Principles of Biology - An Introduction to Biological Concepts PDF
Document Details
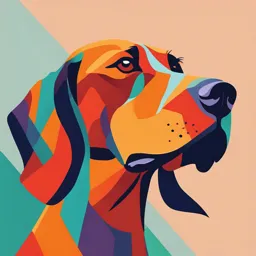
Uploaded by ObservantPipeOrgan442
University of Santo Tomas–Legazpi
Samantha Fowler, Rebecca Roush, James Wise, Mary Ann Clark, Matthew Douglas, Jung Choi, Nina Parker, Mark Schneegurt, Anh-Hue Thi Tu, Philip Lister, Brian M. Forster, J. Gordon Betts, Kelly A. Young,
Tags
Summary
This textbook provides an introduction to biological concepts, drawn from OpenStax textbooks on concepts of biology, biology 2E, microbiology, and anatomy and physiology. It covers a range of topics, including the process of science, the chemistry of life, cell structure and function, cellular respiration and photosynthesis, cell division and genetics, evolution, molecular biology and biotechnology. The textbook is freely accessible online.
Full Transcript
Principles of Biology – An Introduction to Biological Concepts has been modified from several OpenStax textbooks including Concepts of Biology, Biology 2E, Microbiology and Anatomy and Physiology. These textbooks have been cited and attributed below. Each textbook can be accessed for free in its or...
Principles of Biology – An Introduction to Biological Concepts has been modified from several OpenStax textbooks including Concepts of Biology, Biology 2E, Microbiology and Anatomy and Physiology. These textbooks have been cited and attributed below. Each textbook can be accessed for free in its original form by clicking on the links included with each book citation. The OpenStax textbooks are licensed under Creative Commons Attribution License 4.0. Concepts of Biology OpenStax o Authors: Samantha Fowler, Rebecca Roush, James Wise o Publisher/website: OpenStax o Book title: Concepts of Biology o Publication date: Apr 25, 2013 o Location: Houston, Texas o Book URL: https://openstax.org/books/concepts-biology/pages/1-introduction o Section URL: https://openstax.org/books/concepts-biology/pages/1-introduction © Jan 12, 2021 OpenStax. Textbook content produced by OpenStax is licensed under a Creative Commons Attribution License 4.0 license. The OpenStax name, OpenStax logo, OpenStax book covers, OpenStax CNX name, and OpenStax CNX logo are not subject to the Creative Commons license and may not be reproduced without the prior and express written consent of Rice University. Biology 2E OpenStax o Authors: Mary Ann Clark, Matthew Douglas, Jung Choi o Publisher/website: OpenStax o Book title: Biology 2e o Publication date: Mar 28, 2018 o Location: Houston, Texas o Book URL: https://openstax.org/books/biology-2e/pages/1-introduction o Section URL: https://openstax.org/books/biology-2e/pages/1-introduction © Jan 7, 2021 OpenStax. Textbook content produced by OpenStax is licensed under a Creative Commons Attribution License 4.0 license. The OpenStax name, OpenStax logo, OpenStax book covers, OpenStax CNX name, and OpenStax CNX logo are not subject to the Creative Commons license and may not be reproduced without the prior and express written consent of Rice University. Microbiology OpenStax o Authors: Nina Parker, Mark Schneegurt, Anh-Hue Thi Tu, Philip Lister, Brian M. Forster o Publisher/website: OpenStax o Book title: Microbiology o Publication date: Nov 1, 2016 o Location: Houston, Texas o Book URL: https://openstax.org/books/microbiology/pages/1-introduction o Section URL: https://openstax.org/books/microbiology/pages/1-introduction © Aug 20, 2020 OpenStax. Textbook content produced by OpenStax is licensed under a Creative Commons Attribution License 4.0 license. The OpenStax name, OpenStax logo, OpenStax book covers, OpenStax CNX name, and OpenStax CNX logo are not subject to the Creative Commons license and may not be reproduced without the prior and express written consent of Rice University. Anatomy and Physiology OpenStax o Authors: J. Gordon Betts, Kelly A. Young, James A. Wise, Eddie Johnson, Brandon Poe, Dean H. Kruse, Oksana Korol, Jody E. Johnson, Mark Womble, Peter DeSaix o Publisher/website: OpenStax o Book title: Anatomy and Physiology o Publication date: Apr 25, 2013 o Location: Houston, Texas o Book URL: https://openstax.org/books/anatomy-and-physiology/pages/1-introduction o Section URL: https://openstax.org/books/anatomy-and-physiology/pages/1-introduction © Sep 11, 2020 OpenStax. Textbook content produced by OpenStax is licensed under a Creative Commons Attribution License 4.0 license. The OpenStax name, OpenStax logo, OpenStax book covers, OpenStax CNX name, and OpenStax CNX logo are not subject to the Creative Commons license and may not be reproduced without the prior and express written consent of Rice University. ii Return to Table of Contents Principles of Biology – An Introduction to Biological Concepts Table of Contents o Preface to the remixed text, Principles of Biology – An Introduction to Biological Concepts of Biology (pages i-vi) Unit 1. The Cellular Foundation of Life Chapter 1: Introduction to Biology and the Process of Science (pages 1-24) o 1.1 Themes and Concepts of Biology (page 1) o 1.2 The Process of Science (pages 14) Chapter 2: Introduction to the Chemistry of Life (pages 25-61) o 2.1 The Building Blocks of Molecules (page 25) o 2.2 Chemical Bonds (page 38) o 2.3 Water (page 48) o 2.4 pH and Buffers (page 56) Chapter 3 Biologically Important Molecules (pages 63-101) o 3.1 Carbon (page 64) o 3.2 Synthesis and Breakdown of Macromolecules (page 68) o 3.3 Biological Molecules – Carbohydrates (page 72) o 3.4 Biological Molecules – Lipids (page 79) o 3.5 Biological Molecules – Proteins (page 87) o 3.6 Biological Molecules - Nucleic Acids (page 97) Chapter 4: Introduction to Cell Structure and Function (pages 103-144) o 4.1 How Microorganisms Are Studied (page 104) o 4.2 Comparing Prokaryotic and Eukaryotic Cells (page 108) o 4.3 Eukaryotic Cell Components (page 113) o 4.4 Eukaryotic Cell Organelles (page 120) o 4.5 Diversity of cell organelles within the eukaryotes (page 133) Access for free at https://openstax.org/ iii Chapter 5: Structure and Function of the Cell Membrane and an Introduction to Energy (pages 145-191) o 5.1 The Cell Membrane (page 145) o 5.2 Passive Transport (page 152) o 5.3 Active Transport (page 161) o 5.4 Energy and Metabolism (page 166) o 5.5 Law of Thermodynamics (page 171) o 5.6 Types of Energy (page 175) o 5.7 Enzymes (page 184) Chapter 6: Introduction to Cellular Respiration (pages 193-222) o 6.1 Energy in Living Systems (page 193) o 6.2 Glycolysis (page 201) o 6.3 Citric Acid Cycle (page 206) o 6.4 Oxidative phosphorylation (page 210) o 6.5 Fermentation (page 216) o 6.6 Connections to Other Metabolic Pathways (page 220) Chapter 7: Introduction to Photosynthesis (pages 223-246) o 7.1: Overview of Photosynthesis (page 223) o 7.2: The Light-Dependent Reactions of Photosynthesis (page 232) o 7.3: The Calvin Cycle (page 240) iv Return to Table of Contents Unit 2: Cell Division and Genetics Chapter 8: Introduction to Reproduction at the Cellular Level (pages 247-293) o 8.1 The Genome (page 248) o 8.2 The Cell Cycle and Mitosis (page 252) o 8.3 Prokaryotic Cell Division (page 265) o 8.4 Sexual Reproduction (page 269) o 8.5 Meiosis (page 272) o 8.6 Errors in Meiosis (page 287) Chapter 9: Introduction to Patterns of Inheritance (pages 295-333) o 9.1 Gregor Mendel and Genetic Crosses (page 295) o 9.2 Laws of Inheritance (page 301) o 9.3 Extensions of the Laws of Inheritance (page 313) o 9.4 Chromosomal Basis of Inheritance (page 320) o 9.5 Patterns of Inheritance (page 326) Unit 3: Molecular Biology and Biotechnology Chapter 10: DNA Replication and Protein Synthesis (pages 335-376) o 10.1 The Structure of DNA (page 335) o 10.2 DNA Replication (page 343) o 10.3 Transcription (page 355) o 10.4 Translation (page 364) o 10.5 How Genes Are Regulated (pages 372) Unit 4: Evolution and Introduction to Biotechnology Chapter 11: Introduction Evolution (pages 379-405) o 11.1 Discovering How Populations Change (page 378) o 11.2 Mechanisms of Evolution (page 388) o 11.3. Evidence of Evolution (page 396) o 11.4 Common Misconceptions about Evolution (pages 402) Glossary (pages I-XXI) OER Attribution Table (pages XXIII-XXXVI) Access for free at https://openstax.org/ v Preface Principles of Biology – An Introduction to Biological Concepts of Biology About Our Team Principles of Biology – An Introduction to Biological Concepts would not be possible without the work of the following College of Lake County faculty contributing authors and reviewers. Senior Faculty Contributing Author and Reviewer Elizabeth O’Grady, PhD Faculty Contributing Authors and Reviewers Jason Cashmore, MS Marsha Hay, MS Carol Wismer, MS This textbook was put together to increase accessibility and provide a quality textbook at little or no cost to student learners. This work was funded in part by a sabbatical provided by the College of Lake County and support by the Biological and Health Science Division. A special thank you to Mark Coykendall, Biology Department Chair, for his support and encouragement. This textbook would not have been possible without the support and advise of our families. A copy of this book can be found on CLC’s website – Biology OER All written text found in Principles of Biology – An Introduction to Biological Concepts and the OpenStax textbooks are licensed under Creative Commons Attribution License 4.0. The images, photos, graphs, etc. used in this textbook have each been individually attributed. Images, photos, graphs, etc. have different creative commons licenses and have been licensed according to their original authors. An attribution table can be located on the last pages of this textbook. vi Return to Table of Contents Chapter 1: Introduction to Biology and the Process of Science Figure 1.1 This NASA image is a composite of several satellite-based views of Earth. (credit: modification of work by NASA/Concepts of Biology OpenStax) Viewed from space, Earth offers very few clues about the diversity of life forms that can be found there. The first forms of life on Earth are thought to have been microorganisms that existed for billions of years before plants and animals appeared. Mammals, birds, and flowers are all relatively recent additions to the planet, originating 130 to 200 million years ago. Humans have only inhabited this planet for the last 2.5 million years, and only in the last 200,000 years have humans started looking like we do today. 