Renal Physiology: Body Fluids and Kidneys PDF
Document Details
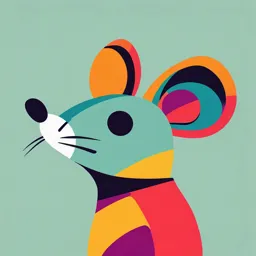
Uploaded by InfluentialJasper4295
University of Exeter
Hiwa S. Namiq
Tags
Summary
This document offers a comprehensive overview of renal physiology, encompassing the intake and output of body fluids and the structure and function of the kidneys. It discusses key concepts like osmosis, edema, and different types of fluid compartments in animal bodies.
Full Transcript
Renal Physiology The Body Fluids and Kidneys/ Dr. Hiwa S. Namiq 20-5-2024 Fluid Intake And Output Daily intake of water. 1. Water ingested as liquids or water in the food, and it is about 2300 ml/day. 2. Water as a result of oxidation of carb...
Renal Physiology The Body Fluids and Kidneys/ Dr. Hiwa S. Namiq 20-5-2024 Fluid Intake And Output Daily intake of water. 1. Water ingested as liquids or water in the food, and it is about 2300 ml/day. 2. Water as a result of oxidation of carbohydrates in side the cells which is about 200 ml/day. Daily loss of water: 1. Insensible water loss. As evaporation from the respiratory tract and diffusion through the skin. It is about 700 ml/day. 2. Fluid loss in sweat. Normally it is about 100 ml/day, but in very hot weather or during heavy exercise can increase to 1 – 2 l/hour. 3. Water loss in feces. It is normally about 100-200 ml/day but highly increased during diarrhea. 4. Water loss by the kidneys. Urine volume can be as low as 0.5 L/day in a dehydrated person or as high as 20 L/day in a person who has been drinking tremendous amounts of water. 2 3 Body fluid compartments The total body fluid is distributed mainly between two compartments: the extracellular fluid and the intracellular fluid. The extracellular fluid is divided into the interstitial fluid and the blood plasma. Transcellular fluid includes fluid in the synovial, peritoneal, pericardial, and intraocular spaces and the cerebrospinal fluid. All the transcellular fluids together constitute about 1 to 2 liters. 4 Constituents Of Extracellular And Intracellular Fluids Major IC and EC fluid cations and Nonelectrolytes of the plasma 5 Basic Principles of Osmosis and Osmotic Pressure Osmosis is the net diffusion of water across a selectively permeable membrane from a region of high water concentration to one that has a lower water concentration. Osmolality and Osmolarity. The osmolal concentration of a solution is called osmolality when the concentration is expressed as osmoles per kilogram of water; it is called osmolarity when it is expressed as osmoles per liter of solution. Osmotic Pressure The precise amount of pressure required to prevent the osmosis is called the osmotic pressure. 6 Effect of Adding Saline Solution to the Extracellular Fluid If isotonic saline is added to the extracellular fluid compartment, the osmolarity of the extracellular fluid does not change; therefore, no osmosis occurs through the cell membranes. The only effect is an increase in extracellular fluid volume If a hypertonic solution is added to the extracellular fluid, the extracellular osmolarity increases and causes osmosis of water out of the cells into the extracellular compartment If a hypotonic solution is added to the extracellular fluid, the osmolarity of the extracellular fluid decreases and some of the extracellular water diffuses into the cells until the intracellular and extracellular compartments have the same osmolarity 7 Total body osmolarity = about 300 mOsm/L 80% of ECF osmolarity is due to NaCl and 50% of ICF is due to K+ Effects of isotonic (A), hypertonic (B), and 8 hypotonic (C) solutions on cell volume Edema: Excess Fluid in the Tissues Edema refers to the presence of excess fluid in the body tissues. It mainly occurs in the extracellular fluid compartment, but it can involve intracellular fluid as well. Intracellular Edema. Causes: 1. Hyponatremia 2. Depression of the metabolic systems of the tissues. 3. Lack of adequate nutrition to the cells. Extracellular Edema. Extracellular fluid edema occurs when there is excess fluid accumulation in the extracellular spaces. (1) Abnormal leakage of fluid from the plasma to the interstitial spaces across the capillaries. (2) Failure of the lymphatics to return fluid from the interstitium back into the blood. 