo chemical conversion of biomass...................................................................... 29 2.10 Classification of Biomass gasifiers................................................................................ 30 2.10.1 Fixed bed gasifiers.................................................................................................. 30 2.10.2 Fluidized bed gasifier............................................................................................. 30 2.11 Gasification process........................................................................................................ 31 2.12 Applications of Biogas.................................................................................................... 32 2.13 Application of Biogas in Engines.................................................................................. 32 2.13.1 Advantages of Biogas............................................................................................. 33 2.13.2 Disadvantages of Biogas........................................................................................ 33 Department of Mechanical Engineering, RNS Institute of Technology. Page 2 of 34 Renewable Energy Power Plants (21ME652) Module 3 1 Wind Energy 1.1 Introduction, Properties of wind Wind results from air in motion. Air in motion arises from a pressure gradient. On a global basis one primary forcing function causing surface winds from the poles toward the equator is convective circulation. Solar radiation heats the air near the equator, and this low-density heated air is buoyed up. At the surface it is displaced by cooler denser higher-pressure air flowing from the poles. In the upper atmosphere near the equator the air thus tend to flow back toward the poles and away from the equator. The net results is a global convective circulation with surface winds from north to south in the northern hemisphere. It is clear from the above over simplified model that the wind is basically caused by the solar energy irradiating the earth. This is why wind utilization is considered a part of solar technology. In actuality the wind is much more complex. The above model ignores the earth's rotation which causes a Coriolis force resulting in an easterly wind velocity component in the northern hemisphere. There is the further complication of boundary layer frictional effects between the moving air and the earth's rough surface. Mountains, trees, buildings, and similar obstructions impair stream line air flow. Turbulence results, and the wind velocity in a horizontal direction markedly increases with altitude near the surface. Then there is the obvious fact of land and water with their unequal solar absorptivity’s and thermal time constants. During day light the land heats up rapidly compared to nearby sea or water bodies, and there tends to be a surface wind flow from the water to the land. At night the wind reverses, because the land surface cools faster than the water. Local winds are caused by two mechanisms. The first is differential heating of land and water. Solar isolation during the day is readily converted to sensible energy of the land surface but is partly absorbed in layers below the water surface and partly consumed in evaporating some of that water. The land mass becomes hotter than the water, which causes the air above the land to heat up and become warmer than the air above water. The warmer lighter air above the land rises, and the cooler heavier air above the water moves into replace it. This is the mechanism of shore breezes. At night, the direction of the breezes is reversed because the land mass cools to the sky more rapidly than the water, assuming a clear sky. The second mechanism of local Department of Mechanical Engineering, RNS Institute of Technology. Page 3 of 34 Renewable Energy Power Plants (21ME652) Module 3 winds is caused by hills and mountain sides. The air above the slopes heats up during the day and cools down at night, more rapidly than the air above the low lands. This causes heated air the day to rise along the slopes and relatively cool heavy air to flow down at night. It has been estimated that 2 per cent of all solar radiation falling on the face of the earth is converted to kinetic energy in the atmosphere and that 30 per cent of this kinetic energy occurs in the lowest 1000 m of elevation. It is thus said that the total kinetic energy of the wind in this lowest kilometer, if harnessed, can satisfy several times the energy demand of a country. It is also claimed that the wind power is pollution free and that its source of energy is free. Such are the seemingly compelling arguments for wind power, not unlike those for solar power. Although solar energy is cyclic and predictable, and even dependable in some parts of the globe, wind energy, however, is erratic, unsteady, and often not reliable, except in very few areas. It does, however, have a place in the total energy picture, particularly for those areas with more, or less steady winds, especially those that are far removed from central power grids, and for small, remote domestic- and farm needs. Conversion of the kinetic energy (i.e., energy of motion) of the wind into mechanical energy that can be utilized to perform useful work, or to generate electricity. Most machines for converting wind energy into mechanical energy consist basically of a number of sails, vanes, or blades radiating from a hub or central axis. The axis may be horizontal, as in the more familiar windmills, or vertical, as it is in some cases. When the wind blows against the vanes or sails they rotate about the axis and the rotational motion can be made to perform useful work. Wind energy conversion devices are commonly known as Wind turbines because they convert the energy of the wind stream into energy of rotation: the component which rotates is called the rotor. The terms turbine and rotor are, however, often regarded as being synonymous. Because wind turbines produce rotational motion, wind energy is readily converted into electrical energy by connecting the turbine to an electric generator. The combination of wind turbine and generator is sometimes referred to as an aero generator. A step-up transmission is usually required to match the relatively slow speed of the wind rotor to the higher speed of an electric generator. 1.2 Availability of wind energy in India In India the interest in the windmills was shown in the last fifties and early sixties. Apart from importing a few from outside, new designs were also developed, but it was not sustained. It is only in the last few years that development work is going on in many institutions. An important Department of Mechanical Engineering, RNS Institute of Technology. Page 4 of 34 Renewable Energy Power Plants (21ME652) Module 3 reason for this lack of interest in wind energy must be that wind, in India are relatively low and vary appreciably with the seasons. Data quoted by some scientists that for India wind speed value lies between 5 km/hr to 15-20 km/hr. These low and seasonal winds imply a high cost of exploitation of wind energy. Calculations based on the performance of a typical windmill have indicated that a unit of energy derived from a windmill will be at least several times more expensive than energy derivable from electric distribution lines at the standard rates, provided such electrical energy is at all available at the windmill site. The above argument is not fully applicable in rural areas for several reasons. First electric power is not and will not be available in many such areas due to the high cost of generation and distribution to small dispersed users. Secondly there is possibility of reducing the cost of the windmills by suitable design. Lastly, on small scales, the total first cost for serving a felt need and low maintenance cost are more important than the unit cost of energy. The last point is illustrated easily: dry cells provide energy at the astronomical coat of about Rs. 300 per kWh and yet they are in common use in both rural and urban