Rad Bio Module 2: Molecular and Cellular Radiation Biology PDF
Document Details
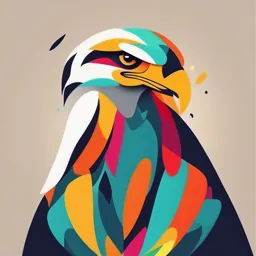
Uploaded by UnboundCarnelian9422
Tags
Summary
This document provides an overview of molecular and cellular radiation biology, specifically focusing on fundamental principles. It includes details on ionizing radiation, the Law of Bergonie and Tribondeau, as well as cellular radiosensitivity. Key concepts like tissue weighting factors and linear energy transfer are also introduced.
Full Transcript
Module 2: Molecular and Cellular Radiation Biology Lesson 1: Fundamental Principles of RBP X-ray energy is absorbed by tissue (dose); with damage primarily occurring at the cellular level Ionizing Radiation Two main types of ionizing interactions that occur in diagnostic imaging are: ○...
Module 2: Molecular and Cellular Radiation Biology Lesson 1: Fundamental Principles of RBP X-ray energy is absorbed by tissue (dose); with damage primarily occurring at the cellular level Ionizing Radiation Two main types of ionizing interactions that occur in diagnostic imaging are: ○ Photoelectric effect ○ Compton scatter production Both interactions involve removal of an electron from an atom → ionization Biological damage begins with one ionization event Law of Bergonie and Tribondeau Radiosensitivity = function of the metabolic state of the cell receiving the exposure. ⇩ Radiosensitivity of a cell is directly proportional to their reproductive activity; and inversely proportional to their degree of differentiation ⇩ Mature fully developed cell is LESS radiosensitive than an immature cell that is actively reproducing. ○ Basis of why children & fetuses are more radiosensitive than adults Bushong 12th ed. Box 30-1 Law of Bergonie and Tribondeau - Stem cells are radiosensitive/ mature cells are radioresistant - Younger tissues and organs are radiosensitive - Tissues with high metabolic activity are radiosensitive. - High proliferation rate for cells & a high growth rate for tissues result in increased radiosensitivity. Classification of cellular radiosensitivity → do NOT need to memorize this (consider Law of B & T) * NOTE: lymphocytes, (although classified as relatively radioresistant by its characteristics) are in fact very radiosensitive. Characteristics Examples Radiosensitivity Rapidly dividing; undifferentiated Erythroblasts, type A spermatogonia, crypt cells Most radiosensitive of intestines, basal cells of epidermis Actively dividing; somewhat differentiated Intermediate spermatogonia, Myelocytes Relatively radiosensitive Irregularly dividing; more differentiated Endothelial cells Fibroblasts Intermediate in radiosensitivity Do not normally divide but retain Parenchymal cells of the liver and adrenal glands Relatively radioresistant capability of division; differentiated Lymphocytes* Bone, muscle cells Do not divide; differentiated Some nerve cells; Erythrocytes; Spermatozoa Most radioresistant Tissue Weighting Factors Sherer 9th ed. Table 4-3 New ICRP Recom. Organ/tissue Weighting Factor (Wt ) Weighting Factor (Wt ) Gonads 0.20 0.08 Red bone marrow, colon, lung, stomach 0.12 0.12 Bladder, liver, esophagus, thyroid 0.05 0.04 Skin, bone surface 0.01 0.01 Breast 0.05 0.12 Remainder*† 0.05 0.12 Interaction of Radiation with Tissue Energy is deposited rapidly and randomly (in less than 10 -10 sec) by ionizing radiation via excitation, ionization, and thermal heating Radiation-induced injury to an organism always begins with chemical changes at the atomic and molecular level Changes produced in molecules, cells, tissues, and organs are not unique Biologic changes resulting from radiation induced damage are apparent only after a period of time (latent period) Only a fraction of the radiation energy deposited causes chemical changes; the majority of the energy is deposited as heat Radiation Energy Transfer Determinants Linear Energy Transfer Relative Biologic Effectiveness Oxygen Enhancement Ratio Linear Energy Transfer (LET) It is an important factor in assessing potential tissue damage from exposure to ionizing radiation. It is a way of expressing radiation quality and determining radiation weighting factors. As ionizing radiation passes through a medium, it may interact with it along its track. This may cause the photon to lose energy. Average amount of energy transferred per unit length of track is called LET LET is described in units of kiloelectron volts per micrometer of track length ○ 1 keV per 1 µm; where 1 µm = 10 -6 m The rate of energy transfer in diagnostic imaging is about 3 keV/ µm ○ this means the LET of diagnostic x-rays is 3 As LET increases, the chance of damaging DNA also increases LET is categorized as either: ○ Low → travels far with few interactions → (x-rays) ○ High → travels short distance with many interactions → (alpha particles) High vs Low LET A → An electron produced from Compton scatter travels further with few interactions B → The alpha particle travels a shorter distance but has many more interactions Low-LET Radiation High-LET Radiation - Gamma rays - Alpha particles - X-rays - Ions of heavy nuclei - Charged particles released from interactions btw neutrons and atoms - Low-energy neutrons Low LET Radiation X-rays, Gamma rays Neither of these has mass or charge Penetrating EMR is sparsely ionizing and interacts randomly along its track It causes damage primarily due to an indirect action that involves the production of free radicals Also, but less likely, radiation may directly induce single-strand breaks in the ladder-like DNA structure Generally cause sublethal damage to DNA, repair enzymes can usually reverse the cellular damage High LET Radiation Particles that possess mass and charge: ○ Alpha particles ○ Low-energy neutrons ○ Charged particles released from interactions between neutrons and atoms. Dense ionization along its track means more likelihood of biologic damage. Lose energy more rapidly than low-LET radiations because they produce many more ionizations per unit of distance traveled Deposit more energy per unit of biologic tissue traversed, therefore they are more destructive to biologic matter Relative Biologic Effectiveness (RBE) RBE describes the relative capabilities of radiation with differing LET’s to produce a particular biologic reaction. ○ All ionizing radiation is capable of producing the same biologic effects; however the magnitude of the effect per unit dose differs among them ○ Equal doses To evaluate effectiveness of different types of radiation; experiments are performed comparing the test radiation against a reference dose (e.g. x-rays produced from 250 kVp) Diagnostic x-rays have an RBE of 1. Radiation with a higher LET will have an RBE greater than 1. RBE is also dependent on the total dose and dose rate Radiation Weighting Factor (WR) The RBE is used to calculate the Radiation Weighting Factor (WR ) ⇩ This is used to calculate the equivalent dose (EqD) ⇩ EqD is used to determine the ability of dose of any kind of ionizing radiation to cause biologic damage. ⇩ The WR for X-radiation is 1 Radiation Energy Transfer Determinants → LET and RBE of Various Radiation Doses Oxygen Enhancement Ratio (OER) Biologic tissue is more sensitive to radiation in an oxygenated or aerobic state than under anoxic or hypoxic conditions. This is known as the oxygen effect. Presence of free oxygen increases the damage caused by low-LET radiation OER is the ratio of the radiation dose required to cause a particular biologic response in cells in an oxygen-deprived environment to the dose required to cause identical response under normal oxygenated conditions. OER describes the effect numerically Oxygen enhancement ratio (OER) is high for low linear energy transfer (LET) radiation and decreases in value as the LET increases. An outcome of an indirect action is the formation of a free radical which dramatically increases the amount of biologic damage Free radicals may combine with oxygen to form organic peroxide compounds therefore rendering the biologic damage permanent. This phenomenon has been called the oxygen fixation hypothesis. Lesson 2: Molecular Radiation Biology → Radiolysis of water Introduction Macromolecules are synthesized from smaller molecules (anabolism). They contain many atoms. Proper synthesis of proteins and nucleic acids is critical to the survival of the cell and to its reproduction. Radiation damage to any macromolecules may result in cell death or late stochastic effects Molecular damage results in the formation of structurally changed molecules that may impair cellular functioning. ○ Damage to somatic cells may result in a disruption of body processes ○ Damage to germ cells may be passed onto future generations in the form of genetic mutations Radiation causes ionizations of: ATOMS which may affect ⇩ MOLECULES which may affect ⇩ TISSUES Which may affect ⇩ ORGANS which may affect ⇩ THE WHOLE BODY Classification