Principles of Antimicrobial Therapy PDF
Document Details
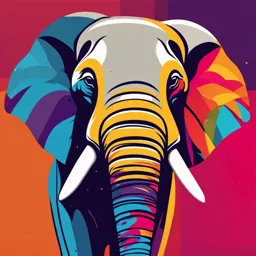
Uploaded by RadiantHyena
null
Tags
Summary
This document provides a detailed overview of antimicrobial therapy, discussing various aspects, including selection of antimicrobial agents, determinants of rational dosing, and potential complications. It also examines the concept of drug resistance from a clinical perspective. It is a useful resource for medical professionals and students focusing on pharmacology and infectious diseases.
Full Transcript
Pharmacology Chemotherapy Principles of Antimicrobial Therapy Principles of Antimicrobial Therapy Content: Selection of Antimicrobial Agents ……………………………………………. 4 Route of Administration ……………………………………………. 38 Determinants of Rational Dosing ……………………………………………. 41 Chemotherapeutic Spectra …………………………………...
Pharmacology Chemotherapy Principles of Antimicrobial Therapy Principles of Antimicrobial Therapy Content: Selection of Antimicrobial Agents ……………………………………………. 4 Route of Administration ……………………………………………. 38 Determinants of Rational Dosing ……………………………………………. 41 Chemotherapeutic Spectra ……………………………………………. 48 Combinations of Antimicrobial Drugs ……………………………………………. 53 Drug Resistance ……………………………………………. 57 Complications of Antibiotic ……………………………………………. 66 Principles of Antimicrobial Therapy Overview: Antimicrobial therapy takes advantage of the biochemical differences that exist between microorganisms and human beings. Antimicrobial drugs are effective in the treatment of infections because of their selective toxicity; that is, they have the ability to injure or kill an invading microorganism without harming the cells of the host. In most instances, the selective toxicity is relative rather than absolute, requiring that the concentration of the drug be carefully controlled to attack the microorganism, while still being tolerated by the host. Selection of Antimicrobial Agents Selection of Antimicrobial Agents Selection of the most appropriate antimicrobial agent requires knowledge of: 1. The identity of the organism. 2. The susceptibility of the organism to a particular agent. 3. The site of the infection. 4. Patient factors. 5. The safety and efficacy of the agent, and, 6. The cost of therapy. However, most patients require empiric therapy (immediate administration of drug(s) prior to bacterial identification and susceptibility testing). Selection of Antimicrobial Agents A. Identification of the Infecting Organism: Characterizing the organism is central to selection of appropriate therapy. A rapid assessment of the nature of the pathogen can sometimes be made on the basis of the Gram stain, which is particularly useful in identifying the presence and morphologic features of microorganisms in body fluids that are normally sterile (blood, cerebrospinal fluid [CSF], pleural fluid, synovial fluid, peritoneal fluid, and urine). However, it is generally necessary to culture the infective organism to arrive at a conclusive diagnosis and determine the susceptibility to antimicrobial agents. Thus, it is essential to obtain a sample culture of the organism prior to initiating treatment. Selection of Antimicrobial Agents A. Identification of the Infecting Organism: Otherwise, it is impossible to differentiate whether a negative culture is due to the absence of organisms or is a result of antimicrobial effects of administered antibiotic. Definitive identification of the infecting organism may require other laboratory techniques, such as detection of microbial antigens, DNA, or RNA, or an inflammatory or host immune response to the microorganism (Figure 1). Newer techniques such as rapid polymerase chain reaction (PCR) and matrix-assisted laser desorption/ionization time-of-flight (MALDI-TOF) mass spectrometry offer accurate, rapid, and cost-effective identification of the infecting organism(s). Selection of Antimicrobial Agents A. Identification of the Infecting Organism: Figure 1 – Some laboratory techniques that are useful in the diagnosis of microbial diseases. Selection of Antimicrobial Agents B. Empiric Antimicrobial Therapy: Ideally, the antimicrobial agent used to treat an infection is selected after the organism has been identified and its susceptibility to antimicrobial agents established. However, in the critically ill patient, such a delay could prove fatal, and immediate