Radiobiology 2nd Lecture PDF
Document Details
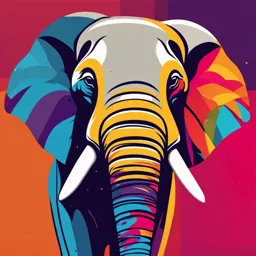
Uploaded by ImpressedBigfoot
Tehran University of Medical Sciences
Tags
Summary
This document discusses various aspects of radiobiology, including the effects of ionizing radiation on cells, different types of radiation interactions, and the concept of Relative Biological Effectiveness (RBE). This document also covers the topic of cellular injury and different dose-response models.
Full Transcript
More DNA is present in one area at this point in the cycle, which is why it is theorized that this is the most radiosensitive time. It is also thought that increased chromatin in cancer cells is why these cells, which have unusually high mitotic rates, are more radiosensitive than norm...
More DNA is present in one area at this point in the cycle, which is why it is theorized that this is the most radiosensitive time. It is also thought that increased chromatin in cancer cells is why these cells, which have unusually high mitotic rates, are more radiosensitive than normal cells. Indirect Interaction The other type of interaction is indirect cellular interaction. Indirect interaction occurs when radiation energy is deposited in the cell, and the radiation interacts with cellular water rather than with macromolecules within the cell. The reaction that occurs is hydrolysis of the water molecule, resulting in a hydrogen molecule and hydroxyl (free radical) molecule. If the 2 hydroxyl molecules recombine, they form hydrogen peroxide, which is highly unstable in the cell. This will form a peroxide hydroxyl, which readily combines with some organic compound, which then combines in the cell to form an organic hydrogen peroxide molecule, which is stable. This may result in the loss of an essential enzyme in the cell, which could lead to cell death or a future mutation of the cell (Table 1). Antioxidants, about which there has been much research and publicity, block hydroxyl (free radical) recombination into hydrogen peroxide, preventing stable organic hydrogen peroxide compounds from occurring. This is one way in which the body can defend itself from indirect radiation interactions on a cellular level, and is one reason that antioxidants have received so much attention recently as a cancer prevention agent. CELLULAR INJURY There are 3 ways for cellular injury to occur after ionizing radiation exposure. They are: 1) division delay, with dose dependent delay in cell division; 2) reproductive failure, when cells fail to complete mitosis either immediately or after one or more generations; 3) interphase death, a relatively prompt death caused by the apoptosis mechanism. The last is seen most commonly with lymphocytes, although some cancer cells show apoptosis in response to radiation In division delay, mitotic division is delayed but later returns to near normal for unknown reasons. This is seen in doses greater than 0.5Gy (50 rads) up to approximately 3 Gy (300 rads). This is the first observable effect from ionizing radiation exposure. At more than 3 Gy (300 rads), the mitotic rate does not recover and the division may never happen, thus killing the cell. Reproductive failure of a cell is based on the dose. At levels at or below 1.5 Gy (150 rads), reproductive failure is random and nonlinear. At doses above 1.5 Gy (150 rads), it is linear and nonrandom. As dose increases, so does reproductive death. In interphase death, cell death can occur many generations from the initial radiation exposure. It is thought that this is either a natural process of aging cells (apoptosis), or that a critical mechanism of cell replication has been altered. It depends on the type of cell affected and the dose to the cell. Generally, rapidly dividing, undifferentiated cells exhibit interphase death at lower doses than non dividing, differentiated cells. Any of these types of cellular injury can happen as a result of either direct or indirect cellular interactions with radiation. RBE Energy loss effects depends on nature and probability of interaction between radiation particle and body material. The amount or quantity of radiation is expressed in terms of the absorbed dose, a physical quantity with the unit of Gray or Rad. Dose is a measure of energy absorbed per unit mass of tissue Equal doses of different types of radiation do not produce equal biologic effects. One gray of neutrons produces a greater biologic effect than 1 Gy of X-rays. The key to the difference lies in the pattern of energy deposition. Deposition of energy of different particles Photon The electrons set in motion if X-rays are absorbed are very light, negatively charged particles. X-rays are sparsely ionizing Neutron By contrast, the particles set in motion if neutrons are absorbed are heavy and densely ionizing. As the density of ionization increases, the probability of a direct interaction between the particle track and the target molecule (possibly DNA) increases Alpha particle RBE In comparing different radiations it is customary to use x-rays as the standard. To normalize these effects as an empirical parameter the Relative Biological Effectiveness RBE of radiation for producing a given biological effect is introduced: dose in Gy from 250 keV X-rays / dose in Gy from another RBE = radiation source to produce the same biologic response The RBE for different kinds of radiation can be expressed in terms of energy loss effects LET. Linear Energy Transfer (LET) is the rate at which energy is deposited as a charged particle travels through matter by a particular type of radiation Linear Energy Transfer (LET): the energy deposited per unit track. It is determined by: Unit is keV/m. quality of radiation quantity of radiation The different kinds of radiation have received dose of radiation different energy loss effects LET. exposure conditions (spatial distribution) The linear energy transfer (LET)of charged particles in the medium is the quotient of dE/dx, where dE is the average energy locally imparted to the medium by a charged particle of specified energy in traversing a distance of dx. On the following diagram, each dot represents a unit of energy deposited. As you will see from the diagram, alpha particles impart a large amount of energy in a short distance. Beta particles impart less energy than alpha, but are more penetrating. Gamma rays impart energy sparsely and are the most penetrating. Remember, gamma and x-rays vary widely in energy. The diagram shows a high energy gamma ray. dispersion of energy air tissue incident radiation high LET (, n, ~) greater radiotoxicity low LET (, x, ~) LET = linear energy transfer Typical Linear Energy Transfer Values The optimal LET LET of about 100 keV/μm is optimal in terms of producing a biologic effect. At this density of ionization, the average separation in ionizing events is equal to the diameter of DNA double helix which causes significant DSBs. DSBs are the basis of most biologic effects. The probability of causing DSBs is low in sparsely ionizing radiation such as x-rays that has a low RBE. For low LET radiation, RBE LET, for higher LET the RBE increases to a maximum, the subsequent drop is caused by the overkill effect. These high energies are sufficient to kill more cells than actually available! In the case of sparsely ionizing X-rays the probability of a single track causing a DSB is low, thus X-rays have a low RBE. At the other extreme, densely ionizingradiations (ex. LET of 200 keV/ μm) readily produce DSB, but energy is “wasted” because the ionizing events are too close together. Thus, RBE is lower than optimal LET radiation. DOSE–RESPONSE MODELS There are many different theoretical types of dose– response models used to explain the effects of radiation exposure. Different models suggest different possibilities of response to radiation exposure. These range from linear–no threshold, which suggests any exposure (even background radiation) is harmful, to the possibility that low-dose radiation exposure is beneficial (radiation hormesis). Three dose– response models used in radiation biology are: 1) linear–no threshold, 2) linear–threshold, 3) linear quadratic, These dose–response models are used to extrapolate high-dose effects (which are known) to the low-dose range (which has not been reliably detected)