PML Lab File - Proximate Analysis of Coal - PDF
Document Details
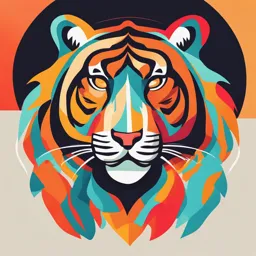
Uploaded by ReformedConnemara1344
Tags
Summary
This document outlines an experiment on proximate analysis of coal. It details the theory, apparatus, procedures, and observations for determining the moisture, volatile matter, ash, and fixed carbon content of a coal sample. Key terms include coal, proximate analysis, and different components of coal.
Full Transcript
Experiment 1 : PROXIMATE ANALYSIS OF COAL Aim: To conduct the proximate analysis of a coal sample. Apparatus: Alumina crucible, weighing balance, oven, muffle furnace, desiccators and coal samples. Theory: Coal is a highly carboneous matter that has been formed as a result of transformation of bi...
Experiment 1 : PROXIMATE ANALYSIS OF COAL Aim: To conduct the proximate analysis of a coal sample. Apparatus: Alumina crucible, weighing balance, oven, muffle furnace, desiccators and coal samples. Theory: Coal is a highly carboneous matter that has been formed as a result of transformation of biological matter (dead plants and animals) under conditions of heat and pressure for millions of years. It is mainly composed of C, H, N & O, besides noncombustible matter such as silica and alumina. The following properties determine the selection of coal for any particular purpose. Calorific Value Calorific value of a fuel (J/kg) is the quantity of heat liberated (J) per unit quantity of fuel (kg), burnt completely. The carbon and hydrogen content of the fuel converted to CO2 and H2O, respectively, are the products of combustion. If the combustion products are cooled down to 25 oC, then the total heat released (chemical heat + sensible heat) is called the gross calorific value. Presence of components other carbon and hydrogen in the fuel, decreases its calorific value and hence its quality. Moisture Content This increases the weight of coal and increases the cost of its transportation. Presence of excessive moisture may quench the fire in the furnace. Volatile Matter In the pyrolysis of coal, i.e. heating of coal in the oxygen-free atmosphere, several components bonded to coal are released as volatile product. The volatile components are H2, CH4, CO, CO2, N2, NH3, H2S, higher hydrocarbons, tar and chlorides. Although some of these components have calorific value, they are not directly useful for a metallurgical reactor and therefore their presence decreases the rank of the coal. Ash Content Coal is associated with certain inorganic matter, which gets deposited along with vegetable matter or gets into coal by subsequent infiltration (during the formation of coal over millions of years). The ash consists mainly of silica, alumina, iron oxide and lime. Higher ash content decreases the efficiency of all furnaces. Fixed Carbon Fixed carbon content increases with the coal rank, in the order of peat → lignite → bituminous → anthracite. Coal Analyses The rank (quality) of a coal is decreased due to the presence of (i) moisture content, (ii) volatile matter and (iii) ash content; the estimation of the mass fraction of these three components is called the proximate analysis of coal. In contrast, performing the chemical analysis of coal to determine the mass fractions of C, H, O, N, S, Cl, etc., is known as the ultimate analysis. Proximate analysis of Indian Coal (mass fraction) Moisture 14% Volatile matter 21% Ash 42% Fixed carbon 31% Procedure: The following procedure is common to determination of moisture, volatile matter and ash content. 1. Weigh the empty crucible. 2. Take around one gram of coal sample in the crucible and weigh it. 3. Place the crucible in the furnace. In the case of volatile matter analysis, the crucible is closed with a lid to prevent the oxidation of coal by air in the furnace. The furnace temperature and certain dwell time for each case is given below. Component analyzed Furnace temperature, oC Dwell time, minutes Moisture 100 60 Volatile matter 900 10 Ash 700 60 4. Remove the crucible with the help of tongs, place it in a desiccator and allow it to cool. 5. Weigh the crucible with the residue in it. Fig. Open and closed crucibles, the latter one is for analysis of volatile matter. Observations Expt. Crucible (Crucible + Sample (Crucible + Residue Experiment No. mass, g Sample)mass, g mass, g Residue) mass, g mass, g 1 Moisture 2 Volatile matter 3 Fixed Carbon Let the mass fractions of moisture, volatile matter, fixed carbon and ash content be denoted by YM, YVM, YFC and YA, respectively. Then the mass fractions are deduced from the following. YM = 1 – (residue mass)Expt_1 / (sample mass) Expt_1 YVM = 1 – (residue mass)Expt_2 / (sample mass) Expt_2 – YM YFC = 1 – (residue mass)Expt_3 / (sample mass) Expt_3 – YM – YVM YA = 1 – YM – YVM – YFC Results The proximate analysis of coal yields the following: 1. Moisture content ____% 2. Volatile Matter ____% 3. Fixed Carbon ____% 4. Ash content ____% Experiment 2 : DEMONSTRATION OF FROTH FLOTATION Aim: To observe the froth floatation process. Theory: The froth flotation process exploits the differences in surface properties of minerals to facilitate the separation of particles into concentrate and gangue. In the flotation process, a slurry (or pulp, containing solid and water in the ratio of about 1:2 by volume) is fed into a tank, which is the floatation cell. Compressed air is injected through a rotating agitator at the base of the cell that disperses the bubbles throughout the cell volume. The following floatation agents are added to aid the process, all in small but calculated quantities: 1. Frother – to create stable bubbles that rise and collect at the top of the cell called as froth. Pine oil is a very common frothing agent. 2. Collector – this modifies the surface of particles in the slurry so that it becomes hydrophobic and attaches itself to a bubble when encountered. Various forms of Xanthates are commonly used as collectors. 3. Activator – acts to enhance the action of Collector by modifying the particle surface suitable to the action of Collector. 4. Depressor – acts to suppress the action of collector. A particle is said to be hydrophobic if it is not wetter by water. A hydrophobic particle thus prefers a bubble over water and will eventually be floated to the surface by the bubble. However, coarser particles might not float due to their weight. Hence griding to fine size is essential for efficient floatation. Most minerals are not hydrophobic in their natural state and hence suitable floatation agents are necessary for modifying the surface properties. The combination of collector, activator and depressor can be manipulated to selectively target the particles containing the mineral of interest such that only they are collected in the froth and the rest sink to the bottom of cell as tailings. Process: A weighed quantity of sulphide ore powder is taken. About 2 times by volume of the sample powder, water is added to prepare a slurry that is poured into the floatation cell. Few drops of pine oil are added to the slurry Compressed air is blown into the slurry through an agitator. A froth rises up and overflows via a launder which is collected in a bucket. Solid particles are seen to be attached to the bubbles of froth. The process is continued until particles cease to appear on the bubbles in the froth. The collected froth is washed in water and is screened to separate the particulate matter, which is the concentrate. The concentrate is dried and weighed. The mass % of mineral of interest in both the initial sample and the concentrate is determined by chemical analysis. The recovery of the mineral of interest is deduced from the measured mass and grade of the feed and concentrate using the standard formula for recovery. Experiment 3 : JAW CRUSHER Aim: To determine the efficiency of jaw crusher for a given ore material. Materials / apparatus required: 1. Ore sample 2. Jaw crusher 3. Sieve shaker 4. Electronic weighing balance Theory: A jaw crusher uses compressive force for breaking of particle, via the two jaws of the crusher of which one is fixed while the other reciprocates. There are three main types of Jaw crushers: Blake Jaw Crusher: It has its moving jaw pivoted at the top. It is classified on the basis of single or double toggle type. A jaw crusher has two jaws said to form a V-shaped at the top through which feed is admitted. One of the jaw is fixed into the main frame and other is movable. The crushing faces are usually made of Hadfield–Mn steel (12-14%Mn, 1%C). The jaw crusher speed varies between 100 and 400 RPM. Gape: It is the distance between jaw plates at the fixed feed end. Set: The distance between the jaw plates in the discharge end. Dodge-Jaw Crusher: In the Dodge Crusher, the moving jaw is pivoted at the bottom. The minimum movement is thus at the bottom and a more uniform product is obtained. However, this type of crusher is not commonly used due to its tendency to choke. The larger opening at the top enables it to take very large feed and to effect a large size reduction. This crusher is usually made in smaller sizes than the Blake-Jaw Crusher due to high fluctuating stresses that are produced in the members of the machine. Universal crusher-In a universal jaw crusher, the pivot is fixed at an intermediate position. Procedure: Write in your own words (in Bullet points) consisting of – Cleaning of jaw crusher – setting of gap at the discharge end – taking about 100g of dry feed sample – crushing – collecting – weighing – cleaning of sieve bottom tray – setting up of sieve and bottom tray in sieve shaker – sieving of crushed sample – weighing of undersize particles. Precautions: Safety glasses or goggles should be worn at all times. When working around crushers stay behind safety barriers. Observations and Calculations: Sl. Jaw crusher Mass of Sieve size, Mass of Crushing No. discharge-end- crushed mm undersize, g efficiency, gap, mm sample, g % 1. 