1.1 Themes and Concepts of Biology Biology is the science that studies life. What exactly is life? This may sound like a silly question with an obvious answer; however, it is not always easy to define life. For example, a branch of biology called virology studies viruses. Viruses exhibit some of the characteristics of life but lack others. It turns out that although viruses can attack living organisms, cause diseases, and even reproduce with the help of host cells, they do not meet all the criteria that biologists use to define life. From its earliest beginnings, biology has wrestled with four questions: What are the shared properties that make something “alive”? How do those various living things function? Planet Access for free at https://openstax.org/ 1 earth has a diversity of life forms; how do we organize and classify these different organisms? Finally, how did this diversity arise, and how is it continuing? As new organisms are discovered every day, biologists continue to seek answers to these and other questions. Properties of Life All living organisms share the following key characteristics: order, response to stimuli, reproduction, adaptation, growth and development, homeostasis, and energy processing. These seven characteristics serve to define life. It is essential that students know these different properties of life and be able to explain each. Order Organisms are highly organized and consist of one or more cells. Even very simple, single-celled organisms are remarkably complex. Inside each cell, atoms come together through chemical bonding and form molecules. Molecules come together to form cell components or structures called organelles. Like the toad shown in Figure 1.2, multicellular organisms can consist of millions of cells. Different groups of cells then specialize in performing specific functions. Without order, specialization would not be possible. Figure 1.2 A toad represents a highly organized individual. (credit: "Ivengo(RUS)"/Wikimedia Commons) Response to Stimuli Organisms respond to diverse stimuli. For example, plants can bend toward a source of light or respond to touch (Figure 1.3). Even tiny bacteria can move toward or away from chemicals, a process called chemotaxis. A movement toward a stimulus is considered a positive response, while movement away from a stimulus is regarded as a negative response. Humans also respond to stimuli. For example, when we become warm on a hot sunny day, the body has tiny glands called sudoriferous, or sweat, glands that make and release sweat onto the skin's surface. The heat from the body can be transferred to the sweat, which acts as a cooling mechanism and helps to maintain constant body temperature. Figure 1.3 The leaves of this sensitive plant (Mimosa pudica) will instantly droop and fold when touched. After a few minutes, the plant returns to its normal state. (credit: Alex Lomas/Concepts of Biology OpenStax) 2 Return to Table of Contents CONCEPTS IN ACTION- Watch this video to see how the sensitive plant responds to a touch stimulus. Reproduction Reproduction is necessary on both a cellular and organismal level. For a population to survive, some individuals within that population must reproduce. Organisms that are multicellular, such as plants and animals, also need to reproduce on a cellular level. As old cells become damaged or worn out, they must be replaced by new cells. For example, skin cells are damaged continuously and need to be replaced every two to three weeks; otherwise, the skin would lose its ability to provide protection. Single-celled organisms must also reproduce. Reproduction begins by first duplicating their genetic material. Once the genetic material is duplicated, it is then divided equally into two new cells (Figure 1.4). The two new daughter cells should be identical to the parent cell. Figure 1.4 Bacteria cell going through division. (credit: Pradana Aumars/Wikimedia Commons) Adaptation All living organisms exhibit a “fit” to their environment. Biologists refer to this fit as adaptation. Adaptations are a consequence of evolution by natural selection. Evolution has had some impact on every lineage of reproducing organisms. Examples of adaptations are diverse and unique. For example, some microorganisms live in boiling hot springs, whereas some moths have tongues the exact length of the flower from which they feed. Adaptations are vital because they enhance an individual's ability to survive and reproduce; however, adaptations are not constant. As an environment changes, natural selection causes the individuals in a population to adapt to those changes. For example, imagine that there is a population of finches living on an island. An environmental event has resulted in two main food sources: soft insects and hard seeds. Not all finches within the population have the same beak length or size. Finches in the population that have long, skinny beaks begin to feed on soft insects because they are easy for those birds to catch and eat. Finches with large, more dense beaks feed on hard seeds because their dense beaks allow them to Access for free at https://openstax.org/ 3 crush and open the hard seeds. Unfortunately, finches that have beaks that are neither long nor dense may slowly begin to decline in number because they are limited in their ability to obtain nutrients. Finches that obtain food can put energy into reproduction and survival needs. When those finches reproduce, they pass along those adaptations that allow them to be successful in their respective feeding environments. Over time two distinct groups may arise, those with thick, dense beaks and those with longer skinnier beaks (Figure 1.5). If these individuals genetically change in such a way that they no longer can interbreed with one another, a speciation event will have occurred. Figure 1.5 Different finch phenotype variations due to environmental changes. (credit: National Human Genome Research Institute's Talking Glossary/Wikimedia Commons) Growth and Development Development is often described as the processes that an individual goes through as it grows and matures. For example, in humans, development begins once the sperm fertilizes the egg. Human development can be broken down into different stages including embryonic development, fetal development, infancy, childhood, puberty, and adulthood. Development can also be observed in many other organisms. For example, butterflies go through a developmental process called metamorphosis that begins at the egg stage and then proceeds to the larva, pupa, and adult stages. Both multicellular and single-celled organisms grow and develop according to specific instructions encoded in their DNA. DNA is organized into genes that provide information for cellular growth and development. An individual's DNA ensures that a species’ young (Figure 1.6) will grow up to exhibit many of the same traits as its parents. Figure 1.6 Although no two looks alike, these kittens have inherited genes from both parents and share many of the same characteristics. (credit: Pieter & Renée Lanser/ Concepts of Biology OpenStax) 4 Return to Table of Contents Homeostasis Even the smallest organisms are complex and require multiple regulatory mechanisms to coordinate internal functions, such as the transport of nutrients (Figure 1.7), response to stimuli, and coping with environmental stresses. Homeostasis or “steady state” is the ability of an organism to regulate and maintain constant internal conditions. Figure 1.7 Human circulatory system plays an important role in transporting oxygen, removal of waste, and delivering nutrients to every cell. (credit: Public domain/Wikimedia Commons) Cells require appropriate conditions such as proper temperature, pH, and concentrations of nutrients to function correctly. Although these conditions may change, organisms can maintain internal conditions within a narrow range. For example, many organisms regulate their body temperature in a process known as thermoregulation. Organisms that live in cold climates, such as the polar bear (Figure 1.8), have body structures such as thick layers of fur or fat, which help them withstand low temperatures and conserve body heat. In hot climates, plants carry out unique versions of photosynthesis to reduce water loss and optimize their potential of making sugar. a b Figure 1.8a. Polar bears and other mammals living in ice-covered regions keep their body temperature relatively constant, even though the environment can be very hot during the day and cold at night. (credit: "longhorndave"/Flickr) b. Polar bear maintain their body temperature by generating heat and reducing heat loss through thick fur and a dense layer of fat under their skin. In this infrared image the polar bear‘s body heat hardly registers; only the uninsulated eyes and mouth show temperatures significantly warmer than the environment. (credit: Arno/Coen/Wikimedia Commons) Access for free at https://openstax.org/ 5 Organisms like humans (Figure 1.9), use their skeletal muscles to generate heat. The contraction of skeletal muscles helps humans maintain stable internal body temperature as environmental conditions fluctuate. If body temperature drops below a certain point, metabolism begins to slow and may even stop, leading to death. Conversely, if body temperature rises above a certain point, it can lead to the destruction of key molecules called proteins. Students that continue and take Anatomy and Physiology classes will spend time discussing how the body works to maintain homeostasis. Students will also look at what occurs when the body loses its ability to maintain stable internal conditions, otherwise referred to as a homeostatic imbalance. Figure 1.9 Thermogram of a snake wrapped around a human arm. (credit: Arno/Coen/Wikimedia Commons) Energy Processing All organisms, including the California condor shown in Figure 1.10, use a source of energy for their metabolic activities. Some organisms can obtain energy through metabolic pathways such as photosynthesis. Photosynthesis is a process where light energy can be captured and converted into chemical energy. Organisms that are capable of making their own chemical energy are referred to as autotrophs. Others must obtain their chemical energy by consuming other organisms. These individuals are referred to as heterotrophs. Regardless of whether an organism is an autotroph or a heterotroph, all living cells must have energy to drive metabolism. Figure 1.10 A lot of energy is required for a California condor to fly. (credit: Pacific Southwest Region U.S. Fish and Wildlife/ Concepts of Biology OpenStax) 6 Return to Table of Contents Levels of Organization of Living Things Living things are highly organized and structured. The atom is the smallest and most fundamental unit of matter. It consists of a nucleus surrounded by electrons. Atoms form molecules. A molecule is a chemical structure consisting of at least two atoms held together by a chemical bond. Many biologically important molecules are macromolecules. A macromolecule is a large molecule that is typically formed by combining smaller molecules. For example, nucleotides are small molecules linked together to form the macromolecule, DNA (deoxyribonucleic acid) (Figure 1.11). DNA contains the instructions necessary for cells and organisms to maintain homeostasis. Figure 1.11 A molecule, like this large DNA molecule, is composed of atoms. (credit: "Brian0918"/Wikimedia Commons) CONCEPTS IN ACTION- To see an animation of this DNA molecule, click here. Some cells contain collections of macromolecules surrounded by membranes; these are called organelles. Organelles are small structures that exist within cells and perform specialized functions. For example, in some cells, DNA is enclosed within a membrane-bound organelle called the nucleus (plural: nuclei). All living