empiric therapy is indicated. Selection of Antimicrobial Agents B. Empiric Antimicrobial Therapy: 1. Timing: Acutely ill patients with infections of unknown origin—for example, a neutropenic patient (one who is at risk for infections due to a reduction in neutrophils) or a patient with meningitis (acute inflammation of the membranes covering the brain and spinal cord)—require immediate treatment. If possible, therapy should be initiated after specimens for laboratory analysis have been obtained but before the results of the culture and sensitivity are available. Selection of Antimicrobial Agents B. Empiric Antimicrobial Therapy: 2. Selecting a Drug: Drug choice in the absence of susceptibility data is influenced by the site of infection, the patient history (for example, previous infections, age, recent travel history, recent antimicrobial therapy, immune status, whether the infection was hospital- or community-acquired), and local susceptibility data. Broad-spectrum therapy may be indicated initially when the organism is unknown or polymicrobial infections are likely. The choice of agent(s) may also be guided by known association of particular organisms in a given clinical setting. Selection of Antimicrobial Agents B. Empiric Antimicrobial Therapy: 2. Selecting a Drug: For example, gram-positive cocci in the spinal fluid of a newborn is unlikely to be Streptococcus pneumoniae and most likely to be Streptococcus agalactiae (a group B streptococci), which is sensitive to penicillin G. By contrast, gram-positive cocci in the spinal fluid of a 40-year-old patient are most likely to be S. pneumoniae. This organism is frequently resistant to penicillin G and often requires treatment with a high-dose third-generation cephalosporin (such as ceftriaxone) or vancomycin. Selection of Antimicrobial Agents C. Determination of Antimicrobial Susceptibility: After a pathogen is cultured, its susceptibility to specific antibiotics serves as a guide in selection of antimicrobial therapy. Some pathogens, such as Streptococcus pyogenes and Neisseria meningitidis, usually have predictable susceptibility patterns to certain antibiotics. In contrast, most gram-negative bacilli, enterococci, and staphylococcal species often show unpredictable susceptibility patterns and require susceptibility testing to determine appropriate antimicrobial therapy. The minimum inhibitory and bactericidal concentrations are used in determining susceptibility of a drug and can be experimentally determined (Figure 2). Selection of Antimicrobial Agents C. Determination of Antimicrobial Susceptibility: Figure 1 – Determination of minimum inhibitory concentration (MIC) and minimum bactericidal concentration (MBC) of an antibiotic. Selection of Antimicrobial Agents C. Determination of Antimicrobial Susceptibility: 1. Bacteriostatic Versus Bactericidal Drugs: Antimicrobial drugs are commonly classified as either bacteriostatic or bactericidal. Historically, bacteriostatic drugs were thought to only arrest the growth and replication of bacteria at drug levels achievable in the patient, whereas bactericidal drugs were able to effectively kill ≥99.9% (3-log reduction) within 18 to 24 hours of incubation under specific laboratory conditions. There is a growing consensus that this classification may be too simplistic, as most bacteriostatic agents are able to effectively kill organisms; however, they are unable to meet the arbitrary cutoff value in the bactericidal definition. Selection of Antimicrobial Agents C. Determination of Antimicrobial Susceptibility: 1. Bacteriostatic Versus Bactericidal Drugs: Figure 3 shows a laboratory experiment in which a bactericidal agent is compared to a bacteriostatic agent and a control. Note that the rate of in vitro killing is greater with bactericidal agents, but both agents are able to effectively kill the organism. It is also possible for an antibiotic to be bacteriostatic for one organism and bactericidal for another. For example, linezolid is bacteriostatic against Staphylococcus aureus and enterococci, but is bactericidal against most strains of S. pneumoniae. Selection of Antimicrobial Agents C. Determination of Antimicrobial Susceptibility: 1. Bacteriostatic Versus Bactericidal Drugs: In addition, recent data have demonstrated that bactericidal and bacteriostatic agents have similar efficacy for treating common