Greisens and Related Ore Deposits PDF
Document Details
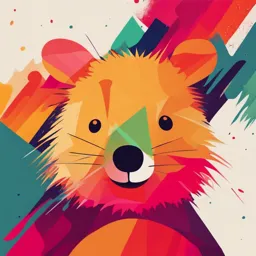
Uploaded by FriendlyTrust
University of the Witwatersrand
Linda Iaccheri
Tags
Summary
This document provides a description of greisens and related ore deposits, focusing on their formation, types, and characteristics. The text details the hydrothermal processes involved in the formation of these deposits and highlights their association with specific metal mineralizations. Examples of greisen deposits from various locations are also included.
Full Transcript
GEOL3051A L15 Greisens and related ore deposits Dr Linda Iaccheri School of Geosciences [email protected] Greisen 2 3 Types of hydrothermal ore deposits: 1. Deposits that have a close spatial and temporal association to magmatic activity 2. Deposits that form du...
GEOL3051A L15 Greisens and related ore deposits Dr Linda Iaccheri School of Geosciences [email protected] Greisen 2 3 Types of hydrothermal ore deposits: 1. Deposits that have a close spatial and temporal association to magmatic activity 2. Deposits that form during periods of regional magmatism and tectonism, but are not clustered close to or around magmatic centres 3. Deposits that lack widespread or temporally related magmatic activity Greisens and their mineralisation Greisen = hydrothermal alteration of granitic rocks by hot magmatic fluids assemblage of quartz + mica which can be accompanied by other minerals typically including fluorite +/- topaz +/- tourmaline. Greisen systems are typically associated with Sn, W, Mo, Be, Bi, Li and F mineralisation. Greisens have been a major source for Sn cassiterite (SnO2), also tungsten scheelite (CaWO4), wolframite (Fe,Mn)WO4 they are generally small deposits associated with 4 granites. Hydrothermal alteration assemblage of granitic rocks Main silicate minerals: quartz + Muscovite Minor minerals: fluorite, tourmaline, topaz Greisens are similar to phyllic alteration of the porphyry copper deposits, but here hydrothermal fluids are rich in F and B, and deposits and alteration halos are much smaller Greisens often form aureoles around late-stage veins, particularly quartz- cassiterite- wolframite veins The greisens can either be enclosed within the granite (endo-greisen) or can be within the roof-rocks to the granite (exo-greisen) or sometimes both endo and exo. 5 A greisen is a hydrothermal assemblage of minerals formed from altered granite or pegmatite by late stage magmatic fluids exsolved from a granite magma. Metals are likely to be transported in the fluid by either Cl- or F- complexes Gangue minerals include: Quartz-mica (muscovite, lepidolite, Li-Fe biotites) ± topaz, tourmaline, fluorite The process of greisen formation is greisenisation Tourmaline W-bearing vein Cligga Head, with greisenisation Cornwall, UK alteration halo Examples of Greisen deposits: Cornwall (UK), Erzgebirge-Krusne hory (Germany-Czech Republic), Zaaiplaats (Bushveld, S. Africa), Nigerian A-type granites, Mt. Bishoff (Tasmania, Australia). Deposits are typically hosted in vein systems (Cornwall) or may be disseminated within the granite (Zaaiplaats) or both (Kinta Valley, Malaysia) Greisen deposits are smaller than porphyry copper deposits Important source of Sn (tin) in cassiterite and W By products: Cu, Zn, Bi and Mo Example: ZAAITPLAAS Tin Mine in South Africa Mineralisation in the Bushveld granites Mineralisation in the Bushveld granites ZAAIPLAAS Tin mineralisation The Bushveld Complex 2060-2054 Ma Within the Lebowa Granite Suite of the Bushveld Complex The Rustenburg Layered Suite Granites RUSTENBERG LAYERED SUITE Lebowa Granite Suite 2000 m thick Belong to Bushveld granites and overlie the Rustenburg Layered Suite 4000 m thick Considered A-type (anorogenic) granites: produced from mantle melting with variable amounts of crustal contamination. 400 - 1000 m thick chromitites 800 m thick 0 - 1600 m thick Lebowa Granite Suite: Bobbejaankop Granite: coarse-grained, miarolitic, disseminated ore, mineralised pipes. Lease Granite: fine-grained, miarolitic, low-grade ore, mineralised pipes; They are overlain by the Rashoop Granophyre Suite ZAAIPLAAS Tin mineralisation hosted in: The exsolved magmatic-hydrothermal fluids crystallise within the upper part of the intrusion and alter the intrusion itself: Greisen mineralisation Exsolved magmatic hydrothermal fluids crystallise within the pluton forming: - pockets and/or pipes of highly fractionated part of the granitic suite + mineralisation within the intrusion - Disseminated mineralisation occurs within the centre part of the granitic intrusions (cassiterite SnO2) in a zone beneath the Lease Granite N Mogalakwena Mine Lease Granite View on the old processing and concentration plant Old workings on the Bobbejaankop granite ZAAIPLAAS Tin mineralisation hosted in: pockets and/or pipes in the Lease Granite Disseminated mineralisation lower part of the Lease Granite Deposits may also include alteration and veins that extend from greisens into rocks that have different alteration assemblages Take the form of lenticular bodies, irregular patches or sheeted veins with low grade ore e.g. 0.25% Sn Sometimes disseminated ore may be mineable Pipe-like body in Bobbejaankop Granite Why are there holes? What is that Miarolitic Bobbejaankop Granite halo? Tourmaline pipes and greisen Greisen Alteration zoning: Pipe-like body in Bobbejaankop Granite Miarolitic Bobbejaankop Granite at the type locality Taylor (2005) Vonopartis (2021) Unaltered Bobbejaankop Sericite & Outer silicic Tourmaline + Cassiterite core alteration Quartz alteration zone Greisenisation Process of hydrothermal alteration of primary granite minerals (feldspar and muscovite) to aggregates of quartz and tourmaline Cassiterite-tourmaline-sulphide association at Zaaiplaats Zoning Outer silicic zone High grade cassiterite occurs in Core of sericite of bleached granite tourmaline-rich ore pipes and in ore and cassiterite pockets Extend from Bobbejaankop granite to Lease granite Pipe-like form (0.1-3 m diameter) and in circular in plan view May be horizontal or vertical with richest ore in vertical pipes Sericitic alteration common Tourmaline and Sulphides, scheelite and molybdenite quartz are also present Formation of the Zaaiplaats Tin Granites a. Early separation of a H2O-NaCl-CO2 fluid enriched in boron to produce pipe-like conduits by collection and upward flow beneath crystallisation fronts. b. Accumulation of fluorine-rich fluids evolved at a more advanced stage beneath downward advancing crystallization fronts to produce strongly miarolitic zones within the Lease Granite containing Vonopartis (2022) disseminated cassiterite. Formation of the Zaaiplaats Tin Granites c. Accumulation of fluorine-rich hydrothermal fluids producing a disseminated cassiterite mineralisation zone d. Pervasive alteration of the granites accompanied by filling of miarolitic cavities and pipes Vonopartis (2022) In Summary Granite related Sn-W deposits are commonly associated with greisenisation. Greisenisation can occur within and outside a granitic body. Metals are carries within magmatic-hydrothermal fluids within metal-Cl complexes. The magmatic-hydrothermal fluids interact with the wall- rocks/meteoric fluids. This causes alteration and changes the composition of the fluids – resulting in precipitation of metals. Greisenisation is an acidic reaction that commonly produces white- micas. Uses of tin Major producers Tin plating China Solder Thailand Bronze, brass and pewter Malaysia Chemicals for paint and glass Indonesia industry, plastics and insecticide Brazil Bolivia Linda Iaccheri School of Geosciences [email protected] 3 Types of hydrothermal ore deposits: 1. Deposits that have a close spatial and temporal association to magmatic activity 2. Deposits that form during periods of regional magmatism and tectonism, but are not clustered close to or around magmatic centres 3. Deposits that lack widespread or temporally related magmatic activity VOLCANOGENIC MASSIVE SULPHIDE (VMS or VHMS) DEPOSITS Massive sulphides mineralisation hosted in mafic volcanic rocks Not magmatic, but due to hydrothermal processes on mafic oceanic crust Complex ore deposits Cu-bearing massive pyrite deposits by-product Au, Ag, Pb, and Zn Major VMS deposits in the world Characteristics of VMS deposits VMS deposits occur worldwide throughout geological time Associated with altered volcanic mafic rocks (basalts) comprise massive sulphides (> 60%) which occur in lens-like or tabular bodies parallel to the volcanic stratigraphy or bedding The deposits usually zoned with massive syngenetic sulphide ores above an epigenetic footwall stockwork of vein and stringer sulphide mineralisation and hydrothermal alteration VMS deposits are usually zoned: massive syngenetic sulphide ores Altered basalt (host- rock) Epigenetic footwall of stockwork mineralisation and sulphides stringers Zoning in the massive syngenetic sulphide ores Core: chalcopyrite + pyrite + pyrrhotite + magnetite Grading upward: pyrite ± sphalerite ± galena Then: sphalerite ± galena ± pyrite ± barite Au, Ag usually are highest in the fringes with barite