of Ionizing Radiation Interaction Ionizations and excitations are produced either in biologic macromolecules or in water. Based on these sites, the effect of radiation on the cell is classified as either: Direct → Radiation interacts with macromolecules Indirect → Damage occurs due to ionization of water molecules Direct Action Biologic damage occurs as a result of ionization of atoms on essential macromolecules. ○ These macromolecules may become inactive or functionally altered. More likely to occur with high LET radiation This radiation may damage macromolecules such as DNA, RNA, proteins and enzymes through photoelectric and Compton interactions. Human body contains approximately 80% water molecules. Therefore, most radiation interactions in the body are with water molecules. If an x-ray photon ionizes a water molecule the result could be a water molecule with a positive charge and a free electron. → HOH+ and e- The electron could recombine with the positively charged water molecule creating a stable water molecule. Or, the free electron could combine with a stable water molecule resulting in a negative water ion. Positive and negative water molecules are unstable. → HOH - HOH+ and the HOH-are unstable The positive and negative water molecules may break apart into smaller molecules. Formation of ion pairs Hydrogen ion (H + ) and hydroxyl radical (OH*) Hydroxyl ion (OH - ) and hydrogen radical (H*) If the ions recombine into a water molecule, then no biologic damage will occur. Free radicals: Approximately two thirds of all radiation-induced damage is believed to be ultimately caused by the hydroxyl free radical (OH*) May cause biologic damage by transferring their excess energy to other molecules Can produce damage in areas remote from initial interaction Free Radicals Direct Action – radiolysis of water Production of Cell-Damaging Substances ○ Free radicals can combine with each other to form other molecules such as hydrogen peroxide OH* + OH* → H2O2 is highly toxic to the cell Oxygen combines with hydrogen free radical to form hydroperoxyl radical H* + O2 → HO2* These 2 are believed to be most damaging The final result of the interaction of radiation with water is the formation of an ion pair (H + and OH – ) and two free radicals (H* and OH*). Indirect Action Involves the breakdown of water molecules into ions and free radicals. When free radicals act on a molecule such as DNA, the damaging action of ionizing radiation is indirect because it is not the radiation itself that is causing the damage. The byproducts of the ionization are causing the damage Free radicals, produced during radiolysis, may cause damage to the macromolecule; this is called indirect action ○ The possibility of indirect action is great because the human body is 80% water and less than 1% DNA Lesson 3: Effects of Radiation on DNA and Chromosomes Target Theory DNA is considered to be the target molecule. If the target molecule becomes inactivated, then the cell will die. If ionizing radiation damages DNA, then that is considered a hit. More than 1 hit on target molecule is required to severely damage a cell. Hits on the target molecule can be through direct or indirect effects. This is the basis for Target Theory Target theory is the concept used to explain cell death and non- fatal cell abnormalities caused by exposure to ionizing radiation. The interactions between radiation and cellular components is random. ○ Hits occur through both direct and indirect effects An indirect hit on the target is more likely due to the mobility of free radicals. With low LET radiations (with absence of oxygen) the probability of a hit is low due to large distances between events. If oxygen is present with low LET radiations, free radicals are formed and the likelihood of a hit increases. With high LET radiations, the probability of a direct hit on the target increases. Effects of Ionizing Radiation on DNA Single-strand break Double-strand break Double –strand break in the same rung of DNA Mutation Covalent cross-links Single-Strand Break The energy from ionizing radiation is transferred to the DNA molecule resulting in the disruption of the phosphate bond on one side rail or strand Called a point lesion; commonly occur with low LET radiation Repair enzymes are capable of reversing this damage Double-Strand Break The energy from ionizing radiation is transferred to the DNA molecule resulting in the disruption of the phosphate bond