clinical infections. In the end, other factors may have a greater impact, including the host immune system, drug concentration at the site of infection, and underlying severity of the illness. Selection of Antimicrobial Agents C. Determination of Antimicrobial Susceptibility: 1. Bacteriostatic Versus Bactericidal Drugs: Figure 3 – Effects of bactericidal and bacteriostatic drugs on the growth of bacteria in vitro. Selection of Antimicrobial Agents C. Determination of Antimicrobial Susceptibility: 2. Minimum Inhibitory Concentration: The minimum inhibitory concentration (MIC) is the lowest antimicrobial concentration that prevents visible growth of an organism after 24 hours of incubation. This serves as a quantitative measure of in vitro susceptibility and is commonly used in practice to streamline therapy. Computer automation has improved the accuracy and decreased the turnaround time for determining MIC results and is the most common approach used by clinical laboratories. Selection of Antimicrobial Agents C. Determination of Antimicrobial Susceptibility: 3. Minimum Bactericidal Concentration: The minimum bactericidal concentration (MBC) is the lowest concentration of antimicrobial agent that results in a 99.9% decline in colony count after overnight broth dilution incubations (Figure 2). Note: The MBC is rarely determined in clinical practice due to the time and labor requirements Selection of Antimicrobial Agents D. Effect of the Site of Infection on Therapy - The Blood–Brain Barrier: Adequate levels of an antibiotic must reach the site of infection for the invading microorganisms to be effectively eradicated. Capillaries with varying degrees of permeability carry drugs to the body tissues. Natural barriers to drug delivery are created by the structures of the capillaries of some tissues, such as the prostate, testes, placenta, the vitreous body of the eye, and the central nervous system (CNS). Of particular significance are the capillaries in the brain, which help to create and maintain the blood–brain barrier. Selection of Antimicrobial Agents D. Effect of the Site of Infection on Therapy - The Blood–Brain Barrier: This barrier is formed by the single layer of endothelial cells fused by tight junctions that impede entry from the blood to the brain of virtually all molecules, except those that are small and lipophilic. The penetration and concentration of an antibacterial agent in the CSF are particularly influenced by the following: Selection of Antimicrobial Agents D. Effect of the Site of Infection on Therapy - The Blood–Brain Barrier: 1. Lipid Solubility: The lipid solubility of a drug is a major determinant of its ability to penetrate the blood–brain barrier. Lipid-soluble drugs, such as chloramphenicol and metronidazole, have significant penetration into the CNS, whereas β-lactam antibiotics, such as penicillin, are ionized at physiologic pH and have low lipid solubility. Therefore, they have limited penetration through the intact blood–brain barrier under normal circumstances. Selection of Antimicrobial Agents D. Effect of the Site of Infection on Therapy - The Blood–Brain Barrier: 1. Lipid Solubility: In infections such as meningitis in which the brain becomes inflamed, the barrier does not function as effectively, and local permeability is increased. Some β-lactam antibiotics can enter the CSF in therapeutic amounts when the meninges are inflamed. Selection of Antimicrobial Agents D. Effect of the Site of Infection on Therapy - The Blood–Brain Barrier: 2. Molecular Weight: A drug with a low molecular weight has an enhanced ability to cross the blood– brain barrier, whereas compounds with a high molecular weight (for example, vancomycin) penetrate poorly, even in the presence of meningeal inflammation. 