and cherty silica as common gangue accessory minerals Modern massive sulphides Submarine Alvin in 1977 in deep-sea on the East Pacific Rise, at 21°N, found 300°C hot springs emerging in plumes along the oceanic ridge, 2500 m below sea level - BLACK SMOKERS minerals precipitated out of the solution as soon as it emerged around the vents was a blanket of sulphides a modern analogue of Volcanic Massive Sulphides deposits found in old orogenic belts scientists discovered populations of previously unknown organisms living near the vents modern massive sulphides modern deposits are important because conditions of formation can be measured directly, and they show how little time is needed to produce appreciable tonnages of 'ore‘. Their rapid oxidation and destruction show the transitory nature of these deposits. Cross-section of a black smokers and massive sulphides Locations of formation of modern VMS deposits Schulz 2012. USGS Report. Modified after Schmincke (2004) and Galley and others (2007). https://www.interridge.org/ VMS deposits are related to the formation of Black smokers on oceanic floors “Black smokers” – a modern analogue for VMS deposit formation □ Black smokers are hot (up to 400 °C), metal charged, reduced, and slightly acidic hydrothermal fluids that vent onto the sea floor, usually in zones of extension and active volcanism along mid-ocean ridges □ The fluids originate from cold (2 °C), alkaline, oxidizing, and metal-deficient seawater. □ The fluids circulate through the basaltic ocean crust and, in so doing, scavenge metals to form the hydrothermal fluids □ Precipitation of base metals (mainly Cu and Zn transported as chloride complexes; Scott, 1997) and, in certain cases, precious metals (Au transported as a gold–bisulfide complex; Scott, 1997) is virtually instantaneous as the ore fluids mix with an essentially infinite volume of very cold (2–4 °C) seawater. 1 5 “Black smokers” – a modern analogue for VMS deposit formation □ Black smoker fluids usually vent through tube-like structures, called chimneys □ Chimneys are built out of a mixture of anhydrite, barite, and sulfide minerals such as pyrite, pyrrhotite, chalcopyrite, and sphalerite, as well as gangue opaline silica. □ Metal-charged fluids venting on the ocean floor point to the hydrothermal exhalative processes by which most, if not all, VMS deposits can be explained □ Drop in Temperature (seawater T few degrees) at the exit of the chimneys causes an instantaneous drop of the sulphide minerals Modelled hydrothermal fluids cell responsible for the formation of black smokers and VMS deposits close to mid- oceanic ridges 1 7 Exhalative vent site showing the construction of anhydrite– sulfide chimneys on top of a mound of massive sulfide mineralization. Fluid characteristics and circulation patterns in mid-ocean ridge environments that give rise to the formation of “black smokers” on the sea floor. 1 9 Formation of ophiolite-hosted VMS deposits Ocean water flows through fractures and at divergent plate normal faults in mafic oceanic crust and boundaries percolates deep into the oceanic crust via faults Seawater is progressively heated by the underlying mafic magma chamber, and becomes a hydrothermal fluid. A seawater convection cell reacts with basalts, alters them, and leaches metals. Formation of ophiolite-hosted VMS deposits Dissolved metals are transported back up at divergent boundaries to the ocean floor. When hot hydrothermal fluid mixes with the cold bottom ocean waters, there is a sudden decrease in temperature causes metals to precipitate from the solution Source of hydrothermal fluids and paths Seawater Percolate deep into the oceanic crust via normal faults Source of metals ore metals are derived and scavenged/leached from the mafic volcanic rocks that form the oceanic crust Cu and Zn are derived from alteration of pyroxenes Formation of ophiolite-hosted VMS deposits metals precipitated are sulphides and sulphates they form sulphide-rich mounds and chimneys around plumes of smoke Chimneys may be finger size of several metres high – chimneys may be active for periods of a few years to decades Black smokers - fine-grained pyrrhotite with minor sphalerite and pyrite White smokers -silica, barite and pyrite Major VMS deposits in the world VMS can be found in ophiolite (e.g. Cyprus and Oman) Ophiolite is a remnant of oceanic crust preserved in continental collision zones Ophiolitic sequences Simplified VMS ores associated with the basalts and pillow lavas; ophiolitic sequence massive ores dominated by pyrite together with variable amounts of chalcopyrite, sphalerite and pyrrhotite massive sulphide overlie stringer zones (stockwork mineralisation) Section through a typical ophiolite- hosted, Cyprus- type VMS deposit. Footwall rocks may consist of a sheeted dyke complex and the associated volcanics are often pillowed and have a tholeiitic composition. Ore deposits associated with ophiolites GEOL3051A L17 Hydrothermal deposits - Linda Iaccheri IOCG School of Geosciences [email protected] 3 Types of hydrothermal ore deposits: 1. Deposits that have a close spatial and temporal association to magmatic activity 2. Deposits that form during periods of regional magmatism and tectonism, but are not clustered close to or around magmatic centres 3. Deposits that lack widespread or temporally related magmatic activity What are IOCGs? Hydrothermal ore deposits characterized by large amounts of hydrothermally precipitated iron oxide (magnetite and/or haematite) hematitic alteration with associated copper-iron sulfides and gold. As we will see, it is a very broad group of ore deposits. IOCG Deposits did not exist as a deposit-type before the discovery of Olympic Dam in 1975- 1976. The first academic paper defining them is: Hitzman et al., (1992) Geological characteristics and tectonic setting of Proterozoic iron oxide (Cu-U-Au-REE) deposits. Once Olympic Dam was discovered, evaluated, and found to be the world’s: 5th largest copper deposit, 3rd largest gold deposit and the world’s largest uranium deposit – Everybody wants one!!! Now there are IOCG’s all over the world. The Gawler Craton & Olympic Dam The Olympic Dam IOCG deposit is located in the north of the Gawler craton (South Australia), fairly close to the craton margin. The Gawler Craton and the discovery of Olympic Dam Olympic Dam has been discovered by geophysics: it is buried and not exposed, in an area of very flat topography: Before Olympic Dam discovery Olympic Dam today 7 Olympic Dam: Discovery in 1975 Western Mining Tim O’Driscoll lifelong interest in regional and continental linear structures Olympic Dam: Discovery in 1975 1974 selected targets for drilling campaign based on regional linear discontinuities and magnetic anomalies Beginning of modern geological exploration. World map of IOCG deposits and significant Na(-Ca) and K alterations from Barton (2014) Diversity is indeed a hallmark of the IOCG deposit class: diversity within the IOCG deposits in terms of the mineralogy of the iron oxide phase, with the terms ‘hematite-group’ and ‘magnetite-group’ The sources of fluids associated with IOCG deposits can vary from magmatic to sedimentary A large spectrum of alteration minerals associated with IOCG deposits IOCG deposits are complex ore deposits economic elements like uranium, iron, lead, zinc, silver, bismuth and rare earth elements, in addition to the main ore forming elements copper and gold Copper in IOCGs, Olympic Dam has more Cu than two of the largest porphyry copper deposits (Bingham Canyon and Grasberg) Groves et al., 2010 Gold in IOCGs, Olympic Dam is a more important gold deposit than any VMS, and any porphyry Au system Table with resource data for IOCG deposits from Australia and South America Mt Cu (%) U3O8 (ppm) Au (ppm) Other Gawler Craton (South Australia) Olympic Dam 8,330 0.8 280 0.76 3.95 g/t Ag Prominent Hill 152.8 1.18 0.48 Hillside 170 0.7 0.2 Wilcherry Hill 60 31% Fe Carapateena 203 1.13 0.56 South America Punta del Cobre (Chile) La Candelaria (Chile) 600 0.95 0.2 3 g/t Ag Mantoverde (Chile) 580 ~0.5 Mantos Blanco (Chile) >500 1.0 Cloncurry District (Queensland, Australia) Ernest Henry 122 1.18 0.55 Mt Elliot 475 0.5 0.3 South America Cristalino (Brazil) 500 1.0 0.2-0.3 Sossego (Brazil) 355 1.1 0.28 Igarape Bahia (Brazil) >30 2 Salobo (Salobo) 986 0.82 0.49 It has been difficult obtaining a consensus on what constitutes or characterises a “typical” IOCG, because there is diversity within the deposits. critical features of the IOCG sensu stricto deposits IOCG Sensu Stricto (strictly speaking): hydrothermal deposits that contain economic Cu + Au grades (generally 0.7-1.5% Cu; 0.8 – 1 g/t Au); Structurally controlled and commonly containing significant volumes of breccia; Commonly associated with pre-sulfide sodic or sodic-calcic alteration on a large, often regional scale relative to economic mineralization; IOCG Sensu Stricto (strictly speaking, cont.): Contains abundant low-Ti iron oxides (hematite or magnetite) associated with, but generally earlier than, Fe-Cu sulfides; They have low-S sulfides (= lack abundant pyrite); They generally have