areas. This raises the question of that the felt needs are that a windmill might satisfy while large scale energy production at some favorable sites in India is a possibility that needs to be explored, there appears to be a definite need for small sources of mechanical energy in rural areas. For example, even casual polls among villagers quickly reveal that their first concern is invariably water for drinking, washing and irrigation; and lifting water is a task which a windmill can perform. For such a task, a windmill should produce about 100 W, considering that a pair of bullocks, often used for lifting water in villages, typically provides about 250 W power. Many projects on windmill systems for water pumping and for production of small amount of electrical power have been taken up by various organizations, such as National aeronautical laboratory Banglore, central salt and Marine Chemicals Research Institute Bhavnagar, Central Arid Zone Research Institute (CAZRI)Jodhpur etc. Wind energy offers another source for pumping as well as electric power generation. India has potential of over 20,000 MW for power generation and ranks as one of the promising countries for tapping this source. The cost of power generation from wind farms has now become lower than diesel power and comparable to thermal power in several areas of our country especially near the coasts. Wind power projects of aggregate capacity of 8 MW including 7 wind farms projects of capacity 6.85 MW have been established in different parts of the country of which 3 MW capacity has been completed in 1989 by NES. Wind farms are operating successfully and have already fed over 150 lakh units of electricity to the respective state grids. Over 25 Department of Mechanical Engineering, RNS Institute of Technology. Page 5 of 34 Renewable Energy Power Plants (21ME652) Module 3 MW of additional power capacity from wind is under implementation. Under demonstration Programme 271 wind pumps have been installed up to February 1989. Sixty small wind battery chargers of capacities 300 watts to 4 kW are under installation. Like wise to stand-alone wind electric generators of 10 to 25 kW are under installation. 1.3 Basic Principles of Wind Energy Conversion 1.3.1 The Nature of the Wind The circulation of air in the atmosphere is caused by the non-uniform heating of the earth's surface by the sun. The air immediately above a warm area expands, it is forced upwards by cool, denser air which flows in from surrounding areas causing a wind. The nature of the terrain, the degree of cloud cover and the angle of the sun in the sky are all factors. which influence this process. In general, during the day the air above the land mass tends to heat up more rapidly than the air over water. In coastal regions this manifests itself in a strong onshore wind. At night the process is reversed because the air cools down more rapidly over the land and the breeze therefore blows off shore. The main planetary winds are caused in much the same way Cool surface air sweeps down from the poles forcing the warm air over the tropics to rise. But the direction of these massive air movements is affected by the rotation of the earth and the net effect is a large countries-clockwise circulation of air around low-pressure areas in the northern hemisphere, and clockwise circulation in the southern hemisphere. The strength and direction of these planetary winds change with the seasons as the solar input varies. Despite the wind's intermittent nature, wind patterns at any particular site remain remarkably constant year by year. Average wind speeds are greater in hilly and coastal areas than they are well inland the winds also tend to blow more consistently and with greater strength over the surface of the water where there is a less surface drag. Wind speeds increase with height. They have traditionally been measured at a standard height of ten meters where they are found to be 20--25% greater than close to the surface. At a height of 60 m they may be 30-60% higher because of the reduction in the drag effect of the earth's surface. 1.3.2 The Power in the Wind Wind possesses energy by virtue of its motion. Any device capable of slowing down the mass of moving air, like a sail or propeller, can extract part of the energy and convert is into useful work. Three factors determine the output from a wind energy converter: i. the wind speeds. Department of Mechanical Engineering, RNS Institute of Technology. Page 6 of 34 Renewable Energy Power Plants (21ME652) Module 3 ii. the cross-section of wind swept by rotor; and iii. the overall conversion efficiency of the rotor, transmission system and generator or pump. No device, however well-designed, can extract all of the wind's energy because the wind would have to be brought to a halt and this would prevent the passage of more air through the rotor. The most that is possible is for the rotor to decelerate the whole horizontal column of intercepted air to about one-third of its free velocity. A 100% efficient aerogenerator would therefore only be able to convert up to a maximum of around 60% of the available energy in wind into mechanical energy. Well-designed blades will typically extract 70% of the theoretical maximum, but losses incurred in the gearbox, transmission system and generator or pump could decrease overall wind turbine efficiency to 35% or loss. The power in the wind can be computed by using the concept of kinetics. The wind mill works on the principle of converting kinetic energy of the wind to mechanical energy. We know that power is equal to energy per unit time. The energy available is the kinetic energy of the wind. The kinetic energy of any particle is equal to one half its mass times the square of its velocity, or 1 mV2. The amount of air passing in unit time, through an area A, with velocity V, is A.V, 2 and its mass m is equal to its volume multiplied by its density ρ of air, or = (m is the mass of air transversing the area A swept by the rotating blades of a wind mill type generator). Substituting this value of the mass in the expression for the kinetic energy, we obtain i e i e e =1 2 watts 2 i ei e e = 1 3 watts 2 Department of Mechanical Engineering, RNS Institute of Technology. Page 7 of 34 Renewable Energy Power Plants (21ME652) Module 3 Above equation tells us that the maximum wind available the actual amount will be somewhat less because all the available energy is not extractable-is proportional to the cube of the wind speed. It is thus evident that small increase in wind speed can have a marked effect on the power in the wind. Equation also tell us that the power available is proportional to air density (1.225 kg/m3 at sea level). It may vary 10-15 percent during the year because of pressure and temperature change. It changes negligibly with water content. Equation also tells us that the wind power is proportional to the intercept area. Thus an aero turbine with a large swept area has higher power than a smaller area machine but there are added implications. Since the area is normally circular 2 of diameter D in horizontal axis aero turbines, then = D 4 Available wind power = 1 2 3 2 4 1 = 2 3 8 1.4 Major problems associated with wind power The two major disadvantages of wind power include initial cost and technology immaturity. Firstly, constructing turbines and wind facilities is extremely expensive. The second disadvantage is technology immaturity. High cost of energy can, in part, be addressed directly with technology innovations that increase reliability and energy output and lower system capital expenses. Offshore wind energy produces more energy than onshore wind energy, but costs much more to establish. The primary costs of wind turbines include construction and maintenance. New technology is needed to lower costs, increase reliability and energy production, solve regional deployment issues, expand the resource area, develop infrastructure and manufacturing facilities, and mitigate known environmental impacts. Therefore, one may argue that implementation of wind energy must be delayed until technological advancements are made. Other Problems include: 1. Aesthetic impact: Many people are concerned with the visual effects that wind turbines have on the beautiful scenery of nature. They believe that giant wind turbines distract viewers from the beautiful surroundings. 