3. Protein Binding: A high degree of protein binding of a drug restricts its entry into the CSF. Therefore, the amount of free (unbound) drug in serum, rather than the total amount of drug present, is important for CSF penetration. Selection of Antimicrobial Agents D. Effect of the Site of Infection on Therapy - The Blood–Brain Barrier: 4. Susceptibility to Transporters or Efflux Pumps: Antibiotics that have an affinity for transporter mechanisms or do not have an affinity for efflux pumps have better CNS penetration. Selection of Antimicrobial Agents E. Patient Factors: In selecting an antibiotic, attention must be paid to the condition of the patient. For example, the status of the immune system, kidneys, liver, circulation, and age must be considered. In women, pregnancy or breast-feeding also affects selection of the antimicrobial agent. Selection of Antimicrobial Agents E. Patient Factors: 1. Immune System: Elimination of infecting organisms from the body is highly dependent on an intact immune system, and the host defense system must ultimately eliminate the invading organisms. Alcoholism, diabetes, HIV infection, malnutrition, autoimmune diseases, pregnancy, advanced age, and immunosuppressive drugs can affect immunocompetence. High doses of bactericidal agents or longer courses of treatment may be required to eliminate infective organisms in these individuals. Selection of Antimicrobial Agents E. Patient Factors: 2. Renal Dysfunction: Poor kidney function may cause accumulation of certain antibiotics. Dosage adjustment prevents drug accumulation and adverse effects. Serum creatinine levels are frequently used as an index of renal function for adjustment of drug regimens. However, direct monitoring of serum levels of some antibiotics (for example, vancomycin, aminoglycosides) is preferred to identify maximum and/or minimum values and prevent potential toxicities. Selection of Antimicrobial Agents E. Patient Factors: 2. Renal Dysfunction: Note: The number of functional nephrons decreases with age. Thus, elderly patients are particularly vulnerable to accumulation of drugs eliminated by the kidneys, even with normal serum creatinine levels. Selection of Antimicrobial Agents E. Patient Factors: 3. Hepatic Dysfunction: Antibiotics that are concentrated or eliminated by the liver (for example, erythromycin and doxycycline) must be used with caution when treating patients with liver dysfunction. 4. Poor Perfusion: Decreased circulation to an anatomic area, such as the lower limbs of a diabetic patient, reduces the amount of antibiotic that reaches that site of infection, making it more difficult to treat. Decreased perfusion of the gastrointestinal tract may result in reduced absorption, making attainment of therapeutic concentrations more difficult with enteral routes. Selection of Antimicrobial Agents E. Patient Factors: 5. Age: Renal or hepatic elimination processes are often poorly developed in newborns, making neonates particularly vulnerable to the toxic effects of agents such as chloramphenicol and sulfonamides. Young children should not be treated with tetracyclines or quinolones, which affect bone growth and joints, respectively. Elderly patients may have decreased renal or liver function, which may alter the pharmacokinetics of certain antibiotics. Selection of Antimicrobial Agents E. Patient Factors: 6. Pregnancy and Lactation: Many antibiotics cross the placental barrier or enter the nursing infant via the breast milk. Prescribers should consult the product labeling of an antibiotic to review the risk summary and clinical considerations for use in pregnancy and lactation. Although the concentration of an antibiotic in fetal circulation or in breast milk is usually low, the total dose to the infant may be sufficient to produce detrimental effects. For example, congenital abnormalities have been reported after administration of tetracyclines to pregnant women, and these agents should be generally be avoided in pregnancy due to the risk to the fetus. Selection of Antimicrobial Agents E. Patient Factors: 7. Risk Factors for Multidrug-Resistant Organisms: Infections with multidrug-resistant pathogens need broader antibiotic coverage when initiating empiric therapy. Common risk factors for infection with these pathogens include prior antimicrobial therapy in the preceding 90 days, hospitalization for greater than 2 days within the preceding 90 days, current hospitalization exceeding 5 days, admission from a nursing home, high frequency of resistance in the community or local hospital unit (assessed using hospital antibiograms), and immunosuppressive diseases and/or therapies. Selection of Antimicrobial Agents F. Safety of the Agent: Penicillins are among the least toxic of all antimicrobial drugs because they interfere with a site or function unique to the growth of microorganisms. Other antimicrobial agents (for example, chloramphenicol) have less specificity and are reserved for