things are made of cells; the cell is the smallest fundamental unit found in living organisms. Cells exhibit all of the properties of life discussed above. Viruses are often not considered living because they are not made of cells, nor are they capable of reproducing on their own. To make new viruses, they must invade and take over a living cell. Some organisms consist of a single cell, while others are multicellular. In most multicellular organisms, cells combine to make tissues, which are groups of similar cells carrying out the same function. Organs are collections of tissues grouped based on a common function. Organs are present not only in animals but also in plants. An organ system is a higher level of organization that consists of functionally related organs. For example, vertebrate animals have many organ systems, such as the circulatory system that transports blood throughout the body; it includes organs such as the heart and blood vessels. Organisms are individual living entities. For example, each tree in a forest is an organism. Single-celled prokaryotes and single-celled eukaryotes are also considered organisms and are typically referred to as microorganisms. Access for free at https://openstax.org/ 7 Figure 1.12 From an atom to the entire Earth, biology examines all aspects of life. (credit "molecule": modification of work by Jane Whitney; credit "organelles": modification of work by Louisa Howard; credit "cells": modification of work by Bruce Wetzel, Harry Schaefer, National Cancer Institute; credit "tissue": modification of work by "Kilbad"/Wikimedia Commons; credit "organs": modification of work by Mariana Ruiz Villareal, Joaquim Alves Gaspar; credit "organisms": modification of work by Peter Dutton; credit "ecosystem": modification of work by "gigi4791"/Flickr; credit "biosphere": modification of work by NASA/ Concepts of Biology OpenStax) 8 Return to Table of Contents All the individuals living within a specific area are collectively called a population. For example, a forest may include many white pine trees. All these pine trees represent the population of white pine trees in this forest. Different populations may live in the same area. The forest with the pine trees includes populations of flowering plants, insects, and microbial populations. A community is the set of populations inhabiting a particular area. For instance, all the trees, flowers, insects, and other populations in a forest form the forest’s community. The forest itself is an ecosystem. An ecosystem consists of all the living things in a particular area together with the abiotic, or non-living, parts of that environment, such as nitrogen in the soil or rainwater. At the highest level of organization (Figure 1.12), the biosphere is the collection of all ecosystems on planet Earth. It includes land, water, and portions of the atmosphere. Answer: (b) The Diversity of Life The science of biology is very broad because there is a tremendous diversity of life on Earth. The source of this diversity is evolution. Evolution is the process of genetic change in a population. Evolution helps explain how new species can arise from older species. Speciation events can occur when individuals within a population are separated and begin to change or evolve independently of one another. If the individuals change to the point where they can no longer interbreed, a speciation event has occurred, and species diversity has increased. Evolution will be discussed in much greater detail in chapter 11. In the 18th century, a Swedish scientist named Carl Linnaeus first proposed organizing living organisms into a hierarchical taxonomy. In this system, species that are most similar to each other are put together within a grouping known as a genus. Furthermore, similar genera (the plural of genus) are put together within a family. This grouping continues until all organisms are collected together into groups at the highest level. The current taxonomic system now has eight levels in its hierarchy, from lowest to highest, they are species, genus, family, order, class, phylum, kingdom, and domain (Figure 1.13). Access for free at https://openstax.org/ 9 Figure 1.13 This diagram shows the levels of taxonomic hierarchy for a dog, from the broadest category—domain—to the most specific—species. (credit: Fowler et al./Concepts of Biology OpenStax) The highest taxonomy level, domain, is a relatively new addition (1990's) to the system. Scientists now recognize three domains of life: the Eukarya, the Archaea, and the Bacteria. The domain Eukarya is very diverse and includes the kingdoms of fungi, plants, animals, and several kingdoms of protists. Humans, plants, yeast, and mushrooms are just a few representatives of the domain Eukarya. These organisms are classified as eukaryotes because they have nuclei and other membrane-bound organelles. Both the Archaea and Bacteria are single-celled organisms classified as prokaryotes (Figure 1.14). Prokaryotes are organisms that lack nuclei and other membrane-bound organelles. Prokaryotes, like eukaryotes, are very diverse and can be subdivided into phyla, class, order, etc. Figure 1.14 These images represent different domains. The scanning electron micrograph shows (a) bacterial cells belong to the domain Bacteria, while the (b) extremophiles, seen all together as colored mats in this hot spring, belong to domain Archaea. Both the (c) sunflower and (d) lion are part of the domain Eukarya. (credit a: modification of work by Rocky Mountain Laboratories, NIAID, NIH; credit b: modification of work by Steve Jurvetson; credit c: modification of work by Michael Arrighi; credit d: modification of work by Frank Vassen / Concepts of Biology OpenStax) 10 Return to Table of Contents Evolution in Action - Carl Woese and the Phylogenetic Tree The evolutionary relationships of various life forms on Earth can be summarized in a phylogenetic tree. A phylogenetic tree is a diagram showing the evolutionary relationships among biological species based on similarities and differences in genetic or physical traits or both. The pioneering research of American microbiologist Carl Woese at the University of Illinois has shown that life on Earth has evolved along three lineages, now called domains. The phylogenic tree in Figure 1.15 can be used to show the separation of living organisms into those three domains: Bacteria, Archaea, and Eukarya. Figure 1.15 This phylogenetic tree was constructed by microbiologist Carl Woese using genetic relationships. (credit: modification of work by Eric Gaba/ Concepts of Biology OpenStax) Branches of Biological Study The scope of biology is broad and therefore contains many branches and sub-disciplines. For instance, molecular biology studies biological processes at the molecular level, including interactions among molecules such as DNA, RNA, and proteins. Microbiology is the study of the structure and function of microorganisms. It is quite a broad branch itself, and depending on the subject of study, there are also microbial physiologists, ecologists, and geneticists, among others. Paleontology, another branch of biology, uses fossils to study life’s history (Figure 1.16). Zoology and botany are the study of animals and plants, respectively. Biologists can also specialize as biotechnologists, ecologists, or physiologists, to name just a few areas. Access for free at https://openstax.org/ 11 Biotechnologists apply the knowledge of biology to create useful products. Ecologists study the interactions of organisms in their environments. Physiologists study the workings of cells, tissues, and organs. This is just a small sample of the many fields that exist within biology. Figure 1.16 Researchers work on excavating dinosaur fossils at a site in Castellón, Spain. (credit: Mario Modesto/ Concepts of Biology OpenStax) CAREER CONNECTION - Forensic Scientist Forensic science is the application of science to answer questions related to the law. Biologists, as well as chemists and biochemists, can be forensic scientists. Forensic scientists provide scientific evidence for use in courts, and their job involves examining trace materials associated with crimes. Interest in forensic science has increased in the last few years, possibly because of popular television shows that feature forensic scientists on the job. The development of molecular techniques and the establishment of DNA databases have updated the types of work that forensic scientists can do. Their job activities are primarily related to crimes against people, such as murder, rape, and assault. Their work involves analyzing samples such as hair, blood, and other body fluids, and processing DNA (Figure 1.17a) found in many different environments and materials. Forensic scientists also analyze biological evidence left at crime scenes, such as insect parts or pollen grains (Figure 1.17b). Students who want to pursue careers in forensic science will most likely be required to take chemistry and biology courses as well as some intensive math courses. Figure 1.17a This forensic scientist works in a DNA extraction lab. (credit: U.S. Army CID Command Public Affairs/ Concepts of Biology OpenStax) b. This scientist uses microscopy for sample analysis. (credit: National Cancer Institute /Public Domain) 12 Return to Table of Contents Section Summary Biology is the science of life. All living organisms share several key properties such as order, response to stimuli, reproduction, adaptation, growth and development, homeostasis, and energy processing. Living things are highly organized following a hierarchy that includes atoms, molecules, organelles, cells, tissues, organs, and organ systems. Organisms are grouped as populations, communities, ecosystems, and the biosphere. Evolution is the source of the tremendous biological diversity on Earth today. A diagram called a phylogenetic tree can be used to show evolutionary relationships among organisms. Biology is very broad and includes many branches and sub-disciplines. Examples include molecular biology, microbiology, neurobiology, and ecology, among others. Exercises 1. Which of the following statements is false? a. Tissues exist within organs which exist within organ systems. b. Communities exist within populations which exist within ecosystems. c. Organelles exist within cells which exist within tissues. d. Communities exist within ecosystems which exist in the biosphere. 