2. Wildlife: Wind turbines may be dangerous to flying animals. Many birds and bats have been killed by flying into the rotors. Experts are now conducting research to learn more about the effects that wind turbines have on marine habitats. Department of Mechanical Engineering, RNS Institute of Technology. Page 8 of 34 Renewable Energy Power Plants (21ME652) Module 3 3. Remoteness of location: Although this may be an advantage (placing wind turbines in desolate areas, far away from people), it may also be a disadvantage. The cost of travel and maintenance on the turbines increases and is time consuming. Offshore wind turbines require boats and can be dangerous to manage. 4. Noise: Some wind turbines tend to generate a lot of noise which can be unpleasant 5. Safety at Sea: In the darkness/at night it may be difficult for incoming boats to see wind turbines thus leading to collisions. 1.5 Aerodynamic consideration in wind turbine The basic requirement in the design of a wind turbine, it must utilize maximum energy available with the wind. This totally depends upon the blade shape which is fully responsible for extracting maximum energy from the wind. Therefore, it is necessary to understand the basic principle of aerodynamic theory when the wind is flowing over the blade surface area. 1.5.1 Terms Associated with Aerofoil It is necessary to be familiar with the terms associated with aerofoil section as shown in Fig. before going to analysis of the system 1. Aerofoil. It is a streamlined curved surface designed for the air to flow over it in order to produce high lift force and low drag force. 2. Angle of Attack. It is the angle between the chord of the aerofoil and relative air flow direction. 3. Leading and trailing edges of the aerofoil. It is the front edge of the blade towards the wind flow direction and trailing edge is the rear edge of the blade. 4. Chord-line. It is a straight line joining leading edge and trailing edge of the blade 5. Mean-line. It is line which is at equidistance between upper and lower surfaces of the blade at all points. 6. Chamber. It is the maximum distance between the mean line and chord. 7. Tip speed ratio (2). It is the ratio of the blade tip velocity to the undisturbed wind velocity (V) 8. Pitch angle. It is the angle made by the chord to the plane of blade rotation. A system used to change the pitch angle according to the wind speed is known as pitch control system. It is necessary for the efficient operation of the system. 9. Swept Area. It is the area covered by the rotating rotor. 10. Solidity (o). It is the ratio of the blades area to the swept area. Department of Mechanical Engineering, RNS Institute of Technology. Page 9 of 34 Renewable Energy Power Plants (21ME652) Module 3 11. Drag and Lift Forces. Drag force is the component of the force along the velocity of the wind and lift force is the component perpendicular to drag force. 12. Cut-in and cut-out speeds. Cut-in is the wind speed at which wind-turbine starts operation and cut-out wind speed at which the turbine stops operating for the safety. Rated speed of the wind mill is that speed at which the turbine provides maximum power. 1.5.2 Aerodynamic forces on aerofoil blades Presently, wind turbines designed for electric generation are built with a propeller type rotor on a horizontal axis with two or three blades. When the air-flows over the blades, it creates two forces known as lift (responsible for blade rotation) and drag which creates friction over the blade surface. For efficient blade, lift force must be highest possible and drag force must be minimum possible. As the wind flows over the aerofoil, it is accelerated over the upper surface and deaccelerated below it. This creates higher pressure at the bottom and lower pressure at the top and the effect is to create a resultant upward force known as lift force (FL) which is responsible for the rotation of the blade. Every lift force is associated with drag force (FD) but it is minimum with streamlined bodies. In case of wind-turbines, the top surface of the aerofoil is made curved and bottom surface nearly flat. The air flowing over the top surface travels a longer distance to reach the top end of the aerofoil compared with the air flow under the aerofoil. This creates a pressure difference Department of Mechanical Engineering, RNS Institute of Technology. Page 10 of 34 Renewable Energy Power Plants (21ME652) Module 3 which is responsible for lift as mentioned earlier. The ratio of (FL/FD) is as high as 30 for a good designed aerofoil. The values of F L and FD are 2 = 2 2 = 2 CL, and CD are known as lift and crag coefficients for a given blades. 1.6 Types of wind machines and their characteristics 1.6.1 Horizontal Axis Turbines These are very commonly used for converting wind energy into electrical energy. These a further classified as 2-blades, 3-blades and multiblade machines. 1.6.1.1 Two blade-Rotor. These are used for electric power generation and used in the range 1 MW to 3 MW. They are subjected to large vibrations during running. A teething-cont is provided to this machine to reduce the fatigue to the main shaft. This is because the bla in upper position experiences greater force than blade at lower position. The tip speed ratio of this rotor is 33% higher than 3- blade machines. 1.6.1.2 3-Blade Rotor. This rotor is commonly used as its efficiency is higher than two-blade rotor but the cost is 30 - 40% high. It is used from 15 kW to 5 MW capacity range. A small scale machine of this type is roof top mounted for generating the power to run all electrical equipments in the house. Small size rotor of 2 m diameter is generally used to produce 800-1000 watts power. These Department of Mechanical Engineering, RNS Institute of Technology. Page 11 of 34 Renewable Energy Power Plants (21ME652) Module 3 units are provided with wind-vane at tail which makes the rotor to come inline with wind direction. 1.6.1.3 Multiblade Rotor. This rotor has high solidity factor and generally used for water pumping purposes. This is because of high starting torque-characters which is needed for water pumping. This rotor has low tip speed ratio and therefore less noisy. The main disadvantage is less efficient. 1.6.1.4 Up-ward and Down-ward rotors. The rotor is also classified as upward and downward machine. In upward machine the rotor is located in front of towar whereas it is located behind the tower Downward machine allows the use of free yaw-system and also allows the blades to deflect away from the tower when loaded. But, it suffers from wind-shadow-effect of tower on the blades (in region of separated flow). On the other hand, upward machine produces higher power as it eliminates the effect of tower shadow on the blades. This also results in lower noise, lower fatigue on the blades and smoother power output. Department of Mechanical Engineering, RNS Institute of Technology. Page 12 of 34 Renewable Energy Power Plants (21ME652) Module 3 1.6.2 Vertical Axis Wind-Mill In this system, the rotor is mounted on the vertical shaft. The types of the blades mounted are of different configurations as shown in Fig. Among all, two configurations described beloware commonly used. (a) Primary Drag type 1.6.2.1 Savonious wind mill. The rotors of these turbines are S-type in shape and supported at top and bottom by two circular plates as shown in Fig. The two curved blades are fixed on central pipe and free to rotate. The air strikes on concave side of the blade, rotate through centre of rotor and glides over the convex surface of the other blade. This turbine suffers from the disadvantage that when the convex surface faces the wind, the speed of the turbine slows down. This effect is eliminated by providing 4-blades so that one concave surface will always be there to allow the air-flow irrespective of wind direction. These machines are used for pumping water and grinding mills because of low speed (100 RPM). They are not preferred for electric power generating purposes because of low speed. Department of Mechanical Engineering, RNS Institute of Technology. Page 13 of 34 Renewable Energy Power Plants (21ME652) Module 3 The advantages of this machine are: 1. Low speed wind is successfully used. 2. Pitch and yaw-controls are not required. 