elevated (anomalous) Ce and La (REE); IOCG Sensu Stricto (strictly speaking, cont.): Lack abundant quartz veins or silicification; Show a clear temporal, but often not close spatial, relationship to major magmatic intrusions; Form from highly oxidized, saline fluids. Characteristics of IOCG's Age: Late Archean to Pliocene (basically most of Earth’s geological history). However, most of them formed in the Archean to mid Proterozoic. Characteristics of IOCG's Tectonic setting: - extremely variable - Associated to major crustal structure and shear zones - Most major districts appear to be “anorogenic.” Mesozoic Andean (and Mexican) deposits in subduction-related settings – probably fundamentally different from Precambrian examples. Characteristics of IOCG's Association with igneous activity: the vast majority are spatially related to magmatic event, but this does NOT mean genetically related No specific magma composition is related to the deposits. Extremes: Raul, Peru, South America – direct spatial, temporal, and genetic relationship to dacite dome field Wernecke, Yukon, Canada – no evidence of spatial, temporal, or genetic relationship to any igneous rocks (driven by circulating basinal brines in evaporite basin?) Characteristics of IOCG's Structural control: Localised along high-angle (most common) or low-angle faults which are commonly splays off crustal-scale structures. Very commonly in zones of ductile-brittle transition with sulphides precipitated during transition(s) between ductile and brittle deformation or in the brittle phase. Commonly with large volumes of breccia formed in different regimes (in- situ replacement breccias and brittle structural breccias). Characteristics of IOCG's Mineralogy: - Iron oxide minerals (magnetite, hematite) dominant, - minor sulfides (generally chalcopyrite, lesser pyrite), - gangue: feldspar, mica, calc-silicate minerals, carbonates, barite, (quartz) Large alteration footprint Alteration minerals change with protolith and depth and/or distance from the hydrothermal fluid conduit. Characteristics of IOCG's IOCGs – Elemental Variation A hallmark of these deposits is that each district (if not deposit) has a slightly different trace element signature: –Olympic Dam, Gawler Craton, Australia (U, Ag, F) –Salobo, Carajas district, Brazil (F, anomalous As, Co, Mo, Ni) –Cloncurry, Australia – Mt. Dore (Zn, Pb); Mt. Cobalt (Co); –Candelaria, Chile (Zn, Co, Ag) –Great Bear, NWT Canada – Nico (Co, Bi); Port Radium (U) –Guelb Moghrein, Mauritania (anomalous Bi, Te) This reflects the geochemistry of the crust through which the hydrothermal fluids moved. 24 Characteristics of IOCG's Alteration: There is a variety of alteration types, generally with regular time- space pattern Characteristic alteration type of IOCGs is sodic or sodic- calcic Most IOCG sulfides ore with potassic, calcic, or hydrolytic alteration assemblages. Vertical Variation in IOCG System Alteration Patterns 5 km 1 km Hydrolytic alteration Potassic/calcic alteration Sodic/sodic-calcic alteration IOCG deposits Mgt-apatite (IOA) deposits (“Kiruna-type”) Vertical Variation in IOCG System Alteration Pb, Zn? Patterns Ag, Co, U, S 5 km 1 km Fe, Cu, S, Au Hydrolytic alteration Potassic/calcic alteration Fe Sodic/sodic-calcic alteration IOCG deposits Fe Mgt-apatite (IOA) deposits (“Kiruna-type”) IOCGs Alteration Sodic and Sodic-calcic: typical of this deposit type Alteration mineralogy is wallrock controlled: » Albite (scapolite) in more felsic host rocks » Albite-actinolite-diopside (scapolite) in more mafic host rocks Sodic Alteration (NB albite is not always a white colour – it “White Rock” can also be red) Rhyodacite ash flow altered to albite with disseminated hematite (“red “ rock”), footwall of Kiruna magnetite orebody, Sweden. Massively albitized diorite with minor chlorite- magnetite. El Espino, Chile Felsic volcanic rocks altered to albite (red with hematite“dust”) and actinolite. Archer Prospect, Cloncurry district, Australia. IOCGs Alteration Potassic alteration: Potassium-bearing minerals include both biotite and orthoclase. » Orthoclase in more felsic rocks » Biotite in more mafic rocks Potassic zones with variably abundant magnetite - generally >10%. High level systems may have hematite at expense of magnetite. IOCGs Alteration Potassic alteration: Potassic alteration primarily as wall-rock replacement, not vein stockwork at scale of hand specimen. Quartz veinlets are rare. Ore bearing portion of many systems. Potassic alteration – Rhyolite ash flow altered to Potassic Feldspar: orthoclase (Ksp) in the upper Hauki hematite ores of the Kiruna system, Sweden NB: it is sometimes difficult to distinguish albitic alteration from potassic alteration in hand specimen. Outcrop Potassic alteration – Biotite: Andesite altered to massive Bio Mgt biotite-magnetite rock. Candelaria deposit, Chile Thin section IOCGs Alteration Calcic: Calcic Not recognized at time of early papers (early 1990’s). Now seen to be major component of many IOCG systems. Calc-silicate minerals (actinolite, diopside, hornblende, grunerite, garnet) Calcic zones with variably abundant magnetite - generally >10%. Grunerite-garnet clasts (Calcic Calcic Alteration alteration) in a biotite- grunerite matrix (Potassic-calcic alteration). Salobo deposit, Carajas district, Brazil Coarse-grained pyroxene (diopside) with later vug filling calcite. (“skarn”). Mt. Elliot, Cloncurry district, Australia Calcareous siltstone altered to diopside- magnetite and cut by later sulfides. Mt. Elliot Calcic Alteration Calcareous siltstone altered to magnetite- epidote-garnet. Siltstone replaced by albite-epidote- El Espino, Chile garnet-magnetite. Transitional with sodic- calcic alteration. El Espino, Chile IOCGs Alteration Hydrolytic or HSCC (Haematite-Sericite-Carbonate-Chlorite) alteration: Generally developed at high levels in the systems. Footprint generally small relative to other alteration types. May be difficult to distinguish from “epithermal” alteration types. IOCGs Alteration Hydrolytic or HSCC (Haematite-Sericite-Carbonate-Chlorite) alteration: Mineral assemblage of chlorite-sericite-carbonate-(quartz) Haematite is the dominant iron oxide May contain ore (HSCC alteration encases all major deposits on Gawler Craton, but few other economic systems recognized elsewhere in the world). Hydrolytic Alteration Edge of Olympic Dam (Austalia) breccia body with wallrock granite altered to sericite- carbonate-hematite-(chlorite). Volcanic rock altered to sericite- carbonate in hematite matrix. Prominent Hill, Australia Hydrolytic Alteration Biotitized clasts of volcanic rocks (potassic alteration) in a matrix of sericite- carbonate-(haematite) with chalcopyrite. Sossego deposit, Carajas district, Brazil Dacites altered to sericite – siderite - haematite - (fluorite) with late calcite veins. San Xavier deposit, Sonora, Mexico IOCG Ore System Alteration Variable by district Variability partially depends on local host rock types. Variability probably depends on: 1. total hydrothermal fluid volumes (water/rock ratio), 2. permeability of rock mass, 3. magmatic/host rock brine ratio of fluids, 4. regional wallrock composition, and 5. depth of erosion. Energy: Driven by Lower Crustal Processes Heat + H2O Very large hydrothermal systems – driven by intrusions at base of crust which provide high heat flux. This may cause higher level intrusions which may be temporally (and overall genetically) related to deposits. Source of Metal Content of IOCGs Need overall oxidized rock package (magnetite-bearing granite or granitic gneiss, subaerial volcanic rocks, siiciclastic dominant sediments – not black shales, reduced carbonates) to buffer hydrothermal fluids to oxidized state. Each IOCG displays a different metal “fingerprint” (U, Ag, Zn, Mo, Co, Bi, LREE, etc.). This is expected as the enormous hydrothermal systems which forms as these fluids “see” different crust along their flow path. Fluids & Transport in the IOCG Ore System Large volumes of fluids (huge alteration areas) – much more fluid than available from cooling of individual plutons or even batholiths. Structural pathways guide fluids. IOCG Ore System Traps …Still very poorly known! Fluid inclusion work + IOCG mineral assemblage (sulphide, particularly pyrite, poor) suggests need to mix dominant hydrothermal fluid with another fluid containing reduced sulphur at the level of the deposits. Lack of significant quartz in most systems suggests that temperature drop alone is not important (fluids must have been silica saturated after moving through crust). Redox trap potential has probably been under appreciated. 