2. The smallest unit of biological structure that meets the functional requirements of “living” is the ________. a. organ b. organelle c. cell d. macromolecule 3. Which of the following sequences represents the hierarchy of biological organization from the most complex to the least complex level? a. organelle, tissue, biosphere, ecosystem, population b. organ, organism, tissue, organelle, molecule c. organism, community, biosphere, molecule, tissue, organ d. biosphere, ecosystem, community, population, organism 4. Briefly explain how evolution is a source of species diversity. Answers 1. (b) 2. (c) 3. (d) 4. Evolution leads to genetic changes in a population. For example, if you had a population of insects that live on a maple tree, some insects may begin to feed selectively on the bark of the tree, while others may selectively feed on the leaves. Over time, genetic changes can occur that may prevent these two groups from breeding with one another. In this case, a speciation event has occurred, increasing species diversity. Access for free at https://openstax.org/ 13 Glossary atom: a basic unit of matter that cannot be broken down by normal chemical reactions autotroph: an organism that can make its own food from materials in its environment biology: the study of living organisms and their interactions with one another and their environments biosphere: a collection of all ecosystems on Earth cell: the smallest fundamental unit of structure and function in living things community: a set of populations inhabiting a particular area domain: the highest level of the taxonomic hierarchy; includes the Eukarya, Archaea, and Bacteria ecosystem: all living things in a particular area together with the abiotic, nonliving parts of that environment eukaryote: an organism with cells that have nuclei and membrane-bound organelles evolution: the process of gradual change in a population that can also lead to new species arising from older species heterotroph: an organism that cannot make its own food and must consume other organisms to obtain its energy homeostasis: the ability of an organism to maintain constant internal conditions macromolecule: a large molecule typically formed by the joining of smaller molecules molecule: a chemical structure consisting of at least two atoms held together by a chemical bond organ: a structure formed of tissues operating together to perform a common function organ system: the higher level of organization that consists of functionally related organs organelle: a membrane-bound compartment or sac within a cell organism: an individual living entity phylogenetic tree: a diagram showing the evolutionary relationships among biological species based on similarities and differences in genetic or physical traits or both population: all individuals within a species living within a specific area prokaryote: a unicellular organism that lacks a nucleus or any other membrane-bound organelle tissue: a group of similar cells carrying out the same function 14 Return to Table of Contents 1.2 The Process of Science Figure 1.18 (a) cyanobacteria seen through a light microscope are some of Earth’s oldest life forms (b) stromatolites along the shores of Lake Thetis in Western Australia are ancient structures formed by the layering of cyanobacteria in shallow waters. (credit a: modification of work by NASA; scale-bar data from Matt Russell; credit b: modification of work by Ruth Ellison / Concepts of Biology OpenStax) Biology is a science that gathers knowledge about the natural world (Figure 1.18). Specifically, biology is the study of life. Biological discoveries are made by a community of researchers who work both individually and together using agreed-on methods. The methods of science include careful observation, record keeping, logical and mathematical reasoning, experimentation, and submitting conclusions to the scrutiny of others. Science also requires considerable imagination and creativity; a well-designed experiment is commonly described as elegant or beautiful. Science has significant practical implications and applications, for example, in the prevention of disease (Figure 1.19). Other types of science are motivated by curiosity. Whatever its goal, there is no doubt that science, including biology, has transformed human existence and will continue to do so. Access for free at https://openstax.org/ 15 Figure 1.19 In this micrograph, the bacterium is visualized using a scanning electron microscope and digital colorization. (credit: Eric Erbe; digital colorization by Christopher Pooley, USDA-ARS / Concepts of Biology OpenStax) The Nature of Science Science can be defined as knowledge about the natural world. It is a precise way of learning about the world and is largely responsible for the technological revolutions that have taken place. There are, however, areas of knowledge and human experience that the methods of science cannot be applied to. These include such things as answering moral questions, aesthetic questions, or spiritual questions. Science cannot investigate these areas because they are outside the realm of natural phenomena and cannot be observed and measured. The scientific method is a method of research with defined steps that includes careful observation and experiments. The steps of the scientific method will be examined in greater detail later, but one of the most important aspects of the scientific method is the testing of hypotheses. A hypothesis (plural hypotheses) is a suggested explanation for a scientific question or an observation, which can be tested. A good hypothesis should be clear and concise. It should also lead to predictions, which are statements that describe what should happen if the hypothesis is correct and supported. A hypothesis should also be falsifiable, meaning the hypothesis can be incorrect if data that is collected refutes the hypothesis. An example of a hypothesis that is not falsifiable is, “Chicago is the most beautiful city in the world.” There is no experiment that might show this statement is false. Once a hypothesis has undergone rigorous testing, and large amounts of data have been collected by multiple research groups who have drawn the same or similar conclusions, the hypothesis is referred to as a theory. In science, a theory is a confirmed explanation for a set of observations or phenomena that has been thoroughly tested and supported with substantial amounts of data. In this way, it is very different than a hypothesis. However, like hypotheses, theories are testable, falsifiable, and lead to predictions. A scientific theory is the foundation of scientific knowledge. Also, in many scientific disciplines (less so in biology), there are scientific laws, often expressed in mathematical formulas. Scientific laws describe how elements of nature will behave under certain specific conditions. There is not a strict process that a hypothesis must go through to become a theory or a law. Hypotheses are the day-to-day material that scientists work with, and they are developed within the context of theories. Laws are concise descriptions of parts of the world that are amenable to formulaic or mathematical description. 16 Return to Table of Contents Natural Sciences Those fields of science related to the physical world and its phenomena and processes are considered natural sciences. There is no complete agreement when it comes to defining what the natural sciences include (Figure 1.20). For some experts, the natural sciences are astronomy, biology, chemistry, earth science, and physics. Other scholars choose to divide natural sciences into life sciences and physical sciences. Life sciences study living things and include biology. Physical sciences study nonliving matter and include astronomy, physics, and chemistry. Some disciplines, such as biophysics and biochemistry, build on two sciences, and are interdisciplinary. Figure 1.20 Some fields of science include astronomy, biology, computer science, geology, logic, physics, chemistry, and mathematics. (credit: "Image Editor" Flickr / Concepts of Biology OpenStax) Scientific Inquiry One thing is common to all forms of science: the ultimate goal is to obtain knowledge. Curiosity and inquiry are the driving forces for the development of science. Scientists seek to understand the world and the way it operates. Two methods of logical thinking are used: inductive reasoning and deductive reasoning. Inductive reasoning is a form of logical thinking that uses related observations to arrive at a general conclusion. This type of reasoning is common in descriptive science. A life scientist such as a biologist makes observations and records them. These data can be qualitative, which is descriptive or categorical, or they can be quantitative, consisting of numbers. From many observations, the scientist can infer conclusions, inductions, based on evidence. Inductive reasoning involves formulating generalizations inferred from careful observation and the analysis of a large amount of data. Brain studies often work this way. Many brains are observed while people are doing a task. The part of the brain that lights up, indicating activity, is then demonstrated to be the part controlling the response to that task. Access for free at https://openstax.org/ 17 Deductive reasoning or deduction is the type of logic used in hypothesis-based science. In deductive reasoning, the pattern of thinking moves in the opposite direction as compared to inductive reasoning. Deductive reasoning is a form of logical thinking that uses a general principle or law to predict specific results. From those general principles, a scientist can extrapolate and predict the specific results that would be valid so long as the general principles are valid. For example, a prediction would be that if the climate is becoming warmer in a region, the distribution of plants and animals should change. Comparisons have been made between distributions in the past and the present, and the many changes that have been found are consistent with a warming climate. Finding the change in distribution is evidence that the climate change conclusion is valid. Both types of logical thinking are related to the two main pathways of scientific study: descriptive science and hypothesis-based science. Descriptive or discovery science aims to observe, explore, and discover. Hypothesis-based science begins with a specific question or problem and a potential answer or solution that can be tested. The boundary between these two forms of study is often blurred because most scientific endeavors combine both approaches. Observations lead to questions, questions lead to forming a hypothesis as a possible answer to those questions, and then the hypothesis is tested. Thus, descriptive science and hypothesis-based science are in continuous dialogue. Hypothesis Testing Biologists study the living world by posing questions about it and seeking science-based responses. This approach is common to other sciences as well and is often referred to as the scientific method. The scientific method typically starts with an observation that leads to a question. Observations can be made using any or all of an individual's general senses such as touch and/or their special senses such as vision. (Students planning to take Anatomy and Physiology will learn more about your different senses.) Let’s think about a scenario that starts with an observation and apply the scientific method to address the observation. One Monday morning, a student arrives in class and quickly discovers that the classroom is too warm. That is an observation that also describes a problem: the classroom is too warm. The student then asks a question: “Why is the classroom so arm?” Recall that a hypothesis is a testable explanation to the question. Several hypotheses may