3. Generator can be mounted on the ground which further simplifies the tower design. The noise is more as well as its power density is lower compared with horizontal axis and mills. 1.6.2.2 Darrieus wind-mill. This is the only vartical axis machine used successfully on commercial basis. It is named in the honor of French engineer Georges Darrieus. This type of turbine is shown in Fig. It consists of 2 or 3 thin curved aerofoil section, C shaped blades. Both the ends of the blades are fixed to the vertical shaft. The rotor rotates on two bearings placed at top and bottom of the pipe. It looks like an egg-beater and operates with the wind coming from any direction. This type of wind mills are available in the range of 4 to 14 MW capacity and with an efficiency of 35 - 40%. The major advantages of this machine are, the generator can be mounted on the ground and it does not require yaw mechanism to turn the rotor as per wind direction. The major disadvantages of this machine are, the machine is not self starting and therefore requires some power source to start, the shapes of the blades are difficult to manufacture, install and costly. These turbines suffer from a problem of stalling when the wind speed is high. 1.7 Advantages and disadvantages of wind energy 1.7.1 Advantages 1. The electrical energy generation is totally pollution free. 2. It is renewable form of energy and available free of cost. 3. It is more suitable in rural, offshore and onshore areas. 4. It is reliable and cost effective for large capacity units. Department of Mechanical Engineering, RNS Institute of Technology. Page 14 of 34 Renewable Energy Power Plants (21ME652) Module 3 5. Wind does not require any transportation. 6. It does not require any water like thermal and nuclear power plants. 7. The operating cost is considerably low. 8. This plant does not require any regular man power. 9. It is economically competitive with other energy sources. 1.7.2 Disadvantages: 1. The power output is not uniform and some back-up is required. This system requires storage batteries. They are very far away from the cities and load centers. 2. Wind power density (W/m2) is very low. 3. The wind-rotor efficiency is low (10-40%). 4. Capital cost (Rs/kW) for small unit is considerably high. 5. The weight of this system (kg/kW) is high compared with conventional system. 6. Wind energy generates noise pollution. 7. It requires large open area. 8. As it is located far away from the point of utilization, therefore transmission losses are large if connected to main grid. 9. It is observed that big wind farms change the rainfall pattern which is not desirable for crops. 10. The windmill creates interference when placed between radio and television stations as it reflects electromagnetic radiation. It is also hazardous for civil aviation. 1.8 Problems in operating large wind power generators 1. Location of site: The most important factor is locating a site big enough which has a reasonable average high wind velocity. Sourashtra and Coastal Regions in India are promising areas. 2. Constant angular velocity: A constant angular velocity is a must for generating A.C power and this means very sensitive governing. 3. Variation in wind velocity: The wind velocity varies with time and varies in direction and also varies from the bottom to top of a large rotor. This causes fatigue in blades. 4. Need of storage system: At zero velocity conditions, the power generated will be zero and this means some storage system will have incorporated along with the wind mill. 5. Strong supporting structure: Since the wind mill generator will have to be located at a height, the supporting structure will have to be designed to withstand high wind velocity and impacts. This will add to the initial costs of the wind mill. Department of Mechanical Engineering, RNS Institute of Technology. Page 15 of 34 Renewable Energy Power Plants (21ME652) Module 3 6. Occupation of large areas of land: large areas of land will become unavailable due to wind mill gardens. The whole area will have to be protected to avoid accidents. Inspite of all these difficulties, interest to develop wind mills is there since this is a clean source of energy. Department of Mechanical Engineering, RNS Institute of Technology. Page 16 of 34 Renewable Energy Power Plants (21ME652) Module 3 2 Energy from Biomass Biomass is a scientific term for living matter, but the word biomass is also used to denote products derived from living organisms - wood from trees, harvested grasses, plant parts and residues such as twigs, stems and leaves, as well as aquatic plants and animal wastes. All the Earth's biomass exists in a thin surface layer called the biosphere. This represents only a tiny fraction of the total mass of the Earth, but in human terms it is an enormous store of energy - as fuel and as food. More importantly, it is a store which is being replenished continually. The source which supplies the energy is of course the Sun, and although only a tiny fraction of the solar energy reaching the Earth each year is converted into biomass, it is nevertheless equivalent to over five times total world energy consumption. The plants which are grown on land (terrestrial) and aquatic (grown in water) and their derivatives, results in the formation of organic matter called "Biomass". The forest crops, animal manure etc all comes under Biomass. As plant lite renews and adds to itself every year. Biomass is a type of renewable source of energy. It is considered as indirect form of solar energy. It is the way of harnessing solar energy by photosynthesis. We can write Solar energy > photosynthesis > Biomass > energy Biomass energy or "bio-energy" includes any solid, liquid or gaseous fuel, or any electric power or useful chemical product derived from organic matter; whether directly from plants or indirectly from plant-derived industrial, commercial, or urban wastes, or agricultural and forestry residues. Thus bio-energy can be derived from a wide range of raw materials and produced in a variety of ways. Because of the wide range of potential feed stocks and the variety of technologies to produce them and process them, bio-energy is usually considered as a series of many different feedstock/ technology combinations. If biomass is cultivated and harvested in a way that allows re growth without depleting nutrient and water resources, it is a renewable resource that can be used to generate energy on demand, with little net additional contributions to global "Green house gas" emissions The Biomass resources are grouped into: 1. In the form of solid mass (wood and agricultural waste) which could burn to release energy directly 2. In non traditional form (liquid fuels) in which ethanol (ethyl alcohol) and methanol (methyl alcohol) are used as fuels to run the engines. 