44 Vertical Variation in IOCG System Alteration Pb, Zn? Patterns Ag, Co, U, S 5 km 1 km Fe, Cu, S, Au Hydrolytic alteration Potassic/calcic alteration Fe Sodic/sodic-calcic alteration IOCG deposit Fe Mgt-apatite deposit (“Kiruna-type”) Linda Iaccheri School of Geosciences [email protected] DRIVERS of hydrothermal fluids to create hydrothermal ore deposits (a)Gravity-driven fluid flow in response to the creation of a hydrostatic head in an area of uplift. (b)Orogeny-driven fluid flow in response to rock compaction in a fold and thrust belt. H2O Vapour CO2 globule (c)Thermally driven fluid flow in the ocean crust in response to high heat flow at mid- Various tectonic scenarios illustrating the mechanisms by which major fluid movements in the Earth’s crust take place (Robb 2005, modified after ocean ridges Garven and Raffensperger, 1997) & Microphotograph showing the typical appearance of a H2O–CO2bearing fluid inclusion (Robb, 2005) DRIVERS of hydrothermal fluids to create hydrothermal ore deposits (d) Thermally driven fluid flow in a permeable unit within a basin or rift. (e)Dilatancy- or fault- driven fluid flow along major, seismically active structural features by seismic pumping and H2O CO2 Vapour globule fault valve mechanisms Various tectonic scenarios illustrating the mechanisms by which major fluid movements in the Earth’s crust take place (Robb 2005, modified after Garven and Raffensperger, 1997) & Microphotograph showing the typical appearance of a H2O–CO2bearing fluid inclusion (Robb, 2005) Relevant for strata- bound Cu deposits Relevant for orogenic Au deposits H2O Vapour CO2 globule Various tectonic scenarios illustrating the mechanisms by which major fluid movements in the Earth’s crust take place (Robb 2005, modified after Garven and Raffensperger, 1997) & Microphotograph showing the typical appearance of a H2O–CO2bearing fluid inclusion (Robb, 2005) Summary Hydrothermal ore deposits: enrichment by selective dissolution, transport and precipitation of different hydrothermal fluids Types of hydrothermal ore deposits: 1. Deposits that have a close spatial and temporal association to magmatic activity 2. Deposits which form during periods of regional magmatism and tectonism, but are not clustered close to or around magmatic centres 3. Deposits that lack widespread or temporally related magmatic activity Examples of a deposit that lacks widespread or temporally related magmatic activity are: 1. Sediment-hosted strata-bound copper deposits 2. Orogenic Au deposits Sediment-hosted stratabound copper deposits are some of the world’s important sources of copper – second after porphyry copper are widely distributed in space and time they may have consistent grades and extensive lateral continuity hydrothermal ore deposits Represent most important global source of Co as well as many other metals – Pb, Zn, Ag, U, Au, PGE. Global distribution of sediment-hosted copper districts: Neoproterozoic Katangan basin of the Central African Copperbelt Permian Zechstein basin of Europe (the Kupferschiefer) Devonian to Carboniferous Chu-Saryasu basin of Kazakhstan Paleoproterozoic Kodaro - (Hitzman et al., 2010) Udokan basin of Siberia Stratabound copper deposits – general model Typically hosted within an intra-continental rift and within related sedimentary sequence Early deposition of oxidised (red-bed) aeolian assemblage rapidly oxidised during burial and diagenesis during Graben formation A shallow marine transgression deposited a more reduced assemblage of shales, carbonates and evaporites in the rift basin Stratabound copper deposits – general model Basin-derived fluids circulation was promoted by high heat flow associated with rifting along active faults Fluids moved through porous clastic sediments Circulating water was saline, relatively oxidised and pH neutral. Metals, in particular Cu, derived from alteration of detrital magnetite, biotite, hornblende, and pyroxene Stratabound copper deposits – general model Many deposits of this type but are dominated by the huge resources of two regions: 1. Kupferschiefer (copper-shale) of central Europe 2. Central African Copperbelt The Central African Copper Belt (CACB) It extends in a broad arc ~600 x 100 km, from Luanshya in Zambia, to the Kolwezi area of Katanga Province in DRC into eastern Angola. The CAC is one of the great metallogenic provinces of Africa. It contains some of the largest and richest copper, cobalt and uranium deposits in the world at Nchanga, Konkola and Tenke Fungurumi It has ~10% of world’s copper, and 34% of the world’s cobalt reserves. The geological context of the Central African Copper Belt (CACB) The mineralisation is related to the opening and closing of the LUFILIAN ARC CACB occurs within the Lufilian Arc Lufilian Arc is part of the system of Pan-African Orogenic Belts formed during the amalgamation of Gondwana (~550 Ma): The Lufilian Arc formed as a result of the Pan- African collision between the Kalahari and Congo cratons Pan-African orogeny The Pan-African orogeny was a major tectono- thermal event, occurring at around 500Ma During this event a number of mobile belts formed, surrounding older cratons This brought together old continental cratons, such as West African, Congo, Kalahari andTanzania, forming the supercontinent Gondwana Map of Supercontinent Gondwana at the end of Neoproterozoic time (-540 Ma) after Kusky et al, 2003 Tectonic evolution of the Lufilian Arc Regional rifting associated with the dispersal of the supercontinent Rodinia (~900Ma), responsible for regional rifting Formation of the Lufilian basin and deposition of the copper-bearing Katanga succession which contains volcanic rocks dated between 765 and 735 Ma. Amalgamation of Gondwana (~530Ma), causing regional compression Folding during extensional basin collapse or basin inversion CENTRAL AFRICAN COPPERBELT (CACB) – STRATIGRAPHY of the KATANGA SUPERGROUP Katangan supergroup ~5 – 7km thick Deposited in an intracratonic basin Exact age of sediments are poorly constrained thin sequence of rocks for long period of time (~30Ma) Correlation from marker units, glacial diamictites (Sturtian and Marinoan glaciation) Goes from an initial stage of rifting, renewed extension, glacial events as well as salt diapirism (after Hitzman, 2012) KATANGAN SUPERGROUP Deposited in a rifted basin between 765 and 735 Ma. Immature fluvial sandstones and arkoses The hydrothermal system and ore formation 20 Cross-sectional models of basin-scale fluid flow in sediment-hosted stratiform copper systems (Hitzman, 2005) A)Compaction driven fluid escape. B)Topographically driven gravity recharge and escape. C)Basinal fluid convection with impermeable basin margins. D)Topographic gravity-driven recharge and bittern brine entering the basin from overlying evaporites. E)Red-beds charged with brines from overlying evaporites. F)Red-bed aquifer charged with brine from dissolution of evaporite during episodic diapir growth. Evaporites – SEAL Reducing strata – TRAP Red Beds – SOURCE Evaporites as a source of sulfur 23 CENTRAL AFRICAN COPPERBELT (CACB) – CRUST ARCHITECTURE CH4 CH4 CH4 CH4 CH4 CH4 CH4 CH4 CH4 CH4 CH4 SO42- SO2- CH4 4 Proposed model showing north directed thrusting circulation and chemical reactions forming ore deposits OPEN Convection below evaporites Movement along faults (paths) Basinal fluid circulation Oxidised Basement Redox reactions are key! Red Beds – SOURCE CLOSED Evaporites – SEAL Reducing strata – TRAP Closed systems are more favourable as they are more likely to host larger deposits Schematic cross sections of sedimentary basins (Hitzman et al., 2010) Source of metals Basement erosion resulted in Cu-rich red beds in basin Source of fluids basin dewatering during diagenesis transported Cu. Evaporites within the succession provided Cl- and SO4. Cu was leached from red beds. Lack of evaporites reduces the chance of getting ore deposits because they may be the source of sulphur. Brown, A.C. 2014 Low-temperature aqueous brines leached metals from the oxidised red-beds and basement rocks, transport them as cuprous-chloride complexes deposited them at the redox barrier, possibly as a result of fluid mixing. Reduced Oxidised the boundary between magnetite and haematite stability reflection a REDOX transition/boundary, possibly as a result of fluid mixing. Central African Copper Belt summary Although the CACB deposits occur in a variety of stratigraphic positions most are hosted by reduced rocks that overlie oxidised rocks. Precipitation of sulphides at multiple REDOX interfaces throughout the basin where oxidised metal-charged basinal brines met reduced sediments/fluids with a high organic content beneath an evaporite seal. Reductants may be in situ (carbonaceous material, diagenetic pyrite) or may have been mobile (H2S, CH4, petroleum). Ores are generally zoned at a district scale: barren/haematite – native copper – chalcocite – bornite – chalcopyrite – Pb/Zn/Co sulphides- pyrite 33 Central African Copper Belt summary Most deposits formed close to syn-sedimentary faults, particularly the early basin- margin normal faults and there is also a structural control on the deposits (access for hydrothermal fluids) Fluids show little or no evidence of temperatures in excess of 120ºC The deposits are primarily the product of low-temperature aqueous brines which flowed through large volumes of sedimentary rocks where they leached, transported and ultimately deposited the metals. The source of the metals is thought to be the underlying red- beds. Linda Iaccheri School of Geosciences Agnes Au Mine [email protected] Barberton Greenstone Belt Types of hydrothermal ore