be proposed. For example, one hypothesis might be, “The classroom is warm because no one turned on the air conditioning.” But there could be other responses to the question, and therefore other hypotheses may be proposed. A second hypothesis might be, “The classroom is warm because there is a power failure, and so the air conditioning doesn’t work.” Once a hypothesis has been formulated, a prediction can be made. A prediction is similar to a hypothesis, but it typically has the format “If... then....” For example, the prediction for the first hypothesis might be, “If the student turns on the air conditioning, then the classroom will no longer be too warm.” 18 Return to Table of Contents A hypothesis must be testable to ensure that it is valid. For example, a hypothesis that depends on what a bear thinks is not testable, because it can never be known what a bear thinks. To test a hypothesis, a researcher will conduct one or more experiments designed to eliminate one or more of the hypotheses. This is important. A hypothesis can be shown to be false or eliminated, but it can never be proven true. Science does not deal with proof, like mathematics. If an experiment supports the hypothesis, this is not to say that down the road, a better explanation will not be found, which is why the word "prove" is not used when a hypothesis is supported. Each experiment will have variables, controls, and experimental groups. A variable is any part of the experiment that can vary or change during the experiment. There are typically three kinds of variables: independent, dependent, and standardized. The independent variable is the variable that is being altered or changed by the researcher. It is the variable whose effect is being tested. The dependent variable is the variable that may change when the independent variable is applied. This is what the researcher will observe, measure, and record during the experiment. The standardized variables are variables that must be kept consistent among all test groups; otherwise, they can affect the outcome or results of the experiment. The experimental groups in an experiment receive varying types or amounts of the independent variable. A control group is usually included as a basis of comparison for the experimental groups. For the control group the independent variable is absent or set to some predetermined standard. Look for the variables, controls, and experimental group(s) in the following example. An experiment is conducted to test the hypothesis that phosphate availability limits the growth of algae in freshwater ponds. A series of artificial ponds are filled with water, and half of them are treated by adding phosphate each week, while the other half is treated by adding salt. Salt is a known substance that is not used by algae. The independent variable here is the phosphate. The experimental groups are the ponds to which phosphate was added, and the control group is the ponds to which the salt was added. Adding the salt is a control against the possibility that adding extra matter to the pond influences algae growth. Some factors must be standardized in both the control and experimental ponds. For example, both the temperature and pH of the water should be standardized. If the water in the control ponds has a significantly higher temperature or pH compared to the water used in the experimental ponds, this could influence the growth of algae. These factors need to be measured and kept relatively constant between the two groups. These are examples of standardized variables. If the ponds treated with phosphate show more algae growth than the control ponds, then we have found support for our hypothesis. If they do not, then we reject our hypothesis. Be aware that rejecting a hypothesis does not determine whether or not the other hypotheses can be accepted; it simply eliminates one hypothesis that is not valid (Figure 1.21). Access for free at https://openstax.org/ 19 Figure 1.21 The scientific method is a series of defined steps that include experiments and careful observation. (credit: Fowler et al. / Concepts of Biology OpenStax) In the example below, the scientific method is used to solve an everyday problem. Answers: (3) hypothesis; (4) prediction; The hypothesis would be rejected. Hypothesis 2: The toaster has a loose wire and is broken. 20 Return to Table of Contents The scientific method is not as rigid and structured as it might first appear. Sometimes an experiment leads to conclusions that favor a change in approach. Often, experiments bring about entirely new scientific questions. Many times, science does not operate linearly; instead, scientists continually draw inferences and make generalizations, finding patterns as their research proceeds. Scientific reasoning is more complex than the scientific method alone suggests. Basic and Applied Science The scientific community has been debating for the last few decades about the value of different types of science. Is it valuable to pursue science for the sake of simply gaining knowledge, or does scientific knowledge only have worth if we can apply it to solving a specific problem or bettering our lives? This question focuses on the differences between two types of science: basic science and applied science. Basic science or “pure” science seeks to expand knowledge regardless of the short-term application of that knowledge. It is not focused on developing a product or a service of immediate public or commercial value. The immediate goal of basic science is knowledge for knowledge’s sake, though this does not mean that in the end, it may not result in an application. In contrast, applied science or “technology,” aims to use science to solve real-world problems, making it possible, for example, to improve crop yield, find a cure for a particular disease, or save animals threatened by a natural disaster. In applied science, the problem is usually defined by the researcher. One example of how basic and applied science can work together occurred with the discovery of the DNA structure. This discovery then led to the understanding of the molecular mechanisms that control DNA replication. Every human has unique chromosomes, strands of DNA wrapped around proteins, found in their cells. DNA provides the instructions necessary for life. During cell division, new copies of DNA must be made before a cell divides to form two new cells (Figure 1.22). Understanding the mechanisms of DNA replication enabled scientists to develop laboratory techniques that are now used to identify genetic diseases, pinpoint individuals who were at a crime scene, and determine paternity. Without basic science, it is unlikely that applied science would exist. Figure 1.22 Shows DNA replication and cell division by the process of mitosis. Note: diploid means each cell has pairs of chromosomes. (credit: Mysid / Wikimedia Commons) Access for free at https://openstax.org/ 21 Reporting Scientific Work Whether scientific research is basic science or applied science, scientists must share their findings for other researchers to expand and build upon their discoveries. Communication and collaboration within and between sub-disciplines of science are key to the advancement of knowledge in science. For this reason, an essential aspect of a scientist’s work is disseminating results and communicating with peers. Scientists can share results by presenting them at a scientific meeting or conference, but this approach can reach only a few individuals who are present. Instead, most scientists present their results in peer-reviewed articles that are published in scientific journals. Peer-reviewed articles are scientific papers that are reviewed, usually anonymously, by a scientist’s colleagues, or peers. These colleagues are qualified individuals, often experts in the same research area, who judge whether the scientist’s work is suitable for publication. The process of peer review helps to ensure that the research described in a scientific paper is original, significant, logical, and thorough. There are many journals and the popular press that do not use a peer-review system. Results of any studies published in non-peer reviewed forums are not always reliable, and caution should be used when examining the validity of the work. Sometimes information can be portrayed as scientific fact but lack objective, repeatable data. Pseudoscience is claims or beliefs that are represented as scientific fact but cannot be evaluated using the scientific method. For example, astrology is based on a set of beliefs that connect an individual's personality traits with their astrological sign. Scientists using the scientific method have not been able to generate any data that supports these claims and connections. As a result, astrology can be used as an example of pseudoscience. Today, data and information are readily accessible online through the internet. The internet offers a unique platform to share information across the world, which can help advance both scientific discovery and knowledge. However, it is always important to consider when looking at information online, where the data is coming from, and how valid this information is. 22 Return to Table of Contents Section Summary Biology is the science that studies living organisms and their interactions with one another and their environments. Science attempts to describe and understand the nature of the universe in whole or in part. Science has many fields; those fields related to the physical world and its phenomena are considered natural sciences. A hypothesis is a tentative, testable explanation for an observation or question. A scientific theory is a well-tested and consistently verified explanation for a set of observations or phenomena that has been universally accepted by the scientific community. A scientific law is a description, often in the form of a mathematical formula. Two types of logical reasoning are used in science. Inductive reasoning uses results to produce general scientific principles. Deductive reasoning is a form of logical thinking that predicts results by applying general principles. The common thread throughout scientific research is the use of the scientific method. Scientists present their results in peer-reviewed scientific papers published in scientific journals. Science can be basic or applied. The main goal of basic science is to expand knowledge without any expectation of short-term practical application of that knowledge. The primary goal of applied research, however, is to solve practical problems. Exercises 1. In the example below, the scientific method is used to solve an everyday problem. Which part of the example below is the hypothesis? Which is the prediction? Based on the results of the experiment, is the hypothesis supported? If it is not supported, propose some alternative hypotheses. Jose notices that all the trees in his backyard are dying. They are having a usual dry summer with very little rainfall. His mom also applied fertilizer to the lawn in the early spring. Jose is curious, "why are the trees are all dying?" Jose thinks that because there has been very little rainfall that explains why the trees are dying. If he waters the trees, then they should begin to grow and stop dying. After watering the trees every day for two months, Jose notices that the trees still seem to be dying. 