on both side rails or strands Double-strand breaks occur more commonly with high LET radiations Damage may lead to a mutation; change or loss of nitrogenous base Slighter chance of repair when compared with a single-strand break, however chance of reversing this damage is low Double-strand break in the Same Rung of DNA Results in a cleaved or broken chromosome Each new portion containing an unequal amount of genetic material During cell division each daughter cell receives an incorrect amount of genetic material Results in impaired function or death of the daughter cell. Mutation Generally a result of interaction with high energy radiation The consequence is an alteration of the nitrogenous base sequence The damage may not be reversible causing acute consequences to the cell and passing on of incorrect genetic information to the daughter cells Covalent Cross-Links Chemical unions created between two atoms by the single sharing of one or more pairs of electrons After irradiation molecules can produce small spur-like extensions that become very “sticky”; resulting in the attachment to other macromolecules (interstrand) or other segments of the same macromolecule chain (intrastrand) Covalent cross-links are caused by direct action initiated by high-energy radiation or indirect action at low energies. Potentially fatal if not repaired Effects of Ionizing Radiation on chromosomes When changes occur in the DNA molecule it is the chromosome that exhibits the variation A hit on a chromosome can be observed, and it is assumed that a chromosome hit results in severe damage to DNA. Some of the topics covered in these slides include: ○ Radiation-induced Chromosome Breaks May be viewed microscopically during metaphase and anaphase Radiation-induced chromosome breaks can occur in somatic and reproductive cells ○ Chromosomal Fragments Produced after chromosome breakage Broken ends are very active and have a strong tendency to adhere to another sticky end. Fragments may: Rejoin in original configuration Fail to rejoin and create an aberration (lesion or anomaly) Join to other broken ends and create new chromosomes ○ Chromosome Anomalies → Chromosome aberration Chromosome damage that occurs before DNA synthesis = chromosome aberration Occurs in early interphase Break is in a single strand of chromatin Damaged chromatin does not have a chance to repair The break is replicated in DNA synthesis and each daughter cell will inherit the damaged chromatid ○ Chromosome Anomalies → Chromatid aberration Chromosome damage that occurs after DNA synthesis = chromatid aberration Occur in late interphase (after DNA synthesis) Only one chromatid of a pair may undergo a break Only one daughter cell is affected Cytogenetic Effects A photomicrograph is taken during metaphase when individual chromosomes are demonstrated Almost every type of chromosome aberration may be caused by ionizing radiation; some aberrations may be specific to radiation Chromosome imperfections have been observed after high-dose fluoroscopy procedures Single-Hit Chromosome Aberrations Structural radiation damage to individual chromosomes can be visualized during mitosis phase. In G1 phase chromosome aberrations include deletion and acentric fragment or isochromatids. Occur with very low doses of radiation Bushong 12th ed. Fig 33-11 → You do not need to memorize the cell phases A chromosome hit represents severe damage to the DNA. A single chromosome can sustain more than one hit. In G1 phase chromosome aberrations include ring formation and dicentric More likely to happen at doses above 1 Gyt (100 rad) Multi-hit aberrations are not uncommon. Reciprocal translocation is a chromosome aberration representing severe damage to a cell. Acentric fragments may be lost completely or rearrangement of the genetic material Multi-hit aberrations are considered to be the most significant in terms of latent human damage Consequences to the Cell from Structural Changes in the Nucleus 1. Restitution ○ Breaks rejoin in original configuration ○ No visible damage ○ 95% of single chromosome breaks believed to mend by restitution 2. Deletion ○ Part of the chromosome or chromatid is lost in next cell division ○ Aberration created called an acentric fragment ○ Results in a cell mutation 3. Broken-end rearrangement ○ A grossly misshapen chromatids may be produced ○ Ring chromatids, dicentric chromosomes, and anaphase bridges ○ Results in a cell mutation 4. Broken-end rearrangement