deposits: 1. Deposits that have a close spatial and temporal association to magmatic activity 2. Deposits which form during periods of regional magmatism and tectonism, but are not clustered close to or around magmatic centres 3. Deposits that lack widespread or temporally related magmatic activity Orogenic Gold deposits a type of hydrothermal mineral deposit. More than 75% of the gold recovered by humans through history belongs to the class of orogenic gold deposits. V.G. Orogenic Gold deposits are also known as - Lode Gold deposits - Quartz-vein Gold deposits - Gold-only deposits - Mesothermal Gold deposits - Payable Gold deposits Mostly mined for Au only Rarely by-products As and Sb Most deposits are small few large world-class deposits Orogenic Gold fundamentals: Deposits form in actively evolving orogenic belts They form at high pressure (4 to 15 km in the crust) Temperature around 300 to 450 degrees (Mesothermal) Many orogenic gold deposits are in Archean terranes (on cratons) Mineralisation hosted in regionally high-grade metamorphic rocks Dominantly along fault/shear zones and tectonically active regions Orogenic gold deposits form in response to elevated heat and fluid fluxes during compressional to transpressional deformation in metamorphic belts of accretionary and collisional orogens. Orogenic Gold deposits characteristics – CRUSTAL ARCHITECTURE localization of deposits in orogenic belts, along/near boundaries of terranes/tectonic units. Orogenic Gold deposits characteristics – GEOCHRONOLOGY The timing of ore formation is syn- to post-peak metamorphism and late deformation events Orogenic Gold deposits characteristics – CONDUITS structures controlling fluid flow are crustal-scale shear zones. Orogenic gold deposits are located at shallow to mid-crustal levels, most typically in greenschist and amphibolite facies metamorphic rocks, in brittle/ductile and brittle deformation zones with fault- controlled pressure fluctuation. The distribution of lode gold deposits is strongly controlled by faults and shear zones Huge volumes of fluid needed to transport the metals contained in the multitude of deposits Orogenic Gold deposits characteristics – FLUIDS COMPOSITIONS What type of fluid is responsible for orogenic Au-deposits and what was its source? Two common ways to answer this: analyses of fluid inclusions in minerals isotopic signatures of minerals Fluid inclusions – bubbles of fluid + gas trapped in minerals Their composition help establishing conditions under which they existed Orogenic Gold - FLUIDS COMPOSITIONS H 2 O-CO 2 (±CH4, N2, H2S) inclusions indicate: low to moderate salinity (mainly 3-7 wt % equivalent NaCl) P-T conditions: 200° to 350°C and 1 to 3 kbar pH near neutral slightly alkaline (5.2 to 6.8), typical of fluid in equilibrium with many common silicate rock types. Pettke et al., 2000 Orogenic Gold deposits characteristics – FLUIDS SOURCES fluids are not primary magmatic metamorphic fluids liberated during prograde, regional metamorphism (CO2, low salinity diagnostic) Deeply convecting meteoric fluids in tectonically active region Orogenic Gold deposits characteristics - FLUIDS Devolization of volcanic and sedimentary rocks during metamorphism or the sediment laden oceanic slab during subduction supports generation of large volume fluids of crustal/sub-crustal origin. Migration of these fluids along crustal-scale fault systems mobilize and transport the ore forming elements. Addition of fluids from other local sources along the pathways of the regional scale fluid flow is not excluded. Local admixing of fluids of different origins to the regional scale fluid flow system may explain the observed variation in metal associations and stable/radiogenic isotope properties among deposits occurring in the same orogenic belt and province. Orogenic Gold deposits characteristics – FLUID TRANSPORT and FOCUSING The unifying characteristic of orogenic Au- deposits is the structural control on fluid flow, and directly/indirectly on Au precipitation Deformation enables the large scale, high flux, fluid flow responsible for these deposits by increasing permeability o at ~grain scale (inter- and intra- crystalline strain) – DUCTILE o fracture permeability - BRITTLE enables focusing of the fluid flow Orogenic Gold deposits characteristics – GOLD TRANSPORT How is gold transported in fluids? - Mainly as a bisulfide complex i.e. Au(HS)2 in mesozonal environments At low fluid/rock ratios - and high T Au is soluble enough to be scavenged from range of hosts during alteration AuCl2 rarely occurs (oxidixing, saline, acidic conditions) and is not a good gold complex under fluid conditions present Base metals usually complexed with chloride (Cl), and low chloride abundance (low NaCl) explains why they are rare in the orogenic Au setting Orogenic Gold deposits characteristics – GOLD EXSOLUTION FROM FLUIDS To exsolve the dissolved and transported gold from the fluid: need to lower Au solubility and/or destabilize bisulfide (Au(HS) - ) complex Under fluid and crustal depth conditions that characterize orogenic gold deposits, gold precipitation is most often caused by: 1. fluid – wall rock reactions Au replacing minerals in altered wall rock (structurally and/or lithologically controlled) 2. pressure fluctuations crystallization of Au in veins/fractures Orogenic gold Trap – pressure fluctuations in veins Dube & Gosselin, 2007 after Robert, 1990 At the deposit scale – veins are very common Vein splay structure at the Wonga mine, Australia Vein geometry at Sigma gold mine, Quebec Miller and Wilson, 2004 Orogenic gold Trap – pressure fluctuations in veins In veins – sudden decreases in pressure can cause “boiling” of fluids (really phase separation – volatiles separate) e.g., H2S and CO2 preferentially partitions into volatile phase 1 cm Pf decrease occurs as part of the earthquake Bulyanhulu, Tanzania (C. Chamberlain) cycle Orogenic gold Trap – pressure fluctuations in veins Cyclic pressure fluctuations: Faults as valve behavior Each incremental seismic event results in short episodes of reduced pressure leading to Au exsolution from the fluid (via direct drops in solubility and through phase separation) Sibson, 2001 Formation of crack-seal growth of veins Where the vein filling is made up by a series of small increments of microcracks each followed by a period of precipitation – typically forming complex veins. This typically occurs in the brittle-ductile transition or in the ductile regime. greater complexity than the relatively simple open-space filling. Typically found in orogenic/lode-gold systems. Complex quartz vein formed by repeated crack-seal events from a thrust sheet a about 10 km depth (Fisher & Byrne, 1990) 22 Photographs of complex, crack-seal veins, including a vein breccia from an orogenic / lode gold deposit in the Abitibi Belt, Canada. (Chi & The diagram on the right is an overall Guha, 2011 Geosci Front) interpretation of the sequence of opening and closing in this deposit. 23 Brittle fracture permeability Kenworthy & Hagemann (2007) Orogenic Gold deposits characteristics – LOCALISATION OF DEPOSITS Orogenic gold deposits are typically located along faults irregularities and jogs Faults grow by linking individual fault segments linkage zones = Jogs High fracture density, aperture, and connectivity along jogs favors high fluid flux along the jog axis. Structural controls at deposit scale gold is spatially related to: low displacement faults and brittle-ductile shear zones Competency contrast between lithologies Modified from Hagemann & Cassidy (2000) Distribution of gold deposits and prospects around the Boulder-Lefroy fault system in the St. Ives goldfield, Western Australia. A major contractional jog occurs in the Boulder-Lefroy fault system at the Victory complex. Main-shock rupture is modeled to occur southeast of the jog. Saddle Reef mineralization, Bendigo, Australia Sibson and Scott, 1998 Orogenic Gold deposits characteristics - TRAPS, FOOTPRINTS, VECTORS The precipitation of gold is commonly triggered by phase separation processes in the parent fluids along the fault networks between rocks with contrasting competencies due to transient change in the tectonic stress field and sulphidation/carbonatization of host rocks during fluid-rock interaction. The ore-forming fluids have a wide range of temperatures (250- 700°C), aqueous carbonic composition with low to moderate salinities, and reduced, near-neutral chemistry. SUMMARY: Source – Transport - Trap Source of fluids Metamorphic Deeply convecting meteoric fluids Transport, (i) medium(s); (ii) scale of transport; (iii) process of transport H2O rich fluids Laterally 100s km; vertically ~5-15 km Structurally controlled and structurally facilitated FOCUSED fluid flow Trap (process controlling Au precipitation and veining) structurally enhanced fluid:rock interaction structurally induced pressure drops and Au exsolution from the fluid (phase separation mainly) Shape of the mineralisation Deposits are Lode-shape= they are tabular or planar bodies (example a single continuous quartz vein) Dimension of the lode from 2 meters to hundreds