2. The efficiency of the jaw crusher is calculated as the ratio between the mass of undersize and the mass of crushed sample. Inference: Write your comments on the observed relationship between discharge-end-gap and crushing efficiency Results: Thus, the jaw crusher efficiency is estimated for two different gaps at the discharge end of the jaw crusher. Experiment 4 : RATE OF SCREENING Aim: To determine the time for 90% screening efficiency of the given sieve and sieve shaker. Apparatus Required: A sieve shaker, meshes of a given size, sample powder, weighing machine, collecting tray and a stopwatch. Theory: A screen separates a mass of particles based on their size and yield two products, namely the Undersize that contains the particles that pass through the screen and the other is Oversize. Dry screening at the industrial scale is generally limited to material above 5 mm in size, while wet screening down to 250 μm. Size separation below 250 μm is more commonly undertaken by classification, because the efficiency of screens decreases rapidly with particle fineness. In industrial screening, the material to be screened is fed at one end of a tilted screen that is vibrated in order to expose every particle to the screen aperture and to convey the particles down the screen. The time for which the particles stay on the screen, while they travel down, is the retention time. Given a very long retention time all the undersized particle will pass through the screen (100% efficiency). However, the retention time should be kept smaller as possible to minimize the screen size and energy required for screening. Usually, a 90% efficiency is an optimal target. The following factors adversely affect the screening efficiency. 1) Screening blinding: Presence of particles of the size nearly the same as the screen aperture size, plug the aperture thereby blocking that area for screening. 2) Over feeding: High feed rate prevents the chance for all particles to see the screen aperture within the retention time. 3) Higher tilt angle of screen: Enhances the rate of screening, but does not offer enough time for all particles to see the screen, (similar to the case of over feeding) 4) Particle shape: Needle-like and plate-like particles have the most unfavourable orientation to pass through the screen aperture 5) Excessive vibration: causes excessive bouncing of particles unless the feed rate is high enough to have bed of considerable thickness. For this experiment, we screen using the sieve shaker therefore the sieving time is the retention time. The screening efficiency for a given sieve size is defined as the ratio between (i) the mass of particles in the pan (Undersize) obtained for a given sieving time and (ii) the mass of particles in the pan obtained for a very long sieving time. Procedure: Write in your own words (in Bullet points) consisting of – Size of sieve taken – cleaning of sieve –sieve shaker – initial mass of sample –use of stop clock – mass of sample in underflow in pan after sieving - Observations: Sieve Size = ____ µm Initial mass of sample = ____ g Trial Sieving Mass of sample in pan Screen No. Time (s) (g) (Undersize) Efficiency % 0 0 - 0 1 2 3 4 5 𝑡→∞ 𝑚𝑎𝑠𝑠 𝑜𝑓 𝑠𝑎𝑚𝑝𝑙𝑒 𝑖𝑛 𝑡ℎ𝑒 𝑝𝑎𝑛 𝑆𝑐𝑟𝑒𝑒𝑛𝑖𝑛𝑔 𝑒𝑓𝑓𝑖𝑐𝑖𝑒𝑛𝑐𝑦 (%) = 𝑚𝑎𝑠𝑠 𝑜𝑓 𝑠𝑎𝑚𝑝𝑙𝑒 𝑖𝑛 𝑝𝑎𝑛 𝑠𝑖𝑒𝑣𝑒𝑑 𝑓𝑜𝑟 𝑡 → ∞ Analysis: Construct a smooth plot of time versus the screen efficiency and deduce the time taken for 90% efficiency. Result: Thus, for a sieve size of _____ µm, the time taken for 90% screen efficiency is determined to be ______ s. Experiment 5 : SETTLING RATE IN SEDIMENTATION Aim: To determine the settling rate of a given dispersion using sedimentation process. Apparatus Required: 1L measuring cylinder, water, MgCO3 or CaCO3 powder, stirrer and stopwatch Theory: Sedimentation is the process of gravitational settling of insoluble particles suspended in a liquid medium, resulting in an upper zone of clarified liquid and a lower zone of settled-particles. Sedimentation principle is largely employed in dewatering (also known as thickening, solid–liquid separation) and in classification (ore concentration, solid–solid separation). The settling rate of a single, spherical