life-threatening infections because of the potential for serious toxicity to the patient. Note: Safety is related not only to the inherent nature of the drug but also to the patient factors described above that can predispose to toxicity. Selection of Antimicrobial Agents G. Cost of Therapy: It is common for several drugs to show similar efficacy in treating an infection but vary widely in cost. For example, treatment of methicillin-resistant Staphylococcus aureus (MRSA) generally includes one of the following: vancomycin, clindamycin, daptomycin, or linezolid. Although choice of therapy usually centers on the site of infection, severity of the illness, and ability to take oral medications, it is also important to consider cost of the medication. Selection of Antimicrobial Agents G. Cost of Therapy: Figure 4 – Relative cost of some drugs used for the treatment of Staphylococcus aureus. Route of Administration Route of Administration The oral route of administration is appropriate for mild infections that can be treated on an outpatient basis. Parenteral administration is used for drugs that are poorly absorbed from the GI tract and for treatment of patients with serious infections who require maintenance of higher serum concentrations of antimicrobial agents. In hospitalized patients requiring intravenous (IV) therapy, the switch to oral agents should occur as soon as possible. Switching patients from IV to oral therapy when clinically stable has been shown to decrease health care costs, shorten length of stay, and decrease complications from IV catheters. Route of Administration However, some antibiotics, such as vancomycin and aminoglycosides, are poorly absorbed from the gastrointestinal (GI) tract and do not achieve adequate serum levels via oral administration. Determinants of Rational Dosing Determinants of Rational Dosing Rational dosing of antimicrobial agents is based on pharmacodynamics (the relationship of drug concentrations to antimicrobial effects) and pharmacokinetic properties (the absorption, distribution, metabolism, and elimination of the drug). Three important properties that have a significant influence on the frequency of dosing are concentration-dependent killing, time-dependent (concentrationindependent) killing, and post-antibiotic effect (PAE). Utilizing these properties to optimize antibiotic dosing regimens can improve clinical outcomes and possibly decrease the development of resistance. Determinants of Rational Dosing A. Concentration-Dependent Killing: Certain antimicrobial agents, including aminoglycosides and daptomycin, show a significant increase in the rate of bacterial killing as the concentration of antibiotic increases from 4- to 64-fold the MIC of the drug for the infecting organism (Figure 5A). Giving drugs that exhibit this concentration-dependent killing by a once-a-day bolus infusion achieves high peak levels, favoring rapid killing of the infecting pathogen. Determinants of Rational Dosing A. Concentration-Dependent Killing: Figure 5A – Significant dose- dependent killing effect shown by tobramycin. B.Nonsignificant dose-dependent killing effect shown by piperacillin. cfu = colony-forming units; MIC = minimum inhibitory concentration. Determinants of Rational Dosing B. Time-Dependent (Concentration-Independent) Killing: In contrast, β-lactams, glycopeptides, macrolides, clindamycin, and linezolid do not exhibit concentration-dependent killing (Figure 5B). The clinical efficacy of these antimicrobials is best predicted by the percentage of time that blood concentrations of a drug remain above the MIC. This effect is sometimes called time-dependent (or concentration-independent) killing. For example, dosing schedules for the penicillins and cephalosporins that ensure blood levels greater than the MIC for 50% and 60% of the time, respectively, provide the most clinical efficacy. Determinants of Rational Dosing B. Time-Dependent (Concentration-Independent) Killing: Therefore, extended (generally 3 to 4 hours) or continuous (24 hours) infusions can be utilized instead of intermittent dosing (generally 30 minutes) to achieve prolonged time above the MIC and kill more bacteria. Other drugs, such as fluoroquinolones and vancomycin, work best by optimizing the ratio of the 24-hour area under the concentration–time curve to MIC (AUC24 /MIC). The AUC24 is the overall exposure of