Plant Study Notes (Biology 1B-1.10105) PDF
Document Details
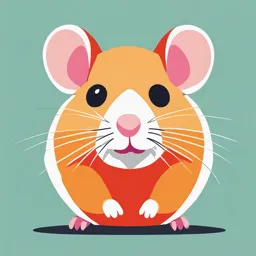
Uploaded by QuietSaxhorn
University of Papua New Guinea
Prof.Lance Hill. Edited by: B.Waiin & R.Totome
Tags
Summary
These study notes cover plant structure and function, including the roles of plants in ecosystems, plant cell organelles, tissues, and organ systems. It provides an overview of plant form and function. The document is for a Biology 1B course at the University of Papua New Guinea.
Full Transcript
UNIVERSITY OF PAPUA NEW GUINEA SCHOOL OF NATURAL & PHYSICAL SCIENCES DIVISION OF BIOLOGICAL SCIENCES PLANT STUDY NOTES Course Name: BIOLOGY 1B Course No.:...
UNIVERSITY OF PAPUA NEW GUINEA SCHOOL OF NATURAL & PHYSICAL SCIENCES DIVISION OF BIOLOGICAL SCIENCES PLANT STUDY NOTES Course Name: BIOLOGY 1B Course No.: 1.10105 Author: Prof.Lance Hill. Edited by: B.Waiin & R.Totome Printed at University of Papua New Guinea Printers, Waigani Campus Parts of this publication is taken from Organisms Diversity Structure & Function, ISBN: 9980-84-703-4 This publication has been for purpose of study for this UPNG course and may not be reproduced, stored or transmitted, in any form or by means, without the prior permission of the publisher. Front Cover Image: Adapted from images from Depositphotos. UNIT 9: PLANT STRUCTURE AND FUNCTION 2 U NI T 9 : P L AN T S T R UC T U RE AN D F U NC TI O N Objectives After you have completed the work of this unit you should be able to: 1. List several important roles plants play in the life of humans. 2. Draw a flowering plant and label the main parts and state their functions. 3. Understand and describe the differences between plant and animal cells. 4. Understand and describe the functions of plant organelles and cell walls. 5. Describe the different cell types and understand their functions. 6. Understand and describe the different plant tissue types. 7. Describe the difference between simple and complex tissues and name the cells found in the different tissues. 8. Understand and describe the differences between primary and secondary growth. 9. State the function of roots. 10. Understand and describe the relationship between form and function in the different zones of a young dicot root. 11. Describe the pathways of water and minerals movement into the root and up the stem to the leaves. 12. Describe the pathway of movement of sugars in the phloem. 13. Describe the difference between the roots of monocots and dicots. 14. State and understand the function of shoots. 15. Describe the differences between the monocot and dicot stems. 16. Understand and describe the difference between primary and secondary growth in stems. 17. Describe several types of modified stems. 18. Describe the difference between monocot and dicot leaves. 19. Describe modifications found in some leaves. 20. Understand and describe the differences between simple and compound leaves. 21. Understand and describe the internal structure of monocot and dicot leaves. A comment on the use of the unit notes Read through these Unit notes to gain an overview of what is covered in this section of the course. You should then review the PowerPoint presentations on this topic that that can be acquired from the course coordinator. You will also undertake further practical activities related to this Unit. And do remember your glossary building! Introduction In this and the next Unit we will examine the structure and function of the remarkable organisms we call plants. All animals are dependent on plant life either directly or indirectly. Of course we use plants for food and many other direct needs such as medicines, buildings materials and clothes. However, plants play a much more essential part in our life since they trap sunlight energy and convert it into carbohydrates via photosynthesis. In the process they also produce the oxygen that is essential for the maintenance of most life. We shall also see that they play other important roles in ecosystems such as nutrient cycling, soil stabilisation and providing places for other organisms to live. 3 Plants are autotrophs that can manufacture their own organic nutrients from inorganic raw materials. Green plants require carbon dioxide and water as raw materials. In addition they require certain mineral elements. They use sunlight energy to drive the synthesis of organic compounds. Plant and animal cells are basically similar in structure and have many of the same organelles. However, plant cells differ from animal cells by having a cell wall of cellulose surrounding the entire cell; this provides support and gives shape to the cells. Plant cells also have some specialised organelles such as chloroplasts (the sites of photosynthesis) and a large central vacuole. The presence of the cell wall around plant cells limits movement of the cell and restricts direct cell-to-cell communication to the plasmodesmata, threads of cytoplasm that