3. In the form of gaseous fuel called biogas. Department of Mechanical Engineering, RNS Institute of Technology. Page 17 of 34 Renewable Energy Power Plants (21ME652) Module 3 The energy density of biomass in liquid and gaseous form is high. The anaerobic decomposition of the organic matter results in the formation of biogas or methane. This gas is produced from cow dung and other wastes. The methane gas is also produced by aquatic biomass. If the mixture contains 7 to 9% solid matter, the gas generation rate will be higher. The mixture of biogas and diesel oil is used to run diesel engine to reduce diesel consumption. Traditional bio energy is in the form of fuel wood, char coal and residues. The dry biomass like wood and straw could be burnt to release energy by direct combustion. The wet biomass contains moisture content like sewage sludge and vegetable matter and is to be dried and burnt. The moisture content of the biomass decreases value of the biomass as fuel. Usually, the wet organic matter or biomass is converted in to premium fuels by digestion or fermentation. The biomass fuels are available in the form of solid, liquid and gaseous fuels: 1) Solids: The solid biomass fuels are available in the form of wood, straw, municipal refuse etc. Wood has low ash and Sulphur content and is used for domestic heating and in timber and furniture industries. Straw burning stoves are used for crop drying, space and water heating and other similar applications. The energy content of the municipal refuse is very low and can be used to generate heat and power. 2) Liquids: The liquid biofuels considered for power generation are alcohols, vegetable oil, hydro carbons from Euphorbia plants etc. Mainly methanol and ethanol are used as fuels in I C engines to replace petrol and diesel. The octane rate is high, but calorific value is less. The vegetable oils used as bio fuels are sun flower and rape seed oil peanuts oil, palm oil, Soya and corn oil etc.., These vegetable oils may be directly burnt to release energy or may be blended with other fuels like diesel and releases energy. 3) Gases: The biogas is a mixture of methane and carbon dioxide and is generated from cow dung and agricultural wastes. The percentage of methane ranges from 50 to 70 % The carbon dioxide may be removed from biogas to increase the fuel quality. biogas can be used for water heating, space heating and cooking purposes. The Biomass gasifies were used as fuel for transport vehicles during 1920 - 1930s in war and Rudolf diesel used Peanut oil as fuel in car engines in 1893. Estimates of how much of the Earth's land-based production is used by the human population worldwide range from a low figure of about 5% to a high of over 30% (this includes food, animal fodder, timber and other products, as well as bioenergy). Biomass energy use worldwide Department of Mechanical Engineering, RNS Institute of Technology. Page 18 of 34 Renewable Energy Power Plants (21ME652) Module 3 been independently estimated at about 55 hex joules per year, or about 2% of annual biomass production on land. Worldwide, biomass is the fourth largest energy resource after coal, oil, and natural gas estimated at about 14% of global primary energy (and much higher in many developing countries). In the U.S., biomass today provides about 3-4% of primary energy (depending on the method of calculation). Biomass is used for heating (such as wood stoves in homes and for process heat in bio processing industries), cooking (especially in many parts of the developing world), transportation (fuels such as ethanol) and, increasingly, for electric power production. Installed capacity of biomass power generation worldwide is about 35,000 MW with about 7,000 MW in the United States derived from forest-product-industry and agricultural residues (plus an additional 2,500 MW of municipal solid waste-fired capacity, which is often not counted as part of biomass power, and 500 MW of landfill gas-fired and other capacity). Much of this 7,000 MW capacity is presently found in the pulp and paper industry, in combined heat and power (cogeneration) systems. 2.1 Photosynthesis It is the biological con version of sun's radiant energy in to sugars and starches which are rich energy compounds. The green pigment chlorophyll of the plant absorbs sun's energy and it is stored in the form of chemical bond energy. It is possible to harvest and burn the plants with high photosynthesis efficiency to generate steam which could be used to generate electricity as in thermal power plants. It is the best way of harnessing solar energy and is a renewable resource. In plants, solar energy con version is only about 1% and overall efficiency of con version of sunlight to electricity is 0.3%. Hence in comparison with photovoltaic cells, which have 10% efficiency, this concept is less attractive. 2.1.1 Photosynthetic oxygen production Photosynthesis is a complex process in which water and CO, molecules are broken down in sun light and releases carbohydrate and pure oxygen. CO2 + H2O + sun light + chlorophyll --› (H2CO)6 + O2 + chlorophyll 6CO2 + 12 H20 --> C6H12 O6 + 6H2O + 6O 2 The absorbed light is in the ultraviolet and infrared range. The chlorophyll absorbs visible light and passes its energy on to the water molecules and releases a hydrogen atom. The hydrogen atom thus produced reacts with CO2 molecule to produce H2CO and O2 At high temperature Department of Mechanical Engineering, RNS Institute of Technology. Page 19 of 34 Renewable Energy Power Plants (21ME652) Module 3 H2CO breaks to release energy. H2CO + O2 --> CO2+ H2O + 112 Kcal / mol of energy. By growing algae in plastic tubes or ponds, we can produce large amount of carbohydrates. The heat could be generated by burning algae and then could be used to generate electricity by conventional methods. Thus, photosynthesis is a reduction and oxidation process which produces carbohydrates such as sugar in the green leaf. The O2 is liberated from H 2O molecule. Mainly the photosynthesis consists of two steps: 1) Due to action of chlorophyll and sunlight, water molecule breaks in to H, and O, This phase is called light reaction in which solar energy is converted in to potential chemical energy. The O, escapes and H, gets converted into some unknown compound. 2) This phase of reaction is called dark reaction which forms CO, and starch or sugar from unknown compound of H, and does not require sunlight. 2.2 Energy Plantation It is a means of extracting maximum solar energy by growing plants. The plants are grown, especially for their fuel value and acts as solar collectors. They are economical, free from pollution and require no maintenance. Energy farms are the best alternatives for present fuel crisis and to replace fossil and nuclear energy sources. In plant farm, the natural photosynthesis process stores ten times more energy annually than consumed by the world. In India, the total forest area is around 25%. Jojaba, Acacia, Tortilla, Albizzia, Lebbak, Prasois, Juliflora are some of the tree species which have been identified for energy farm in our country. Indian Institute of Science, Bangalore running a program to grow monoculture plantations of fast growing species and monitor the biomass productivity. They also studied the economics of monoculture plantations and developed an alternative forestry strategy to meet various village needs. Energy farm is a locally available energy source with highest versatility among renewable energies. No other energy source can open such new opportunities for agricultural and forest development. A number of projects have been taken up to full fill the needs of fuel and power generation. A number of projects, worth about 5M W capacity, have been taken under DNES (Development of Non-conventional Energy Sources) at various places in the country. Gasifiers and sterling engines are used for generation of energy from various types of biomasses. Department of Mechanical Engineering, RNS Institute of Technology. Page 20 of 34 Renewable Energy Power Plants (21ME652) Module 3 2.3 Biogas generation The decomposition of animal, plant and human wastes generates biogas and is a mixture of methane (50 to 70%), carbon dioxide (30 to 40%), hydrogen, hydrogen sulphide and nitrogen. The biogas is a clean, slow burning gas with its calorific value ranging from 21000kJ/kg to 23028 kJ/kg (38131kJ/m3). Biogas is mainly used for cooking applications. The materials used for biogas generation retains its fertilizer properties and return to the soil. The biogas is generated from cow dung, piggery waste, poultry droppings, algae, crop residues, garbage kitchen wastes etc. The cellulosic organic material of animal or plant origin forms raw material with high potential for biogas generation. There are three methods by which biogas could be generated. They are digestion, pyrolysis or hydro gasification. Digestion is a biological process which occurs in a chamber called digester. The process occurs in the presence of anaerobic organisms at atmospheric pressure and temperatures of 35 - 70°C and in the absence of oxygen. 