particle in a motionless fluid at very low Reynolds number (Re < 0.2, as in the case of particles less than 40 µm) is given by Stoke’s law: 𝑔∆𝜌𝑑 2 𝑣(𝑚/𝑠) = 18 𝜂 2 where, d is particle size, g = 9.8 m/s , ∆𝜌 is the density difference between particle and liquid and 𝜂 is the fluid viscosity. From this equation it is inferred that particles larger in size and with larger density difference with the fluid medium, have higher settling rates. in general, the settling rate is also affected by the shape of particles (particles are rarely spherical), the nature of fluid flow and the concentration of particles in the fluid (mass per unit volume of particle-fluid mixture). Depending on the concentration of particles in the fluid medium, say water, the particle settling pattern is classified as follows. 1. Free settling: Under conditions of low particle concentrations, the individual particles settle freely, i.e., unhindered by other particles in a medium of still water or against a rising current of water. 2. Hindered settling: This occurs when the particles settle among a crowded mass of other particles in a medium of still water or against a rising current of water. Fig.1 : Schematic of Sedimentation of suspended particles Procedure: 1. To prepare a dispersion of certain concentration, take a measured quantity of given sample (magnesium carbonate) and add it to water in the 1L measuring cylinder. 2. Mix the contents thoroughly for uniform concentration with the help of a stirrer. 3. Stop mixing and start observing the cylinder for the particles to settle down. 4. Track the location of the interface formed between a rather clear liquid in the upper portion and turbid liquid beneath it. Note down the times at which the interface crosses the major graduations of the measuring cylinder. 5. Continue the procedure until there is no perceptible change in the interface movement. 6. A graph is plotted for interface locations versus time. The water surface level is taken as zero for the interface location. 7. The settling rate is found from the analysis of the graphs. Observations: Table 1: Tracking of Interface location with time S. No Location of interface Time taken (mm) (s) 1. 2. 3. 4. 5. 6. 7. 8. Analysis: The settling curve shall exhibit three regimes (see graph below): (i) an initial slow settling, (ii) steady state settling due to particles having reached their terminal velocities, marked by a steeper curve (iii) final slow settling marked by a flat curve. The average slope of the steady settling regime is the average settling rate of particles. Interface location slope = average settling rate Time, s Result: For a concentration of ____ g of sample powder / liter of water, the average settling rate is ____ cm/s Experiment 6 : SIEVE ANALYSIS AND DETERMINATION OF SIZE DISTRIBUTION IN SAMPLE Aim: To perform sieve analysis and determine the size distribution of powdered sample and hence determine the 50% and 80% passing sizes. Apparatus required: 1. Mechanical sieve shaker including stack of sieves, pan and cover 2. Balance (with accuracy to 0.01 g) Note: The balance to be used should be sensitive to the extent of 0.1% of total weight of sample taken. Test Specimen: The ASTM standard provides a recommendation on the size of the sample used in sieve analysis. If the apparent density of the powder is larger than 1.50 g/cm3, a sample size between 90-110g should be used. Otherwise, a smaller sample between 40-60g may be used. The size distribution of the sample should be such that there is no more than 5% of total mass each in the topmost sieve and in the pan at the bottom. Theory: Sieve analysis is accomplished by passing a known mass of sample powder successively through finer sieves and weighing the amount collected on each sieve to determine the percentage mass in each size class. Sieving is carried out with wet or dry materials and the sieves are usually agitated to expose all the particles to the openings. The process of sieving may be divided into two stages. First, the elimination of particles considerably smaller than the screen apertures, which should occur fairly rapidly and, second, the separation of the so-called "near-size" particles, which is a gradual process rarely reaching final completion. The effectiveness of a sieving test depends on the amount of material put on the sieve (the "charge") and the type of movement imparted to the sieve. Procedure: Write in your own words (in Bullet points) consisting of – Cleaning of sieves – stacking of sieve trays & pan and locking in sieve shaker - amount of initial sample – after shaking, collecting and weighing the mass retained in each tray. Analysis: From the measured mass retained in each