run through holes in the cell walls between adjacent cells. The cells of the plant do not function independently, but are organised, like animal cells, into tissues or groups of similar cells performing some specific task. We saw earlier in the course that animals had four basic tissue types. All plant tissues can be divided into two major categories: meristematic and permanent tissue. Plants have separate, specialised regions, called meristems, where new cells of the plant body are formed. The most important meristems are the apical meristems, that occur near the extreme tips of the shoot and root and at the tips of side branches. Rapid cell division can occur in the meristems. These cells can grow and mature into different types of permanent tissues. We recognise three primary permanent tissues: epidermal, ground and vascular tissue. The epidermal tissue forms a single layer of cells that cover the plant surface. The ground tissue is generally located between the epidermis and the vascular (transporting) tissue. It can be made up of several different cell types – parenchyma, collenchyma, sclerenchyma or pith cells. The vascular tissue is composed of phloem and xylem. Both function in transporting materials around the plant body. Phloem is specialised for transporting the products of photosynthesis from the leaves and other photosynthetic tissue to other parts of the plant. Phloem contains sieve tube elements and companion cells. On the other hand, the xylem is specialised for the transport of water and inorganic salts from the roots to other parts of the plant. The conducting cells are called tracheids and xylem vessel elements. The different tissue types of plants are arranged together into organs. Plants form four basic types of organs, namely, roots, stems, leaves and flowers. Roots anchor the plant in place and collect water and mineral salts from the soil. These are transported through the xylem tissue in the root and stem of the plant to the leaves. The leaves take up carbon dioxide via small openings called stomates and together with the absorbed sunlight and water produce glucose via photosynthesis. Oxygen produced during photosynthesis is released into the atmosphere through the stomates. Angiosperms (flowering plants) now dominate the plant kingdom. Their aboveground shoots consist of stems, leaves, and reproductive parts called flowers. Most species have roots that grow downward and outward through soil. The monocots and dicots are the main angiosperm groups. They have the same tissues organized in somewhat different ways. For example, they differ in the number of cotyledons, with monocots having one and dicots having two. A bud is the start of a new stem, leaves, and flowers. Stems support upright growth and conduct water and solutes through vascular bundles. Monocot stems often have vascular 4 bundles distributed through ground tissue. Most dicot stems have a ring of bundles dividing the ground tissue into cortex and pith. A leaf has veins and mesophyll between an upper and lower epidermis. Air spaces around the photosynthetic cells enhance gas exchange. Water vapour and gases cross the epidermis through stomata. Roots absorb water and dissolved mineral ions for distribution to aboveground parts. Dicots typically have a taproot system, and many monocots have a fibrous root. Xylem and phloem occur in the root's vascular system. Root hairs increase surface area for absorption of water and nutrients. For many land plants, a mutualism, such as mycorrhizae, or root nodules with bacterial symbionts, assists in nutrient uptake. Roots often control uptake of water and solutes at the endodermis and exodermis. Both are cylinders of cells that have a Casparian strip. The cohesion-tension describes how water absorbed from soil moves up through the tracheids and vessel members of xylem to leaves. By transpiration, dry air around leaves promotes evaporation through stomata. Evaporation pulls columns of water molecules that are hydrogen bonded to one another from roots to leaves. A cuticle and stomata help conserve water. A stoma is an opening across the epidermis of a leaf. When open, it permits gas exchange. When closed, it slows water loss. Changes in the turgor pressure of guard cells control stomatal opening and closure. Sucrose and other organic compounds are distributed throughout the plant by the energy- requiring process of translocation. Sugars produced by photosynthesis are loaded into conducting cells of phloem (sieve tubes). Companion cells function in the loading. Sugars are unloaded at the plant’s actively growing regions or at storage regions. The pressure flow theory describes how solutes are pushed from one region to another by pressure gradients. Woody plants add secondary growth by divisions of cells of lateral meristems, the cork cambium and vascular cambium. Wood is classified by location and function (as in heartwood or sapwood) and