9 Lymphatic blockage causes edema. When lymphatic blockage occurs, edema can become especially severe because plasma proteins that leak into the interstitium have no other way to be removed. Edema caused by heart failure. It is sever because the heart fails to pump blood normally from the veins into the arteries; this raises venous pressure and capillary pressure, causing increased capillary filtration. In the lungs, it causes pulmonary edema. Edema caused by decreased kidney excretion of salt and water. Most of the salt and water added to the blood leaks from the blood into the interstitial spaces, but some remains in the blood. The result will be widespread increase in interstitial fluid volume (extracellular edema) and hypertension. Edema caused by decreased plasma proteins. E.g: Cirrhosis of the liver or nephrotic syndrome. 10 Functions of the Kidneys The kidneys serve the following multiple functions: 1. Excretion of metabolic waste products and foreign chemicals 2. Regulation of water and electrolyte balances 3. Regulation of body fluid osmolality 4. Regulation of arterial pressure 5. Regulation of acid-base balance 6. Secretion, metabolism, and excretion of hormones 7. Gluconeogenesis. 11 Physiologic Anatomy of the Kidneys The two kidneys lie on the posterior wall of the abdomen, outside the peritoneal cavity. Each kidney of the adult human weighs about 150 grams and is about the size of a clenched fist. 12 General organization of the kidneys and the urinary system. 13 The Nephron Each kidney in the human contains about 1 million nephrons, each capable of forming urine. After age 40, the number of functioning nephrons usually decreases about 10 per cent every 10 years. The nephron is the functional unit of the kidney Each nephron contains: 1. A tuft of capillaries called the glomerulus, through which large amounts of fluid are filtered from the blood. 2. A long tubule in which the filtered fluid is converted into urine on its way to the pelvis of the kidney 14 Section of the human kidney showing the major vessels that supply the blood flow to the kidney and a schematic of the microcirculation of each nephron 15 Relations between blood vessels and tubular structures and differences between cortical and juxtamedullary 16 nephrons Urine Formation Urine formation involve three renal processes: 1. Glomerular filtration. 2. Reabsorption of substances from the renal tubules into the blood. 3. Secretion of substances from the blood into the renal tubules Urinary excretion rate = Filtration rate - Reabsorption rate + Secretion rate The GFR is high about 180 L/day and because the plasma volume is only about 3 liters, the entire plasma can be filtered and processed about 60 times each day. Determinants of the GFR: (1) The sum of the hydrostatic and colloid osmotic forces across the glomerular membrane (net filtration pressure) (2) The glomerular capillary filtration coefficient, Kf 17 18 19 20 Substances such as urea, creatinine and uric acid are poorly reabsorbed and are therefore excreted in large amounts in the urine. Foreign substances and drugs are also poorly reabsorbed but, in addition, are secreted from the blood into the tubules, so that their excretion rates are high. The amounts of potassium and hydrogen ions depend mainly on their secretion from the peritubular capillaries. Conversely, electrolytes, such as sodium ions, chloride ions, and bicarbonate ions, are highly reabsorbed, so that only small amounts appear in the urine. Certain nutritional substances, such as amino acids and glucose, are completely reabsorbed from the tubules and do not appear in the urine. 21 Determinants of the GFR: (1) The sum of the hydrostatic and colloid osmotic forces across the glomerular membrane (net filtration pressure) (2) The glomerular capillary filtration coefficient, Kf Average GFR = 125ml/min Determinants of the GFR Increased Glomerular Capillary Filtration Coefficient Increases GFR Increased Bowman’s Capsule Hydrostatic Pressure Decreases GFR Increased Glomerular Capillary Colloid Osmotic Pressure Decreases GFR Increased Glomerular Capillary Hydrostatic Pressure Increases GFR This is dependent on three factors: (1) arterial pressure, (2) afferent arteriolar resistance (3) efferent arteriolar resistance Increases in the filtration fraction (glomerular filtration rate/renal plasma flow) increase the rate at which the plasma colloid osmotic pressure rises along the glomerular capillary; decreases in the filtration fraction have the opposite effect. Renal Blood Flow Blood flow through both kidneys is equal to 1100 ml/min (22% of CO) Regulation of renal blood flow is closely linked to regulation of GFR. Renal artery pressure is about equal to systemic arterial pressure, and renal vein pressure averages about 3 to 4 mm Hg Determinants of Renal Blood Flow Renal blood flow is determined by the pressure gradient across