a drug during the dosing interval and takes into account the concentration as well as the time. Determinants of Rational Dosing C. Postantibiotic Effect: The PAE is a persistent suppression of microbial growth that occurs after levels of antibiotic have fallen below the MIC. Antimicrobial drugs exhibiting a long PAE (for example, aminoglycosides and fluoroquinolones) often require only one dose per day, particularly against gramnegative bacteria. Chemotherapeutic Spectra Chemotherapeutic Spectra In this book, the clinically important bacteria have been organized into eight groups based on Gram stain, morphology, and biochemical or other characteristics. They are represented as a color-coded list (Figure 6A). The ninth section of the list is labeled “Other,” and it is used to represent any organism not included in one of the other eight categories. In Figure 6B–D, the list is used to illustrate the spectra of bacteria for which a particular class of antibiotics is therapeutically effective. Chemotherapeutic Spectra Figure 6 – A. Color-coded representation of medically important microorganisms. B. Isoniazid, narrow-spectrum antimicrobial agent. C. Ampicillin, an extended-spectrum antimicrobial agent. D. Tetracycline, a broad-spectrum antimicrobial agent. Chemotherapeutic Spectra A. Narrow-Spectrum Antibiotics: Chemotherapeutic agents acting only on a single or a limited group of microorganisms are said to have a narrow spectrum. For example, isoniazid is active only against Mycobacterium tuberculosis (Figure 6B). B. Extended-Spectrum Antibiotics: Extended spectrum is the term applied to antibiotics that are modified to be effective against gram-positive organisms and also against a significant number of gramnegative bacteria. For example, ampicillin is considered to have an extended spectrum because it acts against gram-positive and some gram-negative bacteria (Figure 6C). Chemotherapeutic Spectra C. Broad-Spectrum Antibiotics: Drugs such as tetracycline, fluoroquinolones and carbapenems affect a wide variety of microbial species and are referred to as broad-spectrum antibiotics (Figure 6D). Administration of broad-spectrum antibiotics can drastically alter the nature of the normal bacterial flora and precipitate a superinfection due to organisms such as Clostridium difficile, the growth of which is normally kept in check by the presence of other colonizing microorganisms. Combinations of Antimicrobial Drugs Combinations of Antimicrobial Drugs It is therapeutically advisable to treat patients with a single agent that is most specific to the infecting organism. This strategy reduces the possibility of superinfections, decreases the emergence of resistant organisms, and minimizes toxicity. However, in some situations, combinations of antimicrobial drugs are advantageous or even required. Combinations of Antimicrobial Drugs A. Advantages of Drug Combinations: Certain combinations of antibiotics, such as β-lactams and aminoglycosides, show synergism; that is, the combination is more effective than either of the drugs used separately. Because such synergism among antimicrobial agents is rare, synergistic combinations are only indicated in special situations (for example, in the treatment of enterococcal endocarditis). Combinations may also be used when an infection is of unknown origin or when there are organisms with variable sensitivity, such as when treating tuberculosis. Combinations of Antimicrobial Drugs B. Disadvantages of Drug Combinations: A number of antibiotics act only when organisms are multiplying. Thus, coadministration of an agent that causes bacteriostasis plus a second agent that is bactericidal may result in the first drug interfering with the action of the second. For example, bacteriostatic tetracycline drugs may interfere with the bactericidal effects of penicillins and cephalosporins. Another concern is the risk of selection pressure and the development of antibiotic resistance by giving unnecessary combination therapy. Drug Resistance Drug Resistance Bacteria are considered resistant to an antibiotic if the maximal level of that antibiotic that can be tolerated by the host does not halt bacterial growth. Some organisms are inherently resistant to an antibiotic. For example, most gram-negative organisms are inherently resistant to vancomycin. However, microbial species that are normally