2.3.1 Anaerobic digestion It is the method of generating biogas through fermentation or bio digestion of different types of wastes by a number of anaerobic and facultative organisms. Facultative organisms are bacteria which grow with or without oxygen. Bacteria are classified in to two groups, aerobic which grow in presence of oxygen and anaerobic - does not require oxygen to grow (The biodegradation or decomposition of the organic matter by fermentation process through anaerobic digestion, results in the formation of biogas. The anaerobic digestion produces sugar, alcohols, pesticides and amino acids by breaking organic matter. This results in the formation of methane by another type of bacteria. The phases of anaerobic digestion are: 1. Enzymatic hydrolysis: In this phase, the fats, starches and proteins present in the cellulose biomass are converted in to simple compounds. 2. Acid formation: In this phase, the complex organic compounds converted in to simple organic acids. The acids and volatile solids are formed by hydrol and fermentation from microorganism of facultative and anaerobic group and together called acid formers. This stage may last about two weeks and this phase results in the formation of large amount of carbon dioxide. 3. Methane formation: In this phase, the acids produced from previous phase converted Department of Mechanical Engineering, RNS Institute of Technology. Page 21 of 34 Renewable Energy Power Plants (21ME652) Module 3 in to methane (CH4) and CO2 by anaerobic bacteria which are also known as methane fermenters. For digestion process to be efficient, these acid formers and methane fermenters must be in a state of dynamic equilibrium. The variation in pH value, will affect the methane formers as they are sensitive to pH variations. For fermentation and biogas generation, a pH value of 6.5 to 8 is suitable. The anaerobic digestion is represented by the general equation CxH yO 2 + [x-y/4-2/2]H20 --> [x/2-y/8+z/4]CO2 + [x/2+y/8-z/4]CH4 For cellulose, (C6H10 O5)n + nH2O -› 3nCO2 +3nCH4 It is also to be noted that, the digestion at high temperature is faster than that at lower temperature. For every 5°C raise in temperature, the gas yield rate increases twice. The temperature ranges that influence the bacteria are Psicrophilic, about 20°C Mesophilic, about35°C Thermophilic, about 55°C In tropical countries, the digesters are operating in psichrophilic range. The digesters are heated in cold climates by using a part of biogas output and temperature ranges up to 35°C. Some digesters work at 55°C, to digest material. Advantages of Anaerobic digestion 1) The anaerobic digestion produces biogas which has a calorific value. Hence this gas could be successfully used to produce steam or hot water. 2) A smaller quantity of excess sludge is produced during anaerobic digestion of organic matter. 3) The running cost is very less when compared to equivalent aerobic system 4) The odor is less 5) The use of biogas in industries reduces the consumption of coal and also reduces air pollution. 6) The nutrient requirement is less due to low production of bacterial solids. 2.4 Classification of biogas plants Biogas plants are classified in to Department of Mechanical Engineering, RNS Institute of Technology. Page 22 of 34 Renewable Energy Power Plants (21ME652) Module 3 1) continuous and batch types 2) The dome and drum types 3) Different variation in the drum type 2.4.1 Continuous and batch type a) Continuous plant: In this type, the raw material is fed in to a single digester and the process is carried out without interruption. The process may be completed in a single stage or two stages i) Single stage process: The raw material is fed to a single chamber in which organic matter is converted to biogas. The sludge is removed from the chamber continuously. ii) Two stage process: This type consists of two chambers. The acid formation is carried out in one chamber and bio-methanation is carried out in a separate chamber. The biogas is generated in the second chamber. In the continuous plant, the size of the digester is small and takes lesser time for digestion process. The biogas generation is continuous and encounters lesser problems compared to batch type. b) The batch plant: In this type, after digestion process, the digester is emptied. The urea lime etc., are fed in to a number of digesters which produces gas for 40to 50 days. As these digesters are charged and emptied one by one in a synchronous manner, the gas will be continuously supplied through a common gas holder. The system uses a number of digesters and gas generation is intermittent. It is expensive and encounters more problems. 2.4.2 The dome and the drum types: Mainly two types of biogas plants are used 1. The floating gas holder plant 2. Fixed dome digester In India, the first type is known as KVIC plant and the later type is called Chinese plant. A vertical or horizontal digester may be used. In both the designs, cylindrical, rectangular and spherical shapes are used. In floating gas holder plant, the gas holder is free from digester whereas in fixed type, the gas holder and digester are combined. Department of Mechanical Engineering, RNS Institute of Technology. Page 23 of 34 Renewable Energy Power Plants (21ME652) Module 3 2.4.3 Different variations in the drum type: The floating design is either provided with water seal or without water seal. The plant works as anaerobic digester with water seal and this design reduces corrosion of the gas holder drum. The materials of gas holder and digester are also varied. Usually bricks and stones are used. In latest designs of gas holder, fiber glass reinforced plastic is used. 2.5 Types of Biogas plant As discussed earlier, the biogas plants are classified into 1) floating gas holder plant and 2) fixed dome digester plant. In India, biogas plants are again classified into K VIC (Khadi Village Industries commission) model and Janata model. The KVIC model biogas plant shown in figure uses floating gas holder design. A masonry well contains raw material slurry. The digestion occurs in this well and the gas formed will be collected and taken out from the top. As the drum floats, this design is called floating gas holder type. Department of Mechanical Engineering, RNS Institute of Technology. Page 24 of 34 Renewable Energy Power Plants (21ME652) Module 3 The fixed dome digester plant is also known as Janata Model or Chinese plant and is similar to KVIC plant except that the masonry fixed'dome replaces floating steel drum. The basic components of this model are. Foundation: The foundation is made of cement, concrete and brick ballast. The plant should provide stable foundation to the digester walls and should bear full load of slurry filled in the digester. In order to avoid water leakage, the foundation is made water proof. Digester: The digester is a cylindrical chamber made of bricks, sand and cement constructed under the ground where fermentation of dung takes place. The slurry flows in and out of digester through rectangular openings provided as shown in figure. Dome: The roof the digester is shaped hemispherical and is called Dome and it is of fixed type. The gas collected in the dome space exerts pressure on the slurry in the digester. Inlet chamber: It is made of bricks, cement and sand and is provided with an opening at ground level. A sloppy wall at the outlet ensures easy movement of the cattle dung to the digester. Outlet chamber: The digested slurry moves out of the digester at a pre-determined height through outlet chamber. A small rectangular opening is provided at the bottom and larger area Department of Mechanical Engineering, RNS Institute of Technology. Page 25 of 34 Renewable Energy Power Plants (21ME652) Module 3 is provided at the top to a pre-defined