the renal vasculature (the difference between renal artery and renal vein hydrostatic pressures), divided by the total renal vascular resistance: Effect of change in afferent arteriolar resistance or efferent arteriolar resistance on glomerular filtration rate and renal blood flow Tubular Processing of the Glomerular Filtrate Reabsorption and Secretion by the Renal Tubules The urine that is formed and all the substances in the urine represent the sum of three basic renal processes Urinary excretion = Glomerular filtration - Tubular reabsorption+ Tubular secretion Tubular Reabsorption Is Selective and Quantitatively Large The rate at which substances shown in table 1 is filtered is calculated as Filtration = Glomerular filtration rate \ Plasma concentration Table 1: From the table above two facts are apparent : 1-The processes of glomerular filtration and tubular reabsorption are quantitatively very large relative to urinary excretion for many substances 2- Unlike glomerular filtration, which is relatively nonselective, tubular reabsorption is highly selective Passive and Active transport For a substance to be reabsorbed, it must first be transported (1) Across the tubular epithelial membranes into the renal interstitial fluid and then; (2) Through the peritubular capillary membrane back into the blood Water and solutes are transported sequentially by: 1.Transcellular route 2.Paracellular route 3.Ultrafiltration (bulk flow)(hydrostatic and colloid Figure1:Reabsorption of filtered water and solutes from the tubular lumen across the tubular epithelial cells, through the renal interstitium, and back into osmotic forces) into PT capillaries the blood Active Transport Primary active transport- Directly coupled to an energy source as Na-K ATPase Secondary active transport-indirectly coupled to an energy source (to an ion gradient) e.g. as glucose reabsorption Water is always reabsorbed by osmosis (passive mechanism). The known examples of active transporters are: Sodium-potassium ATPase, hydrogen ATPase, hydrogen-potassium ATPase, and calcium ATPase. Active Transport 1.Primary Active Transport Through the Tubular Membrane Is Linked to Hydrolysis of ATP (fig 2) 2.Secondary Active Reabsorption Through the Tubular Membrane (fig 3) 3.Secondary Active Secretion into the Tubules (fig 3) 4.Pinocytosis—An Active Transport Mechanism for Reabsorption of Proteins 5.Transport Maximum for Substances That Are Actively Reabsorbed (fig 4) 6.Substances That Are Actively Transported but Do Not Exhibit a Transport Maximum Luminal vs basolateral membrane Fig. 2:Basic mechanism for active transport of sodium through the tubular epithelial cell Fig. 3:Mechanisms of secondary active transport. The upper cell shows the co-transport of glucose and amino acids along with sodium ions through the apical side of the tubular epithelial cells, followed by facilitated diffusion through the basolateral membranes. The lower cell shows the counter-transport of hydrogen ions from the interior of the cell across the apical membrane and into the tubular lumen (secondary active secretion) Fig. 4:Relations among the filtered load of glucose, the rate of glucose reabsorption by the renal tubules, and the rate of glucose excretion in the urine. The transport maximum is the maximum rate at which glucose can be reabsorbed from the tubules. The threshold for glucose refers to the filtered load of glucose at which glucose first begins to be excreted in the urine. Passive transport 1. Passive Water Reabsorption by Osmosis Is Coupled Mainly to Sodium Reabsorption 2. Reabsorption of Chloride, Urea, and Other Solutes by Passive Diffusion (fig 5) fig 5 :Mechanisms by which water, chloride, and urea reabsorption are coupled with sodium reabsorption Reabsorption and Secretion Along Different Parts of the Nephron Proximal Tubular Reabsorption 65 per cent of the filtered load of sodium and water and a slightly lower percentage of filtered chloride are reabsorbed by the proximal tubule before the filtrate reaches the loops of Henle. Proximal Tubules Have a High Capacity for Active and Passive Reabsorption (fig 6) Concentrations of Solutes Along the Proximal Tubule (fig 7) Secretion of Organic Acids and Bases by the Proximal Tubule Solute and Water Transport in the Loop of Henle(fig 8) The loop of Henle consists of three functionally distinct segments: the thin descending segment, the thin ascending segment, and the thick ascending segment fig 6 :Cellular ultrastructure and primary transport characteristics of the proximal tubule. The proximal tubules reabsorb about 65 