of meters along the strike Larger deposits are found in networks of veins or mineralized shear zones Lodes and veins are steeply dipping and plunging. Wiluna Gold Camp, Yilgarn Craton East Lode deposit Many orogenic gold deposits are in Archean terranes (on cratons) Orogenic Gold deposits in the West African Craton Orogenic Gold deposits in the Yilgarn Craton, Western Australia Orogenic Gold deposits in the Superior Craton, Canada Abitibi greenstone belt – principal breaks and significant gold deposits At the camp scale – gold is Porcupine-Destor Fault spatially related to: First-order (transcrustal) fault zones (10 -100’s km in length) Larder Lake-Cadillac Fault Large-scale structural irregularities, such as bends along major faults/shear zones, anticlines, synclines Dube and Gosselin, 2007 Orogenic Gold deposits in the Kaapvaal Craton, South Africa The Barberton Greenstone Belt Orogenic Gold deposits in the Kaapvaal Craton, South Africa Lily Consort The Barberton Sheba Greenstone Belt Fairview Agnes/Galaxy Clusters of mines along main faults and shear-zones Large-scale structural irregularities such as bends along major faults/shear zones, anticlines, synclines Main Archaean greenstone belts of the Kaapvaal Craton Barberton Greenstone Belt (BGB) □ The Barberton Greenstone Belt (BGB) is recognized as one of the World's oldest and best preserved, mineralized greenstone belts. □ The belt forms a folded and highly faulted keel of volcanic and sedimentary rocks, enveloped and intruded by a variety of younger granitic rocks, the oldest of which were emplaced as a series of diapiric plutons. □ The oldest successions, which now form the periphery of the belt, are komatiite-dominated volcanic rocks with related subvolcanic layered ultramafic complexes, the latter bodies occurring mainly along the northern flank. □ These, together with overlying mafic to felsic volcanic rocks and interlayered chert horizons, form the Onverwacht Group and date back to 3500 Ma (De Ronde and De Wit, 1994). The Barberton Greenstone Belt consists of a sedimentary sequence of the Barberton Supergroup: Overwacht Group (oldest) around 3.5 Ga Fig Tree Group Moodies Group (youngest) around 3.2 Ga And associated intrusions, called TTG (plutons) Generalized stratigraphy of the Barberton Supergroup Group Age: oldest to youngest Composition and Formation (Ma = Million Years) Moodies 3225 – 3215 Ma Mudrocks, sandstones and conglomerates in alluvial, fluvial, deltaic, shoreline and tidal environment Fig Tree 3255 – 3225 Ma Sandstones, shales and conglomerates, mostly Group deposited in deep water; chemical sediments, including chert, BIF, baryte; some volcanic strata Onverwacht 3550 – 3260 Ma Komatiites and basalts; occur as fine-grained tuffs, pillow lavas, lava flows, breccias, some chert □ Large-scale fold structures associated with major strike faults, sub-parallel to the northeast- trending greenstone lithologies are a feature of the belt. □ Gold mineralisation is closely associated with these fault systems, especially the Sheba Fault □ Most important mines in the Barberton belt: the Sheba, Fairview, and New Consort mines which together have contributed ~76% of the gold produced from the Barberton goldfield. Geological setting of the Sheba-Fairview Complex (SFC) illustrating the main mineralized structures in carbonate- altered komatiites and argillaceous rocks to the south and in quartzitic rocks of the Eureka Syncline to the north of the Sheba Fault (modified after Wagener and Wiegand, 1986) North-south geological section (A and B) through the Sheba mine showing tight anticlines defined by rocks of the Zwartkoppie Formation within argillite of the Fig Tree Group. 5 different styles of mineralization are depicted: (i) Zwartkoppie section type (ii) Main Reef Complex type (iii) Golden Quarry type (iv) fractures in quartzite (v) Royal Sheba type. Modified after Wagener and Wiegand (1986). □ Gold mineralisation at the Sheba-Fairview Complex occurs in a variety of lithological and structural settings each with its own seemingly unique characteristics. □ Although belonging to a single large, compositionally uniform, hydrothermal mineralising system, there is little evidence of ore types merging and changing with depth or along strike. □ The distinctive alteration assemblages and textures which formed are largely a function of the composition of the host rocks at the various sites of mineralisation. □ Five main styles of mineralisation can be distinguished. Green fuchsitic quartz-magnesite schist from the Zwartkoppie Formation, Sheba Mine. Visible gold in quartz veining in highly fractured and brecciated black Zwartkoppie chert. Consort Contact reef, a sporadically mineralised and folded chert “Bar” containing black chert and arsenopyrite-rich layers at the contact of the hornfelsic Fig Tree Group shales and underlying altered ultramafic schist of the Onverwacht Group. Linda Iaccheri School of Geosciences [email protected] Sedimentary Ore-Forming Processes Processes that are syngenetic with respect to the host sediments and where the ores are themselves sediments or part of the sedimentary sequence. 2 Sedimentary Ore-Forming Processes Most major syn-sedimentary ore deposits tend to occur in a limited range of basin types: Shoreline and fluvial placer deposits (example: heavy minerals such as gold, cassiterite, diamonds, and zircon–ilmenite– rutile black sands) Passive continental margin chemical sedimentary ores (for example, banded iron formations and ironstones, bedded Mn deposits, and phosphate ores) Types of Sedimentary Ore-Forming Processes: 1. the accumulation of heavy, detrital minerals and the formation of placer deposits; 2. the precipitation mechanisms that give rise to Fe and Mn concentrations in chemical sediments; 3. the origins of oil and gas deposits, as well as of coalification processes. 1. Accumulation of clastic sediments and heavy mineral concentration formation of Placer deposits □ The formation of placer deposits is a process of sorting light from heavy minerals during sedimentation. □ A placer deposit is one in which dense (or “heavy”) detrital minerals are concentrated during sediment deposition Placer deposits Placer deposits can contain a wide variety of minerals and metals, including: gold, uraninite diamond cassiterite, ilmenite, rutile, and zircon. Placer deposits examples in South Africa Placer deposits examples in South Africa A well known example of an ancient placer deposit is the late Archean Witwatersrand Basin. Geologically more recent occurrences include: the diamond placers of the Orange River system and the western coastline of southern Africa, and the heavy mineral sands mined at Richards Bay. Hydraulic sorting mechanisms relevant to syn-sedimentary placer formation 1. Entrainment Sorting 2. Shear Sorting 3. Suspension Sorting 4. Transport Sorting (Carling and Breakspear, 2006) Entrainment Sorting *mainly applicable to low turbulence scenarios The ability of a fluid in contact with bed load particles to dislocate certain grains from that bed and move them further downstream. The entrainment threshold of a particle sitting on the bed is determined by: the critical shear stress required to initiate movement of any given particle, influence of bed roughness (sediment transport rates decrease as bed roughness increases) Large grains move faster than smaller ones – smaller grains progress less easily over a rough bed because of trapping and shielding. for any given shear velocity and bed roughness, the largest grains have the fastest transport rates The critical boundary shear stress for entrainment increases as a function of grain density. Shear Sorting The tendency for grains to accumulate in horizons of similar size/density distributions within a “concentrated granular dispersion” (Slingerland, 1984), either due to grain collisions or kinematic sieving (Bagnold, 1954; Middleton, 1965a, 1970) □ Shear sorting of grains is a process that only applies to the concentrated flow of suspended particles in a fluidized bed. □ During the movement of suspended particles in a dense granular dispersion, grain collisions create a net force that is perpendicular to the plane of shearing and disperses the granules toward the free surface (i.e. upwards). □ Counter-intuitively, the dispersive pressure is greater on large and/or dense grains within the same horizon of flow so that these grains migrate upwards relative to smaller and lighter particles. □ e.g. the effect produced by kinetic sieving, where smaller grains simply Suspension sorting The fractionation of grains with different settling velocities into different levels above the bed in a turbulent channel flow system. □ Suspension sorting involves the tendency for lighter, larger grains to stay in suspension for longer (turbulent motion can keep particles suspended for longer). □ As heavy grains can be suspended for shorter distances, this differential causes more proximal deposition, which can then result in the concentration of a placer deposit consisting of the heaviest grains. □ Subsequent to its deposition downstream, sediment sorted in this fashion