without visible damage to the chromatids ○ Chromatid genetic material has been rearranged, though the chromatid appears normal ○ Translocations ○ Results in a cell mutation Summary of Structural Changes Caused by Ionizing Radiation Single-strand break in one chromosome Single –strand break in one chromatid Single-strand break in separate chromosomes A strand break in separate chromatids More than one break in the same chromosome More than one break in the same chromatid Chromosome stickiness or clumping together RECALL: ○ Most chromosomal damage results from indirect action of ionizing radiation ○ Free radicals will interact with vital macromolecules and this can cause chromosome breakage. ○ Only a very small percentage of chromosome breakage is due to the direct action of ionizing radiation. Chromosomal damage that results from direct or indirect action is called a hit. ○ This will produce a visible de-arrangement of the chromosome. The total radiation dose received and the period of time in which the dose is delivered determine the rate of production of chromosome aberrations. Cell Recovery Interphase death occurs when the cell dies before replicating If the cell hasn’t died it can recover from sublethal radiation damage. Repair enzymes can aid in mending damage to a cell There can also be a repopulation of healthy surviving cells The combined processes of intracellular repair and repopulation contribute to recovery from radiation damage. Lesson 4: Cellular Radiation Biology Review Cells contain many molecules and most of these are in abundance So if one molecule gets damaged the others will be able to support cell function. However, DNA is unique and not abundant. Damage to DNA can affect cell function or cause cell death Define Acute dose → total dose of radiation administered over such a short period of time that biological recovery is impossible Effects of Irradiation on the Entire Cell: Overview Ionizing radiation transfers energy to the cell’s nucleus. Damage to the cell’s nucleus can result in ○ Instant death ○ Reproductive death ○ Apoptosis ○ Mitotic or genetic death ○ Mitotic delay ○ Interference with function ○ Chromosome breakage Effects of Irradiation on the Entire Cell: Instant Death Instant death occurs when tissue is irradiated with an acute dose of about 1000 Gy Results in gross disruption of cellular components and severe changes in cellular mechanisms DNA macromolecule breaks up and cellular proteins coagulate. These large doses are NOT reached in diagnostic imaging and unlikely reached in therapeutic treatments Effects of Irradiation on the Entire Cell: Reproductive Death Results from irradiation in the dose range of 1-10 Gy Inability of a cell to undergo repeated divisions and therefore the inability of a cell to produce progeny The cell is considered “dead” though it may technically be alive e.g. ability to metabolize Effects of Irradiation on the Entire Cell: Apoptosis Apoptosis or programmed cell death ○ Non-mitotic or non-division cell death; cells die before attempting mitosis, during the interphase portion of the cell cycle ○ Occurs spontaneously in both healthy and diseased tissue, in human beings and in nature Effects of Irradiation on the Entire Cell: Mitotic Death Ionizing radiation has the potential to adversely affect cell division ○ mitotic or genetic death results when the cell divides once or more after irradiation, then dies. Effects of Irradiation on the Entire Cell: Mitotic Delay A dose of 0.01 Gy to a cell just before it enters division can cause the failure of the cell to start dividing on time. This is referred to as mitotic delay. Following mitotic delay, the cell may resume its normal mitotic function Possible causes of mitotic delay include; ○ alteration of chemical involved in mitosis, ○ required protein for mitosis not being synthesized, ○ change of rate of DNA synthesis after irradiation Effects of Irradiation on the Entire Cell: Interference of Function Exposure to ionizing radiation may result in a permanent or temporary interference of cellular function The potential exists for enzymes to repair the damage allowing cell to recover and continue to function Cellular Effects of Irradiation – Chromosome Breakage Potential outcome of the direct effect of ionizing radiation with a DNA molecule resulting in breaks in one or both DNA sugar-phosphate chains Irradiation during mitosis resulting in chromosome breakage will produce permanent