per cent of the filtered sodium, chloride, bicarbonate, and potassium and essentially all the filtered glucose and amino acids fig 7 :Changes in concentrations of different substances in tubular fluid along the proximal convoluted tubule relative to the concentrations of these substances in the plasma and in the glomerular filtrate. A value of 1.0 indicates that the concentration of the substance in the tubular fluid is the same as the concentration in the plasma. Values below 1.0 indicate that the substance is reabsorbed more avidly than water, whereas values above 1.0 indicate that the substance is reabsorbed to a lesser extent than water or is secreted into the tubules fig 8-A :Characteristics of thin descending loop fig 8-B: Mechanisms of sodium, of Henle (top) and thick ascending segment of chloride, and potassium transport in the loop of Henle (bottom) the thick ascending loop of Henle Distal Tubule (fig 9) 1. Forms part of JGA 2. Diluting segment 3. Site of action of thiazide diuretics Late Distal Tubule and Cortical Collecting Tubule Principal Cells Reabsorb Sodium and Secrete Potassium (fig 10) The principal cells are the primary sites of action of Potassium-sparing diuretics (fig 11) Intercalated Cells Avidly Secrete Hydrogen and Reabsorb Bicarbonate and Potassium Ions H ion is generated by the action of carbonic anhydrase on water and CO2. Dissociate to H and HCO3. The H ion is actively (H ATPase) secreted into the lumen. Medullary Collecting Duct (Fig 12) Reabsorb only 10% of the filtered water and sodium. Permeability to water is ADH dependent (thus reducing urine vol. and concentrating most of the solutes in the urine) Permeable to urea, raising osmolality in this region of kidney, contributing to kidney’s ability to form a conc. Urine. Capable of secreting H ions, and thus playing a key role in acid-base regulation. fig 9 :Mechanism of sodium chloride transport in the early distal tubule fig 10 :Cellular ultrastructure and transport characteristics of the early distal tubule and the late distal tubule and collecting tubule. Fig 11:Mechanism of sodium chloride reabsorption and potassium secretion in the late distal tubules and cortical collecting tubules. Sodium enters the cell through special channels and is transported out of the cell by the sodium-potassium ATPase pump. Aldosterone antagonists compete with aldosterone for binding sites in the cell and therefore inhibit the effects of aldosterone to stimulate sodium reabsorption and potassium secretion. Sodium channel blockers directly inhibit the entry of sodium into the sodium channels. Fig 12:Cellular ultrastructure and transport characteristics of the medullary collecting duct. The medullary collecting ducts actively reabsorb sodium and secrete hydrogen ions and are permeable to urea, which is reabsorbed in these tubular segments. The reabsorption of water in medullary collecting ducts is controlled by the concentration of antidiuretic hormone. Hormonal Control of Tubular Reabsorption Aldosterone Increases Sodium Reabsorption and Increases Potassium Secretion Angiotensin II Increases Sodium and Water Reabsorption ADH Increases Water Reabsorption Atrial Natriuretic Peptide Decreases Sodium and Water Reabsorption Regulation of Extracellular Fluid Osmolarity and Sodium Concentration Chapter 28/Guyton and Hall text book Dr. Hiwa S. Namiq The Kidneys Excrete Excess Water by Forming a Dilute Urine Low urine osmolality (50 mOsm/L) when too much water is there (reduced osmolality). High osmolar urine (1200 to 1400 mOsm/L) when there is deficit of water (high osmolality) Antidiuretic Hormone (vasopressin) When osmolarity of the body fluids increases above normal (that is, the solutes in the body fluids become too concentrated), ADH (from posterior pituitary) increases the permeability of the distal tubules and collecting ducts to water. The result is reabsorption of large amounts of water with subsequent reduction of urine volume Urinary excretion of solutes does not alter. When there is excess water in the body and extracellular fluid osmolarity is reduced, the secretion of ADH by the posterior pituitary decreases, Thereby the permeability of the distal tubule and collecting ducts to water is reduced and large amounts of dilute urine is excreted Fig 2:Formation of a dilute urine when antidiuretic hormone (ADH) levels are very low B-The Kidneys Conserve Water by Excreting Concentrated Urine Obligatory Urine Volume A normal 70-kilogram human must excrete about 600 milliosmoles of solute each day. If maximal urine concentrating ability is 1200 