can result in substantial heavy mineral enrichments. Transport sorting *Most important sorting process for formation of placer deposits □ Differential transport rates exist during movement of particles in a flowing fluid medium. □ Transport sorting corresponds to differences in the unit transport rates for different fractions of the grains present. □ Transport sorting incorporates both entrainment sorting and suspension settling/sorting i.e. Varying rates of movement of grains in suspension (determined by suspension settling). Varying rates of movement of grains both in the bed load (determined by entrainment) Modes of heavy and light mineral sorting over a dune (Carling and Breakspear, 2006) 14 Crossbedding Crossbedding in quartzite Application of sorting principles to placer deposits: small scale Example: heavy minerals along laminae associated with dunes and ripples forming along a stream bed The dune crest is characterized by high shear velocities and non-turbulent flow promotes entrainment of larger, lighter grains and residual concentration of the heavier particles. The dune foreset, on the other hand, is likely to receive heavy mineral concentrations by shear sorting of high concentration grain avalanches down the slope of the advancing dune. The trough or scour forming ahead of the dune is likely to receive heavy grain concentrations by settling sorting in a locally turbulent microenvironment. Rounded Gold grains lying on small-scale cross bed foresets and regarded as evidence of a primary placer origin. Basal Reef, Free State Geduld Mine (W.E.L. Minter, 1990). Source: Tucker et al. 2016 Palaeocurrent direction Rounded Gold grains lying on small-scale cross bed foresets and regarded as evidence of a primary placer origin. Basal Reef, Free State Geduld Mine (W.E.L. Minter, 1990). Source: Tucker et al. 2016 15 Application of sorting principles to placer deposits: intermediate scale Example: in an aggrading point bar forming along the convex bank of a meander channel Deposition in an aggrading point bar forming along the convex bank of a meander channel Dense, heavier minerals, may concentrate where point bars are developed along the convex bank of a meandering river channel. Such sites are viable dredging sites for accumulations of HEAVY MINERALS, such as cassiterite or gold Heavy mineral concentrations actually form in degraded scours along the bottom of the channel itself. Placer processes: Alluvial diamond deposits of the Orange River Map showing the Orange River drainage system in relation to the distribution of deeply eroded kimberlites on the Kalahari Craton. The distribution of alluvial diamond deposits along major river channels and paleo- channels, as well as the beach placers along the west coast of southern Africa (after Lynn et al., 1998). Placer processes: Alluvial diamond deposits of the Orange River Diamonds are trapped in gravel terraces that represent preserved sections of river sediment, abandoned by the present river as it migrates laterally or incises downwards This is a secondary diamond ore deposit. The primary ore deposit is represented by the kimberlite Sediment sorting in beach and eolian environments: heavy mineral sand deposits □ Many important placer deposits are associated with sediments deposited in shoreline environments where sediment sorting is largely controlled by the dynamics of waves and by tidal fluctuations. □ Source fertility is self-evident and dictates whether a placer deposit is likely to contain ilmenite–zircon or diamond concentrations, or none at all. □ Heavy mineral concentrations in some of the enormous beach-related “black sand” (Ti–Zr) placer deposits of the world, such as Richard’s Bay in South Africa, are a likely product of both beach and wind-related sorting processes. Map of Africa, showing the producing heavy minerals deposits heavy minerals mines heavy minerals marginal deposits Rozendaal et al. 2017 Beach Placer Deposits Heavy mineral layers (“black sand”) within quartz beach sand: a) beach and aeolian facies Trivandrum, India Zircon (ZrSiO4), Rutile (TiO2) and Ilmenite (FeTiO3) Zircon (ZrSiO4) Rutile (TiO2) Linda Iaccheri School of Geosciences [email protected] GEOL3051A L21-The Witwatersrand Basin - The World’s Greatest Goldfield The Witwatersrand Basin is the largest goldfield in the world having yielded over 52,000 tonnes of gold (~1,672 Moz.) to the present time, representing more than one third (30%) of all gold ever produced on Earth. □ It is estimated that an inferred resource of about 30,000 tonnes (~965 Moz.) remain in the basin. □ It is also an important source of uranium. □ The Witwatersrand Supergroup is a 2.9 Ga old, Archaean, intracratonic, sedimentary succession, occupying a central position on the Kaapvaal Craton of South Africa. Geological setting of the Witwatersrand Basin in the central portion of the Kaapvaal Craton. □ The Witwatersrand basin covers an elliptical area - 300 km long major axis from the NE to the SW, and is 150 km wide from the SE- NW. □ Seven major goldfields which extend for over 400 km “The Golden Arc”. □ The arc is centred on the conspicuous Vredefort Dome, a major meteorite impact site (2 Ga ago) Seven major goldfields of the “The Golden Arc”: 1. Evander 2. East Rand 2a South Rand 3. Central Rand 4. West Rand 4a West Rand South 5. West Wits 5a. Eastern Section 5b. Carletonville 6. Klerksdorp 7. Welkom Gold Production □ The Witwatersrand was, for over a century, (late 1800s to 2006) the largest producer of gold in the World. □ The Witwatersrand still contains the largest resource of gold in the World, estimated to be in the order of some 30,000 tonnes. □ Gold has been mined down to 4,000 m and the resource has still not bottomed out. □ Major capital is however required to mine at these great depths. A conservative estimate of gold produced from the principal gold fields, on the major reef horizons (Handley, 2004 and Robb & Robb, 1998). History of gold mining in the Wits Basin In 1884, Henry Lewis an Australian digger found gold in the Blaaubank area (Magaliesberg). In 1885, the Nil Desperandum company was formed – the 1st gold-mining Co. in the Wits region Little gold produced – closed end 1887 History of gold mining in the Wits Basin Between 1880 and 1885, Fred and Harry Struben found gold-bearing quartz veins in the Roodepoort area (Kloofendal Nature Reserve) and they mined these veins. In Sept. 1884 Fred discovered the Confidence Reef, very high grades localised in a shear zone, but low recoveries Fred Struben The Strubens’ plant at Wilgespruit, 1883 History of gold In Dec. 1885 George Walker and George Harrison, who had been in the goldfields of Australia, sailed into Durban, and made their way to Barberton. Harrison went to work on farm Langlaate and at weekends panned streams for gold. He identified a conglomerate and panned it Discovered substantial gold, was awarded a discovery claim, which was bought by diamond magnates from Kimberley. Rissik Street Johannesburg around 1890 Ferreira’s camp Been mined for gold since 1886 from conglomerate beds which are generally poorly exposed in outcrop but well documented from over 100 years of mining. 90% of the gold is hosted in conglomerate layers, called ‘reefs’. 1964-1974 was the heydey of production The Witwatersrand Supergroup deposited on top the >3.12 Ga granite-greenstones basement and bimodal rift-related Dominion Group volcanics 3086- 3074 Ma Sedimentation of the Witwatersrand Supergroup occurred in a foreland basin (Wits Basin) during the collision between the Zimbabwe and Kaapvaal cratons ~3.07- 2.71 Ga ago Sedimentary sequence of the Witwatersrand Basin The Witwatersrand Supergroup deposited in the Witwatersrand Basin between 3.0 Ga and 2.7 Ga on an Archean basement of granitoids and Greenstone greenstone belts remnant The Witwatersrand Supergroup of the Witwatersrand Basin The sedimentary sequence is subdivided in: Central Rand Group (2900 m) West Rand Group (4000 m) Greenstone remnant Model showing the main architectural elements of the multistage development of Witwatersrand palaeoplacers (R.F. Tucker, 1980). Eroded Archean Basement Witwatersrand Supergroup - The West Rand Group The West Rand Group consists of shales, quartzites and minor conglomerates Deposited in an epicontinental environment that evolved to a shallow marine shelf Then, compressional tectonics fragmented the basement into a series of discrete blocks resulting in a series of sub-basins within the original basin, into which the Central Rand Group was deposited. Witwatersrand Supergroup - The Central Rand Group The Central Rand Group consists mainly of quartzites and conglomerates. Arenaceous rocks dominate, coarsening upwards important for mineralisation The Central Rand Group comprises several formations which, although varying in thickness, can be traced and correlated, with a few exceptions, in all the goldfields Stratigraphy of the Central Rand Group The gold-bearing conglomerate reefs can be correlated in the seven major goldfields. Regional geological map and section of the Central Rand Group showing the outcrops of the major conglomerate groups (M.J. Viljoen - after E.T. Mellor, 1915). Sedimentology A clear relationship between gold mineralisation and sedimentation has been observed in the Witwatersrand. The most likely source of this detrital gold is detritus derived from the erosion of older, Archaean, lode gold deposits which are present in all the greenstone belts of the Kaapvaal Craton water-cut reef channel containing gold- bearing conglomerate and cross-bedded quartzite in the Middle Elsburg Composite Reef at Randfontein estates gold mine. Quartz pebble conglomerate with white to dark grey quartz pebbles in a pyritic matrix. The dark-rimmed dreikanter pebble near top right attests to aeolian processes on the braid plain (Kimberley Reef) (Photo by C. Pais, Angloval Ltd.). Rounded Gold grains lying on small-scale cross bed foresets and regarded as evidence of a primary placer origin. Basal Reef, Free State Geduld Mine (W.E.L. Minter, 1990) Sedimentology & Pay (Ore) Shoots Sedimentological studies have played an important role in the understanding of gold distribution patterns and the economic evaluation of various reef horizons. Identification of braided fluvial patterns and paleocurrents for locating the accumulation of heavy minerals during sedimentary processes. Conceptual landscape at the time of formation of the Carbon Leader Reef The Pay (ore) shoots consist of the richest part of the ore deposit (reef) Paleo channels where gold and heavy minerals were deposited Pay (Ore) Shoots MRL=Main Reef Leader Main Reef Leader sedimentary channels and related ore shoots largely controlled by NW-SE trending folds and highlighted by a reef isopach plan. Central Rand Goldfield Plan and Section showing the distribution of sedimentological facies in the Carbon Leader Reef, Carletonville Goldfield. (M.J. Viljoen) The determination of palaeocurrent trends and pay shoots patterns has been an important factor in the evaluation and understanding of Witwatersrand reefs. Palaeocurrent trends and pay shoots in the Nigel and Kimberley Reefs of the East Rand Goldfield (After Minter and Loen, 1991) A well-defined west-east pay shoot pattern for the Elsburg Composite Reef on Cooke Section corresponds with fluvial entry points in the vicinity of the Panvlakte fault to the west. Well mineralised highly pyritic composite conglomerate containing buckshot pyrite and large quartz pebbles with individual grades exceeding 100g/t, occurs in the proximal areas of these ore shoots (M.J. Viljoen). Main Reef Group The Main Reef Group (MRG) of conglomerate reefs lies at the base of the Johannesburg Subgroup of the Central Rand Group. □ The Main Reef, Main Reef Leader and South Reef and their equivalents in other goldfields are the most important reefs in this cluster and have been extensively mined along the northern edge of the basin in the East Rand, Central Rand and West Wits Goldfields. □ In the East Rand Goldfield they are collectively reduced to the Nigel Reef while they are not present in the Evander Goldfield. The Main Reef and overlying Main Reef Leader (MRL) were the first reefs to be mined. Outcrop of the Main Reef and Main Reef Leader conglomerates at the Langlaagte Discovery Site on the Central Rand Goldfield (M.J. Viljoen) Main Reef □ The Main Reef is the lowermost of the economically exploitable reefs. □ It is generally a poorly sorted conglomerate with relatively large pebbles (typically up to 5 cm in diameter) and occasional boulder filled channels. □ It is usually composed of a number of pebble bands which coalesce as the reef package thins from over 4 m to less than 2 m as seen on the western and eastern faces, respectively, at the Discovery site on Langlaagte. □ The reef has been sporadically mined along strike, particularly where it is thinner and has higher grades. A substantial resource averaging just less than 4 g/t (using a 3 g/t cut- off) is still present in the Main Reef on the Central Rand. □ The Carbon Leader Reef is the correlative of the Main Reef in the Carletonville Goldfield. It is characterised by an abundance of carbon with visible gold and very high gold grades. Pyrite □ Pyrite is common in the Witwatersrand gold-bearing conglomerates and in certain quartzites and is often enriched in gold. □ Several forms of pyrite are present and attest to a range of formation processes. □ Some of the best gold values in Witwatersrand reefs occur when dull, porous, “buckshot” pyrite is present. Coarse, dull rounded, porous pyrite ("buckshot") on foresets, interpreted as being syngenetic in origin. The gold grade is circa 200 g/t, E9G/d Reef (top of Kimberley Formation) on Cooke Section, Randfontein Estates Gold Mine (Tucker, 1980). Uranium □ Uranium occurs in the conglomerate reefs mainly as the mineral uraninite (UO2) which, due to oxidation, contains a certain proportion of U3O8. □ Uranium is also frequently associated with carbonaceous matter in the reefs (as for example in the Carbon Leader Reef) A water-worn Pyritic pebble with inclusions of uraninite (1), radiogenic galena (2), chromite (3), allogenic pyrite (4), interstitial secondary pyrite (5), chlorite (6) and pyrrhotite (7). The pebble edge is indicated by (8) (R.F. Tucker, 1980). Carbon □ Carbonaceous material is common in the gold bearing Witwatersrand conglomerates. □ It occurs mainly as thin black seams varying from a few millimetres to a few centimetres in width. □ It is particularly common in the Carbon Leader of the Carletonville Goldfield and the Vaal, Basal and Elsburg Reefs. □ As with gold and uranium, there has been considerable debate concerning the origin of the carbon and reasons for its close association with gold in particular Carbon Seam on basal scour of Carbon Leader Reef with columnar carbon structure and abundant visible gold – West Wits Goldfield (Photograph by B. Cairncross). The early Earth atmosphere and the Great Oxidation Event (GOE) The placer origin of the rounded pyrite indicates that before GOE pyrite and uraninite were a stable heavy mineral during erosion and transport (sedimentary processes) in an early Earth, oxygen-poor atmosphere. GREAT OXIDATION EVENT Change in atmosphere’s composition through time Today Is all the gold of the Wits due to sedimentary processes? Gold vein with carbon nodules (black) in a buckshot pyrite matrix. VCR, Carletonville Goldfield (A.A. Lanham) We look now intoTHAT MECHANISMS theHAVE mechanisms that CONTRIBUTED may have TOWARDS contributed THE GOLD towards the goldOF ENRICHMENT enrichment of the Witwatersrand THE WITWATERSRAND BASIN Basin Controversy: how was the Wits gold concentrated? Placer model (syngenetic model) Epigenetic model hydrothermal fluids percolating through the sediments formed an epigenetic gold deposit. Modified placer model Other newer models Placer model gold, pyrite, and other heavy minerals were washed into the Basin as placer heavy minerals, eroded from a fertile source and concentrated by sedimentary processes; Evidence from water-cut channels filled with gold-bearing conglomerate evidence for placer origin Basal Reef gold and pyrite along foresets – Lawrie Minter gold concentrated along fore-sets in coarse sands and conglomerates micro-nuggets of gold, which are typical of detrital deposition. also flattened and rolled gold grains Rh-Os age of gold is older than the sediments and has a mantle signature – 3.01 Ga in Vaal Reef rounded pyrites that are also older than the sediments evidence for placer origin The placer model has dominated the exploration models for predicting the location of gold in the The Vaal Reef, Klerksdorp Wits Basin for the first hundred years of mining. Epigenetic model or hydrothermal model hydrothermal fluids percolating through the sediments preferentially moved through the coarse gravels to form an epigenetic gold deposit. These fluids may have been derived from basin dewatering and/or metamorphism. Schematic representation of the genesis of Witwatersrand gold through metamorphic devolatilization and hydrothermal replacement. In this model, the mafic sequence is shown undergoing regional metamorphism and generating an auriferous fluid (Phillips and Powell, 2014). octahedral gold crystal in Evidence for epigenetic model Quartz vein Skeletal gold can only have formed after the deposition of the Epigenetic (secondary) pyrite sediments from hydrothermal fluids. late appearance of gold in the Skeletal gold paragenetic sequence as microfracture fills and inclusions in sedimentary hydrothermal mineral grains The presence of extensive alteration of host rocks and an overprint of the gold- uraninite mineralization has also been related to the hydrothermal model (Mathur et al, 2013; Phillips and Powell, Woods, 2016 2014). Linda Iaccheri School of Geosciences [email protected] Types of Sedimentary Ore-Forming Processes 1. the accumulation of heavy, detrital minerals and the formation of placer deposits; 2. the precipitation mechanisms that give rise to Fe and Mn concentrations in chemical sediments; 3. the origins of oil and gas deposits, as well as of coalification processes Chemical concentration of metals A chemical sedimentary ore in which concentrations of ore minerals accumul