2. ________claims or beliefs that are portrayed as scientific fact but cannot be evaluated using the scientific method. a. Hypothesis b. Variable c. Pseudoscience d. Theory 3. The type of logical thinking that uses related observations to arrive at a general conclusion is called ________. a. deductive reasoning b. the scientific method c. hypothesis-based science d. inductive reasoning 4. Explain the difference between a hypothesis and a theory. Access for free at https://openstax.org/ 23 Answers 1. The hypothesis is the trees are dying because of the lack of water, and the prediction is if he waters the trees, then they should stop dying. The original hypothesis is not supported, because although he waters the trees, they continue to die. Alternative hypotheses maybe because his mom added fertilizer to the lawn; the trees are dying. 2. (c) 3. (d) 4. A hypothesis is a testable explanation for a scientific question or an observation, which should be both falsifiable and lead to predictions. Once a hypothesis has undergone rigorous testing by many different scientific groups who have drawn the same or similar conclusions, it is referred to as a scientific theory. A scientific theory, therefore, is also testable, leads to predictions, and is falsifiable; however, it has been thoroughly tested and supported with substantial amounts of data. A scientific theory is the foundation of scientific knowledge. Glossary biology: the study of life control: a part of an experiment that does not change during the experiment deductive reasoning: a form of logical thinking that uses a general statement to forecast results dependent variable: the variable that will change when the independent variable is altered; this is what the researcher will measure or observe during the experiment experimental group: the group where the independent variable is applied falsifiable: it can be shown to be false by experimental results hypothesis: a suggested explanation for an event, which can be tested independent variable: is the variable that is being altered or changed by the researcher; it is the variable being tested inductive reasoning: a form of logical thinking that uses related observations to arrive at a general conclusion peer-reviewed article: a scientific report that is reviewed by a scientist’s colleagues before publication predictions: statements that describe what should happen if the hypothesis is supported pseudoscience: claims or beliefs that are portrayed as scientific fact but cannot be evaluated using the scientific method qualitative data: data that is descriptive quantitative data: data that is numerical science: the knowledge that covers general truths or the operation of general laws, mainly when acquired and tested by the scientific method scientific method: a method of research with defined steps that include experiments and careful observation scientific theory: a thoroughly tested and confirmed explanation for observations or phenomena standardized variable: variables that must be kept consistent otherwise they can affect the outcome or results of the experiment 24 Return to Table of Contents Chapter 2: Introduction to the Chemistry of Life Figure 2.1 Atoms are the building blocks that come together through chemical bonding to form molecules in the universe. In this model of a molecule, the atoms of carbon (black), hydrogen (white), nitrogen (blue), oxygen (red), and sulfur (yellow) are in proportional atomic size. The silver rods indicate chemical bonds that hold the atoms together in a specific three-dimensional shape. (credit: modification of work by Christian Guthier) The elements carbon, hydrogen, nitrogen, oxygen, sulfur, and phosphorus are the key building blocks found in all living things. Elements are unique forms of matter with specific chemical and physical properties that cannot be broken down into simpler substances by ordinary chemical processes. They form the carbohydrates, lipids, proteins, and nucleic acids which are the fundamental components of all organisms. In this chapter, we will discuss how the unique properties of atoms allow them to interact and form the molecules of life. 2.1 The Building Blocks of Molecules At its most fundamental level, life is made up of matter. Matter is any substance that occupies space and has mass. Elements are unique forms of matter with specific chemical and physical Access for free at https://openstax.org/ 25 properties that cannot be broken down into simpler substances by ordinary chemical reactions. There are 118 elements, but only 98 occur naturally. The remaining elements are unstable and require scientists to synthesize them in laboratories. Each element is typically designated by a single capital letter, or two letters if the first letter is already “taken” (Figure 2.2). Some elements follow the English term for the element, such as C for carbon and Ca for calcium. Other elements’ chemical symbols are derived from their Latin names. For example, the symbol for sodium is Na, referring to natrium, the Latin word for sodium. Figure 2.2a The element of hydrogen as designated on a periodic table (credit: Science Activist/Flickr) b. The element of helium as designated on a periodic table (credit: Science Activist/Flickr) The four elements common to all living organisms are oxygen (O), carbon (C), hydrogen (H), and nitrogen (N). Some elements common to all living organisms are relatively rare on the earth as a whole, as Table 2.1 shows. For example, the atmosphere is rich in nitrogen and oxygen but contains very little carbon and hydrogen. The earth’s crust contains oxygen and a small amount of hydrogen but has little nitrogen and carbon. In spite of their differences in abundance, all elements obey the same chemical and physical laws. Approximate Percentage of Elements in Living Organisms (Humans) Compared to the Nonliving World Element Life (Humans) Atmosphere Earth’s Crust Oxygen (O) 65% 21% 46% Carbon (C) 18% trace trace Hydrogen (H) 10% trace 0.1% Nitrogen (N) 3% 78% trace Table 2.1 Approximate Percentage of Elements in Living Organisms (Humans) Compared to the Nonliving World (credit: Clark et al./Biology 2E OpenStax) 26 Return to Table of Contents Organisms cannot make elements; they must come from the environment. An example of an element that humans must take in is calcium (Ca). When you consume dairy products, calcium is absorbed and used for many processes, including strengthening bones, cell division, muscle contraction, and nervous system function. The elements in the human body are shown in Figure 2.3, beginning with the most abundant: oxygen (O), carbon (C), hydrogen (H), and nitrogen (N). Trace elements are also important to the body but in much lower quantities. Iodine, which is a trace element, is required to make thyroid hormone, an important hormone that regulates body metabolism. Trace element deficiencies will lead to homeostatic imbalances within the body. Figure 2.3 Elements of the Human Body - The main elements that compose the human body are shown from most abundant to least abundant. (credit: Betts et al./Anatomy and Physiology OpenStax) The Structure of the Atom To understand how elements come together, we must first discuss the element's smallest component or building block, the atom. An atom is the smallest unit of matter that retains all the chemical properties of the element. For example, one gold atom has all the properties of gold, such as being a solid metal at room temperature. A gold coin is simply a very large number of gold atoms molded into the shape of a coin and contains small amounts of other elements known as impurities. We cannot break gold atoms down into anything smaller while still retaining the properties of gold. Access for free at https://openstax.org/ 27 An atom is composed of two regions. The center of the atom, which is called the nucleus, contains subatomic particles called protons and neutrons. The atom's outermost region holds subatomic particles known as electrons. Electrons orbit around the nucleus, as Figure 2.4. illustrates. All atoms, except hydrogen, contain protons, electrons, and neutrons. Most hydrogen atoms contain only one proton and one electron and have no neutrons. Figure 2.4 Atoms are made up of protons and neutrons located within the nucleus, and electrons surrounding the nucleus. (credit: Clark et al./Biology 2E OpenStax) Protons and neutrons have approximately the same mass, about 1.67 × 10 -24 grams. Scientists arbitrarily define this amount of mass as one atomic mass unit (amu). Although similar in mass, protons and neutrons differ in their electrical charge. A proton is positively charged; whereas, a neutron is uncharged (Table 2.2). The number of neutrons in an atom contributes significantly to its mass, but not to its charge. Electrons are much smaller in mass than protons or neutrons, weighing only 9.11 × 10-28 grams. As a result, electrons do not contribute significantly to an element’s overall atomic mass. When calculating atomic mass, it is customary to ignore the mass of any electrons and calculate the atom’s mass based on the number of protons and neutrons alone. Each electron has a negative charge equal to the positive charge of a proton. In uncharged, neutral atoms, the number of electrons orbiting the nucleus is equal to the number of protons inside the nucleus. The atom will have no charge because the positive and negative charges cancel each other out. Protons, Neutrons, and Electrons Charge Mass (amu) Location Proton + 1 nucleus Neutron 0 1 nucleus Electron – 0 orbitals Table 2.2 shows the characteristics of the three subatomic particles. (credit: Clark et al./Biology 2E OpenStax) Most of an atom's volume, greater than 99 percent, is empty space. With all this empty space, one might ask why solid objects do not just pass through one another. The reason this does not occur is due to the electrons that surround all atoms. Electrons are negatively charged, and negative charges of different objects repel one another, preventing this from occurring. 