mOsm/L, the minimal volume of urine that must be excreted, called the obligatory urine volume, can be calculated as Requirements for Excreting a Concentrated Urine—High ADH Levels and Hyperosmotic Renal Medulla (1) a high level of ADH, which increases the permeability of the distal tubules and collecting ducts to water, thereby allowing these tubular segments to avidly reabsorb water, and (2) a high osmolarity of the renal medullary interstitial fluid, which provides the osmotic gradient necessary for water reabsorption to occur in the presence of high levels of ADH. Countercurrent Mechanism Produces a Hyperosmotic Renal Medullary Interstitium Interstitial fluid osmolarity of body is 300 mOsm/L (same osmolality in almost all parts of body) which is similar to osmolarity of plasma. While osmolarity of renal medullary interstitium is much higher, rising progressively to about 1200-1400 mOsm/L in the tip of renal pelvis. Factors contributing to this high medullary osmolarity are 4: 1. Active transport of Na and Co-transport of K and Cl out of thick portion of ALLH. 2. Active transport of ions from the collecting ducts into the medullary interstitium 3. Facilitated diffusion of large amounts of urea from medullary collecting ducts into the medullary interstitium 4. Diffusion of only small amounts of water from the medullary tubules into the medullary interstitium Countercurrent multiplier system in the loop of Henle for producing a hyperosmotic renal medulla (Steps Involved in Causing Hyperosmotic Renal Medullary Interstitium) Urea Contributes to Hyperosmotic Renal Medullary Interstitium and to a Concentrated Urine Urea contributes about 40 to 50 per cent of the osmolarity (500-600 mOsm/L) of the renal medullary interstitium when the kidney is forming a maximally concentrated urine little urea is reabsorbed at distal and cortical collecting tubules (impermeable to urea) high concentrations of ADH, water is reabsorbed rapidly from the cortical collecting tubule and the urea concentration increases rapidly tubular fluid flows into the inner medullary collecting ducts, still more water reabsorption takes place, causing an even higher concentration of urea in the fluid urea diffuse out of the tubule (inner medullary collecting duct) into the renal interstitium (greatly facilitated by specific urea transporters). Role of ADH (activation of urea transporters) Fig 4:Recirculation of urea absorbed from the medullary collecting duct into the interstitial fluid. This urea diffuses into the thin loop of Henle, and then passes through the distal tubules, and finally passes back into the collecting duct Countercurrent Exchange in the Vasa Recta Preserves Hyperosmolarity of the Renal Medulla A sufficient blood supply to the renal medullary cells should be maintained to prevent solute dissipation provided by CCM system. There are two special features of the renal medullary blood flow that preserve the high solute concentrations: 1. The medullary blood flow is low (less than 5% of total renal blood flow) This sluggish blood flow is sufficient to supply the metabolic needs of the tissues but helps to minimize solute loss from the medullary interstitium. 2. The vasa recta serve as countercurrent exchangers, minimizing washout of solutes from the medullary interstitium. The countercurrent exchange mechanism Blood enters and leaves the medulla by way of the vasa recta at the boundary of the cortex and renal medulla. The vasa recta are highly permeable to solutes in the blood, except for the plasma proteins. As blood descends into the medulla toward the papillae, it becomes progressively more concentrated, partly by solute entry from the interstitium and partly by loss of water into the interstitium. By the time the blood reaches the tips of the vasa recta, it has a concentration of about 1200 mOsm/L, the same as that of the medullary interstitium. As blood ascends back toward the cortex, it becomes progressively less concentrated as solutes diffuse back out into the Tubules medullary interstitium and as water moves into the vasa recta (fig 5). Fig 5: Countercurrent exchange in the vasa recta. Plasma flowing down the descending limb of the vasa recta becomes more hyperosmotic because of diffusion of water out of the blood and diffusion of solutes from the renal interstitial fluid into the blood. In the ascending limb of the vasa recta, solutes diffuse back into the interstitial fluid and water diffuses back into the vasa recta. Large amounts of solutes would be lost from the renal medulla without the U shape of the vasa recta capillaries.