sieve, the mass % retained and hence the cumulative % retained are determined. The cumulative mass % of Oversize is determined as the sum of all mass % in the size ranges smaller than and including the current size range. The cumulative mass % of Undersize is determined as (1 – Oversize). The size distribution and the cumulative Undersize size distributions are plotted on the same graph with the Nominal aperture size in the x-axis and mass % in the y-axis. The passing sizes (X50 and X80) are deduced by reading the values on the x-axis, corresponding to the point of the intersection of mass% lines (for 50% and 80%) with the cumulative size distribution curve. Observations: Nominal Sieve Size Mass of Cumulative mass% Aperture Mass % Range retained Size (µm) retained (µm) sample (g) Oversize Undersize +2360 2360 -2360 +1200 1200 -1200 +600 600 -600 +300 300 -300 +150 150 -150 +75 75 -75 +38 38 -38 (pan) 19 Total = Undersize (%) = 1 – Oversize (%) Result: Thus, the particle size distribution and the cumulative size distribution are determined and hence the following parameters are deduced: X50 = _______ µm X80 = _______ µm Experiment 7 : VISCOSITY MEASUREMENT USING REDWOOD VISCOMETER Aim: To determine the viscosity of the given oil using redwood viscometer at different temperatures Apparatus: Redwood Viscometer 50ml Receiving flask Thermometer Stopwatch Theory: Viscosity is a measure of resistance to fluid flow. The role of viscosity is clearly manifested in laminar flows, wherein the fluid flow occurs layer by layer, the flow of a faster moving layer is impeded by the adjoining slower moving layer due to viscosity. For Newtonian fluids, viscosity is a property of the fluid measured by the coefficient of viscosity according to the Newton’s law of viscosity, 𝑑𝑣 𝜏=𝜇 𝑑𝑥 𝑑𝑣 where, 𝜏 (N/m2) is the shear stress exerted by one layer over the other, 𝑑𝑥 is the strain rate (1/s) and 𝜇 (Pa.s) is the coefficient of viscosity. A viscous fluid, whose viscosity to be measured, is allowed to flow through a narrow channel (an orifice) in which laminar flow occurs and its flow rate is measured. The Newton’s law of viscosity equation is then expressed in terms of flow rate and channel dimensions such that the viscosity coefficient can be deduced. This is the principle of viscosity measurement using Redwood Viscometer. Description of the apparatus: Redwood Viscometer consists of the following. a) A cylindrical oil cup that has (i) an oil-fill-level indicator (ii) an orifice at the bottom to enable fluid flow (iii) a concave depression at the mouth of the orifice to facilitate a tight seal with a stopper rod with a ball-tip, that enables start or stop of the oil flow. b) A water jacket surrounding the oil cup, supported on a tripod stand and provided with (i) a circular electrical immersion heater and (ii) a stirring device. c) Two thermometers to measure water bath and oil temperatures. d) A 50ml flask. The setup is standardized so that the time required to fill 50 ml is directly used in the following simple formula to deduce the viscosity. 171 𝜇(𝑃𝑎. 𝑠) = 𝜌 (0.26 𝑡 − ) × 10−6 𝑡 where, 𝜌 is the density of the viscous fluid and 𝑡 is the filling time in seconds. Procedure: 1. Clean the oil cup with a solvent preferably Carbon Tetrachloride and wipe it dry thoroughly with paper napkins or a soft cloth (do not use cotton waste) and the orifice jet with a fine thread. 2. Keep the water bath with an oil cup on the tripod stand and level it. 3. Pour water into the water bath up to 15 to 20 mm below the top portion. 4. Keep the stopper rod in sealing position and pour a clean filtered oil sample (use strainer not coarser than BS 100 mesh) to be tested into the oil cup up to the gauge point and cover itwith the lid. 5. Clean and dry the 50 ml flask and place it under the orifice of the oil cup. 6. Turn on the electrical heater and stir the water jacket and oil continuously while monitoring the two temperatures. Because the water heats up faster than the oil, the water should be heated up to 5 ºC above the desired oil temperature and then turn off the heater. The stirring of both the mediums should continue until the oil reaches the desired temperature. 7. Lift the stopper rod and simultaneously start a stopwatch and allow the oil into the receiving flask. 8. Stop the watch when the oil level touches the 50 ml mark. 9. Repeat the experiment at different temperatures above ambient. Observations: The mean density of the oil in the temperature range of 30 to 70 ºC is 860 kg/m3 Expt. No. Temp. of oil, ºC Time (s) Viscosity coefficient, for collecting oil of 50 ml 𝜇 (Pa.s) Results: The coefficient of viscosity is estimated and plotted in the graph against varying temperatures.