height. It is also provided with opening at the ground level. The digested or spent slurry enters a composite pit through this opening. Mixing tank: It is the chamber in which slurry is formed. The slurry is then enters into the inlet chamber. Gas out let pipe: The Dome is fitted with a gas pipe to con vey the gas to use. The gas flow is regulated by valve provided at the end of gas pipe. Slurry is prepared by thorough mixing of dung and water in the ratio of 1:1 in a mixing tank. Then the slurry is fed to the digester to the required level and then fermentation begins. In the initial stage, the gas is to be allowed to escape as it contains CO,, O, and H,S in large quantities (which will not burn). After this stage, the gas released will consists of right combination of methane (60%) and CO, (40%). At this stage, the plant is to be supplied with dung slurry. Working The slurry of dung and water is filled in the digester up to the level of cylindrical portion. The gas generated due to fermentation gets accumulated in the dome and exerts pressure on the slurry. The slurry in the digester pushed to inlet and outlet chambers. Hence slurry level falls in digester and rises in outlet chamber. The slurry level continues to fall till the level reaches upper edges of inlet and outlet gates. Thereafter, the gas accumulation would exert pressure and gas escapes to atmosphere through gates. The bubbling and froth formation of the slurry in inlet and outlet chambers indicates escape of gas to atmosphere. By knowing increase in slurry volume in these chambers, the quantity of usable gas can be calculated. When gas is supplied for usage, the slurry level in the digester increases and that of in inlet and outlet chamber decreases. During the process, an equivalent amount of digested slurry is discharged from outlet chamber and the fresh slurry enters and settle down in the digester. 2.6 Problems involved with Biogas production 1. Handling of effluent slurry is a major problem and sufficient open space is to be provided to dry the slurry. It also requires human animal labor to carry effluent to the field. 2. The methanogenic bacteria involved in gas generation are very sensitive to temperature and affects the gas generation rate. 3. Due to lack of knowledge about Biogas generation, some persons add urea fertilizer which results in toxicity of ammonia nitrogen and decreases gas production. Department of Mechanical Engineering, RNS Institute of Technology. Page 26 of 34 Renewable Energy Power Plants (21ME652) Module 3 4. When cattle dung and water is not properly mixed, volatile fatty acids will be accumulated and results in failure of digester. 5. It is necessary to maintain an optimum range of PH and volatile fatty acids, otherwise digester will not work properly. 6. There is chance of leakage of gas from gas holder in case of Janata Model 2.7 Advantages and disadvantages of Biomass Advantages 1. The initial investment is low and costlier equipment are not used. 2. The use of Biomass as fuel reduces environmental hazards. 3. The technology is best suited for rural areas of developing countries like India. 4. The byproducts can be fully recycled. 5. Less polluting, suitable for domestic purposes. 6. Easily transportable to consumers Disadvantages 1. The plant uses larger land area. 2. The efficiency of biological energy con version is very less (0.1%). 3. In centralized power generation system, the cost of energy production is higher. 4. Collection and transportation of biomass is expensive. 5. When compared to LPG cylinders, it is difficult to store the gas, as it cannot be liquified ordinarily. 2.8 Factors affecting biogas generation 1. PH or hydrogen ion concentration: In the digester, a suitable PH range is to be maintained to provide constant supply of the gas. In a PH range of 6.5 to 7.5, microorganisms will be very active and bio-digestion will be very efficient. The addition of some material to the digester causes variations in the PH value and results in the imbalance of bacteria population. For sewage solids, the ideal PH is from 7 to 7.5. 2. Temperature: The temperature ranges from 35°C to 38°C results in better methane formation. The gas generation starts decreasing at 20°C and ceases completely at 10°C. 3. Total solid content of feed material: In order to get total solid content of 8 to 10%, the cow dung is to be mixed in the range of 1:1 by weight. Around 80-82% of moisture Department of Mechanical Engineering, RNS Institute of Technology. Page 27 of 34 Renewable Energy Power Plants (21ME652) Module 3 is present in raw cow dung and remaining 18-20% is called total solids. The adjustment made in total solid content increases the bio-digestion rate. 4. Loading rate: The amount of raw material supplied to the fermentation tank (digester) per day per unit volume is known as loading rate. For municipal sewage treatment plants, the loading rate ranges from 0.5 to 1.6 kg/m/day. The optimum range of loading rate ranges from 1.2 to 5.3 kg/m°/day. High loading rate, results in the formation of acids and thus fermentation stops. 5. Seeding: Seeding is nothing but the increase in number of methane formers by artificial means. It uses digested sludge which is rich in methane formers. But higher seeding is also not desirable as gas production decreases beyond certain limits due to reduction of total solid contents of the cow dung. 6. Uniform feeding: In order to provide good fermentation in the digester, a control over quality and quantity of raw material supplied to the digester is essential. Therefore, all the time uniform feeding of digester is necessary. 7. Carbon nitrogen ratio of the input material: For an optimal digestion rate, a carbon nitrogen ratio of 30: 1 is necessary. High carbon in raw material slows down the digester. High nitrogen content of the raw material may stop the fermentation process. The ammonia formed due to nitrogen and hydrogen may kill methane producers. 8. Diameter to depth ratio: It was investigated that the maximum gas production rate occurs with diameter to depth ratio of 0.66 to 1.0. But the effect of temperature at different depths also plays important role is deciding this ratio. 9. Nutrients: In digester, the bacteria always require C, H2, O2, P and S. Out of these nutrients, the supply of N2 and P are always short. In order to compensate this, extra raw material which is rich in phosphorus and N, must be added to increase the gas generation rate. 10. Mixing or stirring or agitation of content of digester: In digester, a proper mixing of slurry is required to improve the fermentation process. Slight mixing results in good fermentation and the digestion may be retarded due to violent agitation. 11. Retention period or feeding rate: The temperature and feed stocks influence the retention period of the material for biogas generation. Usually, the retention period is kept from 30 to 45 days. 12. Pressure: The fermentation process is also influenced by the pressure acting on the slurry surface. Lower pressure gives better fermentation process. Department of Mechanical Engineering, RNS Institute of Technology. Page 28 of 34 Renewable Energy Power Plants (21ME652) Module 3 13. Acid accumulation inside the digester: When fresh raw material is supplied in excess, the PH value decreases due to the formation of acids. The addition of neem cake produces methane from these acids. 