chromosome abnormalities which may lead to genetic mutations in subsequent generations Lesson 5: Cell Types and Their Radiosensitivity Recall Undifferentiated cells undergo rapid cell division and are more radiosensitive. Highly differentiated cells divide at a slower rate and are therefore less radiosensitive. Radiosensitivity varies with different tissues and organs. Other factors that influence a cells radiosensitivity are: ○ LET ○ Oxygen enhancement ○ Dose rate Remember the oxygen effect The tissue is more sensitive to radiation if there is oxygen present. If oxygen is present, then more free radicals will be produced. This increases the possibility of indirect damage to tissues. OER = radiation dose required to cause biological response without oxygen. radiation dose required to cause biologic response with oxygen Dose rate plays a role Dose Rate → define as radiation dose delivered per unit time and measured, for instance, in grays per hour † ○ High dose rates are more effective at producing biologic damage than low dose rates ○ Cells have a greater opportunity to repair sublethal damage at low dose rates than higher dose rates, reducing the amount of damage and increasing the survival fraction Fractionation ○ A radiation dose that is administered as a combination of smaller doses with time between doses. Time allows for repair of sublethal damage to the cell Cell Radiosensitivity changes with stage of cell cycle Stage of Cell Cycle In general, cells are most sensitive to radiation during mitosis (M phase) and RNA synthesis (G2), less sensitive during the preparatory period for DNA synthesis (G1), and least sensitive during DNA synthesis (S phase). Low, moderate, high doses In diagnostic imaging, X-rays have low LET, however, some procedures produce more dose than others. General duty radiography generally imparts the lowest dose. ○ with smaller kV and mAs being low dose (extremities), ○ while higher kV and mAs would be a higher dose (chest, abdomen). CT examinations give more dose to patients than general duty. Patients who undergo long fluoroscopic procedures may be receiving a higher dose because of the length of the exam. ○ Example would be cardiac catheterization, where it may take several minutes of imaging time to complete this exam ○ This type of exam may cause early tissue damage Cell characteristics and Dose Recall, that cells have varying degrees of radiosensitivity. Undifferentiated rapidly dividing cells need less radiation to sustain damage. So, both the cell type and amount of radiation need to be considered to determine the Effective Dose of a particular imaging procedure. Local Tissue Damage vs Whole-Body Dose Local tissue damage considers a localized area of the body. Such as the head or abdomen. A whole-body dose would be more likely to occur in other situations such as in the vicinity of a nuclear power plant explosion. The following slides will discuss the response of the body’s important cell groups to ionizing radiation(IR). Dose amounts stated in the following slides are for your information only and to help you have an idea of the amount of dose required for various effects to appear. You do not need to memorize the dose numbers. Blood Cells Hematologic Depression ○ IR will cause a decrease in the number of cells in the peripheral circulation. Depletion of Immature Blood Cells ○ Radiation can cause a decrease in the number of immature blood cells produced in bone marrow. ○ This will lead to an overall reduction in the number of mature blood cells in the blood stream. ○ Increase dose to bone marrow results in less blood cells Repopulation after a Period of Recovery ○ Bone marrow cells may repopulate after a period of time. ○ The time required depends on the magnitude of the dose received. Low dose < 1 Gyt → repopulation can occur within weeks of irradiation Moderate dose (1-10 Gyt ) to high dose (> 10 Gyt ) requires more time Very high doses may cause a permanent depletion of stem cells Effects on Stem Cells of the Hematopoietic System ○ All stem (precursor) blood cells are more radiosensitive than the circulating mature cells ○ After a high dose of whole body irradiation death typically occurs due to the body’s incapability to combat infection due to destruction of the myeloblasts and by internal hemorrhage due to destruction of megakaryoblasts Whole-Body Doses in Excess of 5 Gyt (500 rad) ○ Death due to a whole-body exposure of this magnitude usually results