28 Return to Table of Contents Atomic Number and Mass Atoms of each element contain a unique number of protons and electrons. The number of protons determines an element’s atomic number, which scientists use to distinguish one element from another. For example, hydrogen has an atomic number of 1, meaning it has one proton. Helium has an atomic number of 2, meaning it has two protons in its nucleus (Figure 2.2). All atoms of a particular element will have the same number of protons. The number of neutrons an atom has is variable. Isotopes are different atoms of the same element that vary only in their number of neutrons. Together, the number of protons and neutrons determines an element’s atomic mass number (Figure 2.5). Note that we disregard the small contribution of mass from electrons in calculating the mass number. We can use this approximation of mass to easily calculate how many neutrons an element has by subtracting the number of protons from the mass number. An element’s isotopes will all have slightly different mass numbers. When scientists determine the atomic mass of an element, they take the mean of the mass numbers for all its naturally occurring isotopes. Often, the resulting number contains a fraction. For example, the atomic mass of chlorine (Cl) is 35.45 because chlorine is composed of several isotopes, most with atomic mass 35 (17 protons and 18 neutrons) and some with atomic mass 37 (17 protons and 20 neutrons). Figure 2.5 Carbon has an atomic number of six, and two stable isotopes with mass numbers of twelve and thirteen, respectively. Its relative atomic mass is 12.011 (credit: Clark et al./Biology 2E OpenStax) Answer: C-12 (6 neutrons); C-13 (7 neutrons) Atomic mass of 26 amu. Access for free at https://openstax.org/ 29 Isotopes As mentioned above, isotopes are different forms of an element that have the same number of protons but a different number of neutrons. Hydrogen-1 contains one proton, zero neutrons, and one electron. Hydrogen-2, also called deuterium, has one proton, one neutron, and one electron (Figure 2.6). These two alternate forms of hydrogen are isotopes. Some elements, such as carbon, potassium, and uranium, have naturally occurring isotopes. Carbon-12 contains six protons, six neutrons, and six electrons; therefore, it has a mass number of 12. Carbon-14 contains six protons, eight neutrons, and six electrons; its atomic mass is 14. Some isotopes are unstable and will lose neutrons, other subatomic particles, or energy to form more stable atoms. These are called radioactive isotopes or radioisotopes. Figure 2.6 Isotopes of Hydrogen. (credit: Betts et al./Anatomy and Physiology OpenStax) Evolution in Action Carbon Dating Carbon-14 (14C) is a naturally occurring radioisotope that is created in the atmosphere by cosmic rays. This is a continuous process, so more 14C is always being created. As a living organism develops, the relative level of 14C in its body is equal to the concentration of 14C in the atmosphere. When an organism dies, it is no longer ingesting 14C, so the ratio will decline. 14C decays to 14N by a process called beta decay; it gives off energy in a relatively slow process (Figure 2.7). After approximately 5,730 years, only one-half of the starting concentration of 14C will have been converted to 14N. The time it takes for half of the original concentration of an isotope to decay to its more stable form is called its half-life. Because the half-life of 14C is long, it is used to age dead organisms or objects, such as fossils. 30 Return to Table of Contents Figure 2.7 The age of remains that contain carbon and are less than about 50,000 years old, such as this pygmy mammoth, can be determined using carbon dating. (credit: Bill Faulkner/NPS/Biology 2E OpenStax) CAREER CONNECTION - Interventional Radiologist The controlled use of radioisotopes has advanced medical diagnosis and treatment of disease. Interventional radiologists are physicians who treat disease by using minimally invasive techniques involving radiation. Many conditions that could once only be treated with a lengthy and traumatic operation can now be treated non-surgically, reducing the cost, pain, length of hospital stay, and recovery time for patients. For example, in the past, the only options for a patient with one or more tumors in the liver were surgery and chemotherapy. Some liver tumors, however, are difficult to access surgically, and others could require the surgeon to remove too much of the liver. Chemotherapy is also highly toxic to the liver, and certain tumors do not respond well. In some cases, an interventional radiologist can treat the tumors by disrupting the patient's blood supply. In this procedure, called radioembolization, the radiologist accesses the liver with a fine needle, threaded through one of the patient’s blood vessels. The radiologist then inserts tiny radioactive “seeds” into the blood vessels that supply the tumors. In the days and weeks following the procedure, the radiation emitted from the seeds destroys the vessels and directly kills the tumor cells in the vicinity of the treatment. Radioisotopes emit subatomic particles that can be detected and tracked by imaging technologies. One of the most advanced uses of radioisotopes in medicine is the positron emission tomography (PET) scanner. The procedure begins with administering a very small dose of radioactive glucose, the simple sugar that cells use for energy. The PET camera shows the medical team, which of the patient’s tissues are taking up the most glucose. Thus, the most metabolically active tissues show up as bright “hot spots” on the images (Figure 2.8). PET can reveal some cancerous masses because cancer cells consume glucose at a high rate to fuel their rapid reproduction. Access for free at https://openstax.org/ 31 Figure 2.8 PET Scan PET highlights areas in the body where there is relatively high glucose use, which is characteristic of cancerous tissue. This PET scan shows sites of the spread of a large primary tumor to other sites. (credit: Betts et al./Anatomy and Physiology OpenStax)[/caption] CONCEPTS IN ACTION- To learn more about atoms and isotopes, and how you can tell one isotope from another, visit this site and run the simulation. The Periodic Table The periodic table organizes and displays different elements. Created by a Russian chemist, Dmitri Mendeleev (1834–1907), in 1869, the table groups elements that, although unique, share certain chemical properties with each other. The properties of elements are responsible for their physical state at room temperature; they may be gases, solids, or liquids. Elements also have specific chemical reactivity. Reactivity is the ability of elements to combine and chemically bond with each other. In the periodic table in Figure 2.9, the elements are organized and displayed according to their atomic number and are arranged in a series of rows and columns based on shared chemical and physical properties. In addition to providing the atomic number for each element, the periodic table also displays the element’s atomic mass. Looking at carbon, for example, its symbol (C) and name appear. Its atomic number of six is shown in the upper left-hand corner and its atomic mass of 12.11. 32 Return to Table of Contents Figure 2.9 Arranged in columns and rows based on the characteristics of the elements; the periodic table provides key information about the elements and how they might interact with each other to form molecules. (credit: Concepts of Biology 1st Canadian Edition) Answer: potassium - (20 neutrons) and oxygen - (8 neutrons) The periodic table groups elements according to chemical properties. Scientists base the differences in chemical reactivity between the elements on the number and spatial distribution of an atom’s electrons. Atoms that chemically react and bond with each other form molecules. Molecules are simply two or more neutral atoms chemically bonded together. The atoms that chemically bond may be identical, or they may be different from one another. Chemical compounds differ from molecules in that they are always made up of different types of atoms held together by chemical bonds. Logically, when two atoms chemically bond to form a molecule or compound, their electrons, which form the outermost region of each atom come together first. Access for free at https://openstax.org/ 33 Electron Shells and the Bohr Model There is a connection between the number of protons in an element, the atomic number, and the number of electrons it has. In all electrically neutral atoms, the number of electrons is the same as the number of protons. Each element, at least when electrically neutral, has a characteristic number of electrons equal to its atomic number. In 1913, Danish scientist Niels Bohr (1885–1962) developed an early model of the atom. The Bohr model describes an atom as having a central nucleus containing protons and neutrons. The electrons orbit the nucleus at specific distances (Figure 2.10). These orbits form electron shells or energy levels, which are a way of visualizing the number of electrons in the outermost shells. These energy levels are designated by a number and the symbol “n.” For example, 1n represents the first energy level located closest to the nucleus. Figure 2.10 Bohr model. (credit: Clark et al./Biology 2E OpenStax) Electrons fill orbitals in a consistent order. First, they fill the orbitals closest to the nucleus. Once the closest orbitals are filled, electrons fill orbitals of increasing energy further from the nucleus. The number of electrons in the outermost energy level determines the atom's energetic stability, how reactive or nonreactive an atom is. These electrons determine the tendency of an atom to form chemical bonds with other atoms. Remember, when atoms form chemical bonds with one another, molecules are formed. Under standard conditions, atoms fill the inner shells first, often resulting in a variable number of electrons in the outermost shell. The innermost shell has a maximum of two electrons, but the next electron shell can hold up to eight electrons. This is known as the octet rule, which states, except for the innermost shell, that atoms are more stable energetically when they have eight electrons in their valence shell, the outermost electron shell. Figure 2.11 shows examples of some neutral atoms and their electron configurations. Notice that in Figure 2.11, helium has a complete outer electron shell, with two electrons filling its first and only shell. Similarly, neon has a complete outer 2n shell containing eight electrons. Because these atoms have full outer shells, they are considered stable or non-reactive. In contrast, chlorine has seven and sodium one electron in their outer shells, and therefore they are unstable and more likely to react and form chemical bonds with other atoms. An atom's reactivity is governed by its need to be more energetically stable, which results if their valence shells are full. 34 Return to Table of Contents Figure 2.11 Bohr diagrams indicate how many electrons fill each principal shell. Group 18 elements (helium, neon, and argon) have a full outer or valence shell. A full valence shell is the most stable electron configuration. Elements in other groups have partially filled valence shells and gain or lose electrons to achieve a stable electron configuration. (credit: Clark et al./Biology 2E OpenStax) Answers: (1), (4) Electron Orbitals Although useful to explain the reactivity and chemical bonding of certain elements, the Bohr model does not accurately reflect how electrons spatially distribute themselves around the nucleus. They do not circle the nucleus like the earth orbits the sun, but we find them in electron orbitals. Scientists call the area where an electron is most likely to be found its orbital. While the concepts of electron shells and orbitals are closely related, orbitals provide a more accurate depiction of an atom's electron configuration. Access for free at https://openstax.org/ 35 Section Summary Matter is anything that occupies space and has mass. It is made up of atoms of different elements. All the 98 elements that occur naturally have unique qualities that allow them to combine in various ways to create compounds or molecules. Atoms, which consist of protons, neutrons, and electrons, are the smallest units of an element that retain all of the properties of that element. Exercises 1. How many neutrons do (K) potassium-39 and potassium-40 have, respectively? 