2.9 Thermo chemical conversion of biomass In bio chemical energy conversion, the action of bacteria results in the formation of biogas. In thermo chemical conversion of biomass, its temperature is raised and processes like pyrolysis, combustion and gasification occurs depending on amount of oxygen supplied. The example for combustion is burning of biomass in open fire and stoves. In this case, oxygen is supplied in excess of theoretically required. When less O2 is supplied, pyrolysis and gasification occur. The conversion of wood and incineration of solid waste is an example of pyrolysis. A gas mixture consisting of carbon monoxide and hydrogen [producer gas] is generated at some temperature and O2 supply. The formation of gaseous mixture of carbon monoxide and hydrogen is called thermal gasification. The conversion of solid fuel in to producer gas by undergoing a series of thermo chemical processes like drying, pyrolysis, oxidation and reduction is known as thermal gasification. Carbon monoxide, hydrogen and oxygen are the main constituents when air is used as gasification agent. A typical gas composition is as follows, Carbon monoxide 18-22% Hydrogen 13-19% Methane 1-5% Hydrocarbons (heavier) 0.2-0.4% Carbon dioxide 9-12% Nitrogen 45-55% Water vapour 4% The dual fuel engines and diesel engines uses this gas as working fluid with some design changes. Department of Mechanical Engineering, RNS Institute of Technology. Page 29 of 34 Renewable Energy Power Plants (21ME652) Module 3 2.10 Classification of Biomass gasifiers A chamber through which oxygen or air required for combustion is passed, known as gasifier. The gasifier converts solid fuel into producer gas by undergoing a series of thermos chemical processes. Gasifiers are classified into 1. According to direction of gas flow: down draught, up draught and cross draught generator. 2. According to generation capacity: small size up to 10 kW, medium size for outputs from 10k W to 50k W, large size from 50 to 300 kW and very large above 300 kW output. 3. According to type of bed: fixed bed and fluidized bed. 2.10.1 Fixed bed gasifiers There are three main designs of fixed bed type: 1. Up draught type: In this type, air entry is below combustion zone and producer gas escapes from top of gasifier. It is suitable for char-coal, in stationary engines. 2. Down draught gasifier: In this type, air entry is at combustion zone and gas escapes through bottom of gasifier. These are suitable for wood and agricultural wastes. 3. Cross draught gasifiers: The gasifier contains charcoal which acts as insulator and dust filter. The gas flows around the gasifier in the annular space. 2.10.2 Fluidized bed gasifier: The use of this type allows gasification of any biomass. It burns any combustible material with high efficiency and less pollution. The inert particles burn in suspension, fluidized by air current flowing upward. Department of Mechanical Engineering, RNS Institute of Technology. Page 30 of 34 Renewable Energy Power Plants (21ME652) Module 3 2.11 Gasification process The figure shows schematic diagram of a gasifier with four zones i.e., drying, pyrolysis, oxidation and reduction. The solid biomass under goes partial combustion in a vertical flow packed bed reactor and results in formation of producer gas. There are three zones namely oxidation, reduction and distillation zones in which reactions occurs. In the oxidation zone, O, reacts with carbon of fuel and releases H, and carbon dioxide. In reduction zone, CO, of oxidation zone is reduced to CO. CO + H20 > CO2 + H In the distillation zone, the condensable and non-condensable gases are formed by preheating and carbonizing the raw fuel. The main reactions involved are, C+ 02 + 3.79 N2 ----› 3.79 N2 + CO2 C+ CO2 + 3.79 N2----------- › 3.79 N2 + 2 CO Department of Mechanical Engineering, RNS Institute of Technology. Page 31 of 34 Renewable Energy Power Plants (21ME652) Module 3 2C + O2 + 3.79 N2 -------› 3.79 N2 +2 CO C+ H2O ----- > CO + H2 C+2H2O ------ › CO2 + 2 H2 CO + H2O ------ › CO2 + H2 Biogas is a renewable energy source produced from the anaerobic digestion of organic materials such as agricultural waste, manure, municipal waste, plant material, sewage, green waste, or food waste. Its applications are diverse, ranging from electricity generation to transportation fuels. Below are detailed applications, including its use in engines and cogeneration plants, along with advantages and disadvantages. 2.12 Applications of Biogas 1. Electricity Generation: a. Biogas Power Plants: Biogas can be used to fuel generators to produce electricity. This is particularly common in rural or off-grid areas. b. Cogeneration (Combined Heat and Power - CHP): Biogas can be used in cogeneration plants to simultaneously produce electricity and useful heat, enhancing overall energy efficiency. 2. Heating: a. Residential Heating: Biogas can be used directly in boilers for space heating and water heating. b. Industrial Heating: It can serve as a fuel for industrial processes requiring heat. 3. Transport Fuel: Bio-CNG: Compressed biogas (bio-CNG) can be used as a renewable alternative to compressed natural gas (CNG) in vehicles. 4. Cooking Fuel: Biogas Stoves: In many rural and developing areas, biogas is used for cooking, providing a cleaner alternative to wood or coal. 5. Waste Management: Organic Waste Treatment: Biogas plants help in the management of organic waste, reducing landfill use and mitigating greenhouse gas emissions. 2.13 Application of Biogas in Engines Biogas can be used in internal combustion engines, both spark-ignition (SI) and compression- ignition (CI) engines, after appropriate treatment to remove impurities and adjust the methane content. 1. Spark-Ignition Engines: Department of Mechanical Engineering, RNS Institute of Technology. Page 32 of 34 Renewable Energy Power Plants (21ME652) Module 3 a. Adaptation: Biogas can be used in modified petrol engines, where the air-fuel mixture is ignited by a spark plug. b. Applications:These engines are commonly used in generators for electricity production. 2. Compression-Ignition Engines: Dual-Fuel Systems: Biogas can be used in dual-fuel systems where it is used alongside diesel. Diesel acts as a pilot fuel to initiate combustion. These engines are suitable for heavy-duty applications and power generation. 3. Cogeneration Plant (CHP) A cogeneration plant, or Combined Heat and Power (CHP) plant, utilizes biogas to produce both electricity and thermal energy in a single, integrated system. a. Operation: Electricity Production: Biogas fuels a generator to produce electricity. b. Heat Recovery: The waste heat from electricity generation is captured and used for heating purposes, such as space heating, water heating, or industrial processes. c. Efficiency: Increased Efficiency: CHP systems are highly efficient, with overall efficiencies often exceeding 80%, compared to separate electricity and heat generation. 2.13.1 Advantages of Biogas 1. Renewable and Sustainable: Biogas is produced from renewable resources, reducing dependence on fossil fuels. 2. Waste Management It provides a way to recycle organic waste, reducing landfill use and associated methane emissions. 3. Reduced Greenhouse Gas Emissions: Utilizing biogas helps mitigate methane emissions from waste decomposition. 4. Lower Air Pollution: Biogas combustion produces fewer pollutants compared to fossil fuels. 5. Energy Security: Local production of biogas enhances energy security and reduces import dependence. 6. Economic Benefits: Job creation in the biogas industry and related sectors. 2.13.2 Disadvantages of Biogas Department of Mechanical Engineering, RNS Institute of Technology. Page 33 of 34 Renewable Energy Power Plants (21ME652) Module 3 1. Initial Costs: High initial investment for biogas plant construction and infrastructure. 2. Efficiency Issues: Biogas engines and CHP plants may have lower efficiency compared to conventional natural gas systems. 3. Maintenance: Requires regular maintenance and management to ensure optimal performance. 4. Impurities: Biogas contains impurities like hydrogen sulfide (H S), which can corrodeequipment and must be removed before use. 5. Supply and Storage: Variable biogas production rates due to the dependence on organic waste availability and storage challenges. In summary, biogas is a versatile and environmentally friendly energy source with applications in electricity generation, heating, transport, and more. While it offers significant advantages in terms of sustainability and waste management, challenges such as high initial costs and maintenance requirements must be addressed to fully realize its potential. Department of Mechanical Engineering, RNS Institute of Technology. Page 34 of 34