in death within 30 to 60 days (LD 50/30 or LD 50/60); death is due to depletion of the stem cells of the hematopoietic system ○ Whole body lethal dose in humans is estimated to be 3.0 to 4.0 Gyt (300 to 400 rad) White Blood Cells Effects of IR on Lymphocytes ○ White blood cells = leukocytes ○ Lymphocytes are a type of white blood cell ○ Dose of 0.25 Gyt or less, complete recovery occurs ○ Higher whole-body doses of 0.5-1 Gyt lymphocyte count decreases to 0. Several months will be required for full recovery Effects of IR on Neutrophils ○ Neutrophils also help to fight infection ○ Dose of 0.5 Gyt IR reduces number of neutrophils in circulating blood. ○ Dose of 2 – 5 Gyt causes depletion to 10% or less. This would require several months for recovery. Effects of IR on Granulocytes ○ Granulocytes fight bacteria ○ After IR they temporarily increase in number ○ Then they rapidly decline in number. ○ They fully repopulate in about 2 months. (depending on dose) Effects of IR on Thrombocytes. ○ Initiate blood clotting and prevent hemorrhage. ○ Live for about 30 days ○ A dose greater than 0.5 Gyt will deplete number in circulating blood ○ A dose 1-10 Gyt causes significant depletion that will require about 2 months for recovery Blood Cells and Diagnostic Imaging Neither the blood or bone marrow should experience appreciable damage due to being exposed to IR in the diagnostic range. ○ Chromosomal damage in circulating lymphocytes is possible ○ This has been documented in patients who underwent fluoroscopic studies that resulted in a long exposure time. Example: Cardiac catheterization Epithelial Tissue Found lining the intestines, the mucous lining of the respiratory tract, pulmonary alveoli and lining of blood and lymphatic vessel. These cells regenerate continuously through mitosis. Therefore, it is very radiosensitive. Muscle Tissue Muscle cells are highly specialized and do not divide Therefore, are relatively insensitive to radiation. Nervous Tissue Found in brain and spinal cord ○ Nerve cells, (neurons) relay messages to and from the brain ○ In the Adult, nerve cells are highly specialized. do not divide → if the nucleus of the nerve cell is destroyed, the cell dies and is not restored. If the nucleus is damaged, the function of the damaged nerve cell will be impaired Require extremely high doses of radiation to cause damage Developing nerve cells are more radiosensitive than mature cells; especially the 8th to 15th weeks of gestation ○ Irradiation during this time may result in microcephaly and mental retardation A 0.1-Sv (10 -rem) fetal EqD is associated with a 4% risk of mental retardation. This level is considered significant compared with risks during a normal pregnancy. Therefore it is important to limit irradiation of the pelvis or abdomen of a pregnant patient and ensuring the risk associated with the exposure is justified Reproductive Cells Spermatogonia ○ The male testes contain both mature and immature spermatogonia The immature spermatogonia are unspecialized and rapidly dividing and therefore extremely radiosensitive ○ A dose of 0.1 Gyt (10 rad) may depress the number of germ cells and may cause genetic mutations in future offspring ○ A dose of 2 Gy (200 rad) may cause temporary sterility; while a 5 or 6 Gy dose may cause permanent sterility ○ In diagnostic imaging, it is highly unlikely that the germ cells of patients would receive a dose of 0.1Gyt and radiographers working under normal conditions would never receive a gonad dose of this level. Ova ○ Between ages of approx. 12 to 50 years, one mature ovum is expelled from ovary every 28 to 36 days ○ Radiosensitivity varies throughout the lifetime of the germ cell Immature ova are very radiosensitive. Damage occurring from exposure to radiation may result in damaged chromosomes and a mutation being passed onto future generations Exposure to ionizing radiation may also cause sterility ○ ovaries of fetus and young child are very radiosensitive They contain large number of oogonia and oocytes ○ least sensitivity between 20 and 30 years Most cells are mature ○ after age 30 and until menopause overall sensitivity increases ○ after menopause sensitivity increases due to the destroyed ova not being replenished a single radiation dose of 2 Gyt will cause temporary sterility. Single dose of 5 Gyt will cause permanent sterility Dose of 0.1 Gyt (10 rad) may cause menstrual irregularities and may cause genetic mutations in future offspring