2. Magnesium has an atomic number of 12. Which of the following statements is true of a neutral magnesium atom? a. It has 12 protons, 12 electrons, and 12 neutrons. b. It has 12 protons, 12 electrons, and six neutrons. c. It has six protons, six electrons, and no neutrons. d. It has six protons, six electrons, and six neutrons. 3. Oxygen has an atomic number of 8. How many electrons would oxygen need to obtain to be considered stable? a. 1 b. 2 c. 4 d. 8 4. An isotope of sodium (Na) has an atomic mass number of 22. How many neutrons does it have? a. 11 b. 12 c. 22 d. 44 5. Compare and contrast protons neutrons and electrons. Answer 1. Potassium-39 has twenty neutrons. Potassium-40 has twenty-one neutrons. 2. (a) 3. (b) 4. (a) 5. Protons, neutrons, and electrons are all subatomic particles that make up an atom. Protons, which have a positive charge, and neutrons that are electrically neutral, can be found in a defined space called the nucleus. Electrons that are negatively charged are found in orbitals that are arranged in discrete energy levels. 36 Return to Table of Contents Glossary atom: an element's smallest component or building block atomic number: the number of protons in an atom compound: are made up of different types of atoms held together by chemical bonds electron: a negatively charged particle that resides outside of the nucleus in the electron orbital; lacks functional mass and has a charge of –1 element: one of 118 unique substances that cannot be broken down into smaller substances and retain the characteristic of that substance; each element has a specified number of protons and unique properties isotope: one or more forms of an element that have different numbers of neutrons mass number: the number of protons plus neutrons in an atom matter: anything that has mass and occupies space molecules: two or more neutral atoms chemically bonded together neutron: a particle with no charge that resides in the nucleus of an atom; has a mass of 1 nucleus: (chemistry) the dense center of an atom made up of protons and (except in the case of a hydrogen atom) neutrons orbital: an area where an electron is most likely to be found its periodic table of elements: an organizational chart of elements, indicating the atomic number and mass number of each element; also provides key information about the properties of elements proton: a positively charged particle that resides in the nucleus of an atom; has a mass of 1 and a charge of +1 radioactive isotope: an isotope that spontaneously emits particles or energy to form a more stable element reactivity: the ability of elements to combine and chemically bond with each other valence shell: the outermost electron shell Access for free at https://openstax.org/ 37 2.2 Chemical Bonds Chemical Reactions and Molecules According to the octet rule, all elements are most stable when their outermost shell is filled with electrons. This is because it is energetically favorable for atoms to be in that configuration, and it makes them stable. Since not all elements have enough electrons to fill their outermost shells, atoms form chemical bonds with other atoms. Forming chemical bonds allows atoms to obtain the electrons they need to achieve a stable electron configuration. When two or more atoms chemically bond with each other, a molecule is formed. The familiar water molecule, H2O, consists of two hydrogen atoms and one oxygen atom. These atoms bond together by sharing electrons to form the water molecule, as Figure 2.12 illustrates. Atoms can form molecules by donating, accepting, or sharing electrons to fill their outer shells. Figure 2.12 When two hydrogens and an oxygen share pairs of electrons via covalent bonds it forms a water molecule. (credit: Clark et al./Biology 2E OpenStax) 38 Return to Table of Contents Chemical reactions occur when two or more atoms bond together to form molecules or when bonded molecules break apart. We usually call the substances used at the beginning of a chemical reaction reactants, and the substances at the end of the reaction products. We typically draw an arrow between the reactants and products to indicate the chemical reaction's direction. To create a water molecule, the chemical equation would be: 2H + O → H2O An example of a simple chemical reaction is breaking down hydrogen peroxide molecules. Each hydrogen peroxide molecule consists of two hydrogen atoms bonded to two oxygen atoms (H2O2). H2O2 is the chemical formula for hydrogen peroxide. A chemical formula is a way to show how many and which atoms make up a molecule. The reactant hydrogen peroxide breaks down into water (H2O), and an oxygen molecule (O2). In the equation below, the reaction includes two hydrogen peroxide molecules and two water molecules. This is an example of a balanced chemical equation. Each element's number of atoms is the same on each side of the equation. According to the law of conservation of matter, the number of atoms before and after a chemical reaction should be equal. Under normal circumstances, atoms cannot be created or destroyed. 2H2O2 (hydrogen peroxide) → 2H2O (water) + O2 (oxygen) All the reactants and products of this reaction are molecules. However, in this reaction, only hydrogen peroxide and water are chemical compounds meaning they contain atoms of more than one type of atom. Molecular oxygen, as Figure 2.13 shows, consists of two oxygen atoms double-bonded together and is not classified as a compound but as a molecule. Figure 2.13 A double bond joins the oxygen atoms in an O2 molecule. (credit: Clark et al./Biology 2E OpenStax) Access for free at https://openstax.org/ 39 Reversible reactions are those that can go in either direction. In reversible reactions, reactants turn into products, but when the product's concentration goes beyond a certain threshold, some of these products convert back into reactants. This back and forth continues until a certain relative balance between reactants and products occur, a state called equilibrium. A chemical equation with a double-headed arrow pointing towards both the reactants and products often denote these reversible reaction situations. For example, in human blood, excess hydrogen ions (H+) bind to bicarbonate ions (HCO3-) forming an equilibrium state with carbonic acid (H2CO3). If we added carbonic acid to this system, some of it would convert to bicarbonate and hydrogen ions. HCO3−+ H+↔H2CO3 Chemical Bonds Chemical bonds are interactions between two or more atoms that result in the formation of molecules. An atom can donate, accept, or share electrons with other atoms to fill its outer shell and satisfy the octet rule. There are three types of bonds or interactions that will be discussed: ionic, covalent, and hydrogen bonds. Ionic and covalent bonds are strong interactions that require a large input of energy to break the bonds apart. Hydrogen bonds are considered weak bonds because they require less energy to break them apart. Ions and Ionic Bonds When an atom does not contain equal numbers of protons and electrons, it is called an ion. Because the number of electrons does not equal the number of protons, each ion has a net charge. Positive ions are formed by losing electrons and are called cations. Negative ions are formed by gaining electrons and are called anions. For example, sodium only has one electron in its outermost shell. It takes less energy for sodium to donate that one electron than it does to accept seven more electrons to fill the outermost shell. If sodium loses an electron, it now has 11 protons and only 10 electrons, leaving it with an overall charge of +1. It is now called a sodium ion, Na +1 (Figure 2.14a and b). The chlorine atom has seven electrons in its outer shell. Again, it is more energy-efficient for chlorine to gain one electron than to lose seven. Therefore, it tends to gain an electron to create an ion with 17 protons and 18 electrons, giving it a net negative (–1) charge. It is now called a chloride ion, Cl-1 (Figure 2.14a and b). This movement of electrons from one element to another is referred to as electron transfer. 40 Return to Table of Contents As Figure 2.14 illustrates, a sodium atom (Na) only has one electron in its outermost shell, whereas a chlorine atom (Cl) has seven electrons in its outermost shell (Figure 2.14a). A sodium atom will donate its one electron to empty its shell, and a chlorine atom will accept that electron to fill its shell, becoming chloride. Both ions now satisfy the octet rule and have complete outermost shells. Because the number of electrons is no longer equal to the number of protons, each is now an ion and has a +1 (sodium) or –1 (chloride) charge. Figure 2.14 Ionic Bonding (a) Sodium donates the electron in its valence shell to chlorine, which needs only one electron to have a full valence shell. (b) The opposite electrical charges of the resulting sodium cation and chloride anion result in the formation of an ionic bond. (c) The attraction of many sodium and chloride ions results in the formation of large groupings called crystals. (credit: Betts et al./Anatomy and Physiology OpenStax) Access for free at https://openstax.org/ 41 When an element donates an electron from its outer shell, as in the sodium atom example above, a positive ion is formed. The element accepting the electron is now negatively charged. Because cations and anions are attracted to one another, these ions stay together and form an ionic bond or a bond between ions. When proportional amounts of Na+ and Cl– ions combine they produce the ionic compound, NaCl, in a crystallized form (Figure 2.14c). The sodium and chloride ions attract each other in a lattice of ions with a net-zero charge forming what is commonly known as table salt Figure 2.15. Figure 2.15 Edible salt. (credit: Miansari66 / Public Domain) Covalent Bonds A covalent bond is another example of a strong chemical bond that can occur between two or more atoms. A covalent bond forms when one or more pairs of electrons are shared between atoms. These are some of the strongest and most commonly formed chemical bonds in living organisms. Their strength is greatly attributed to the fact that large amounts of energy are required to break these bonds apart. For example, the hydrogen atoms and oxygen atom that combine to form water molecules are bound together by covalent bonds. Each atom participating in a covalent bond must share at least one electron. The electron shared by the hydrogen atom divides its time between the outer shell of the hydrogen atom and the outer shell of the oxygen atom. To