responsive to a particular drug may develop more virulent or resistant strains through spontaneous mutation or acquired resistance and selection. Some of these strains may even become resistant to more than one antibiotic. Drug Resistance A. Genetic Alterations Leading to Drug Resistance: Acquired antibiotic resistance requires the temporary or permanent gain or alteration of bacterial genetic information. Resistance develops due to the ability of DNA to undergo spontaneous mutation or to move from one organism to another (Figure 7). Drug Resistance A. Genetic Alterations Leading to Drug Resistance: Drug Resistance B. Altered Expression of Proteins in Drug-Resistant Organisms: Drug resistance is mediated by a variety of mechanisms, such as an alteration in an antibiotic target site, decreased penetration of the drug due to lower permeability, increased efflux of the drug, or presence of antibiotic-inactivating enzymes (Figure 7). 1. Modification of Target Sites: Alteration of the target site of an antibiotic through mutation can confer resistance to one or more related antibiotics. For example, S. pneumoniae resistance to β-lactam antibiotics involves alterations in one or more of the major bacterial penicillin-binding proteins, resulting in decreased binding of the antibiotic to its target. Drug Resistance B. Altered Expression of Proteins in Drug-Resistant Organisms: 2. Decreased Accumulation: Decreased uptake or increased efflux of an antibiotic can confer resistance because the drug is unable to attain access to the site of its action in sufficient concentrations to injure or kill the organism. For example, gram-negative organisms can limit the penetration of certain agents, including β-lactam antibiotics, as a result of an alteration in the number and structure of porins (channels) in the outer membrane. Also, the presence of an efflux pump can limit levels of a drug in an organism, as seen with tetracyclines. Drug Resistance B. Altered Expression of Proteins in Drug-Resistant Organisms: 3. Enzymatic Inactivation: The ability to destroy or inactivate the antimicrobial agent can also confer resistance on microorganisms. Examples of antibiotic-inactivating enzymes include: 1. β-lactamases (“penicillinases”) that hydrolytically inactivate the βlactam ring of penicillins, cephalosporins, and related drugs. 2. Acetyltransferases that transfer an acetyl group to the antibiotic, inactivating chloramphenicol or aminoglycosides; and 3. Esterases that hydrolyze the lactone ring of macrolides. Principles of Antimicrobial Therapy Prophylactic Use of Antibiotics: Certain clinical situations, such as dental procedures and surgeries, require the use of antibiotics for the prevention rather than for the treatment of infections (Figure 8). Because the indiscriminate use of antimicrobial agents can result in bacterial resistance and superinfection, prophylactic use is restricted to clinical situations in which the benefits outweigh the potential risks. The duration of prophylaxis should be closely controlled to prevent the unnecessary development of antibiotic resistance. Principles of Antimicrobial Therapy Figure 8 – Some clinical situations in which prophylactic antibiotics are indicated. Complications of Antibiotic Complications of Antibiotic Complications of Antibiotic: A. Hypersensitivity: Hypersensitivity or immune reactions to antimicrobial drugs or their metabolic products frequently occur. For example, the penicillins, despite their almost absolute selective microbial toxicity, can cause serious hypersensitivity problems, ranging from urticaria (hives) to anaphylactic shock. B. Direct Toxicity: For example, aminoglycosides can cause ototoxicity by interfering with membrane function in the auditory hair cells. Complications of Antibiotic C. Superinfections: Drug therapy, particularly with broad-spectrum antimicrobials or combinations of agents, can lead to alterations of the normal microbial flora of the upper respiratory, oral, intestinal, and genitourinary tracts, permitting the overgrowth of opportunistic organisms, especially fungi or resistant bacteria. These infections usually require secondary treatments using specific anti-infective agents. Sites of Antimicrobial Action Figure 9 – Classification of some antimicrobial agents by their sites of action. THFA = tetrahydrofolic acid; PABA = p-aminobenzoic acid.