plant type. A cross section of a tree from a temperate climate shows growth rings but the situation in tropical trees is much less clear because of the less differentiated seasons. The importance of plants Think about all the different uses you make of plants in a week! Throughout the world plants form the basis of life. Plants provide us with food, medicines, dyes and poisons; they also provide the raw materials needed to build houses, canoes, rafts, tools, weapons, clothing and storage container's of all sorts. In addition to their direct use to us, plants play several important roles in the biosphere we call earth: Plants are the producers upon which most terrestrial animals and decomposers depend for energy Plants are the major source, through the process of photosynthesis, of oxygen in the air. Plants play important roles in all nutrient cycles (i.e. carbon, sulphur, nitrogen, oxygen, phosphorus, and potassium) and in the water cycle. 5 Forest trees and other plant roots stabilise the soil and prevent erosion in our watersheds and plants such as mangroves are important in stabilising our coastlines. In addition to food, plants provide many animals with shelter and breeding places. Introduction to the Angiosperms or flowering plants There are many different types of plants from quite simple forms such as mosses to the more complex ferns, conifers and flowering plants. In this Unit we will concentrate on the flowering plants, since they are the dominant plants on earth today. They dominate in the number of species as well as biomass and ground cover. Go outside and find a small flowering plant and carefully dig it up. Wash the soil away from the roots and have a close look at its overall organisation. As you work through these notes you will be able to identify the various structures on your specimen. You will see that flowering plants normally consists of a root system and a shoot system (see Figure 1). The shoot system of most plants is aerial and is composed of stems, leaves, flowers and fruits, whereas the root system of most plants is usually in the soil. These various plant parts must, as in animals, work together. For example, the leaves on the stem carry out photosynthesis to produce carbohydrates. Some of this is stored as starch, while other products are mobilised and transported from the leaves to the roots where they are used for new growth and maintenance. Similarly, roots absorb minerals and water, which travels through the root, to the stem and up to the leaves, where it is used for maintenance and new growth in the stems. In addition, roots are often used to store carbohydrates for later use by the plant (e.g. kaukau, cassava, and winged bean). Plants seldom grow a set number of branches, as animals grow a set number of legs, eyes or other parts. In fact because plants are rooted and cannot move about, they have very interesting adaptations to the environment. One of these is that both the root and the stem are able to grow continuously. Over the life of the plant, the stem continues to produce new branches (with new leaves, flowers and fruits) and the roots continue to grow spreading in proportion to the growth of the stem. This ability to continuously add new parts is due to special tissues called meristems. If we look at Figure 1, we see that roots usually grow downward into the soil. Their main functions are to: absorb water and minerals needed by plants for growth and reproduction anchor the plant in the soil store food reserves Stems, on the other hand, usually grow upward. They have various functions, which include: supporting the leaves to ensure that they are exposed to the sun (for purposes of photosynthesis) conducting water and minerals from the roots to the leaves and buds conducting the products of photosynthesis from the leaves to the rest of the plant. - supporting and presenting flowers for pollination and fertilisation supporting the fruit as it matures with the growing embryos within its seed(s) storing food reserves and minerals. 6 FIGURE 1: The structure of a typical flowering plant. Leaves are the sites of photosynthesis. The sugars produced in leaves are used by all parts of the plant for growth and respiration. Leaves also 'transpire'. Transpiration is the evaporation of water from the leaf. This process 'pulls' water and minerals from the roots up through the stems and into the leaf and out of the stomates (pores in the leaf that serve for gas exchange and transpiration). Flowers contain the sexual reproductive parts of plants, see Figure 2. The male gametes (sperm) are produced and carried in the pollen grains, which in turn are produced in the anthers. The female gametes (eggs) are produced within the ovule, which is within the ovary. FIGURE 2: The structure and floral parts of a generalized flower. Did you see these various structures on your flowering plant? 7 Activity 1 1. Complete and fully label your plant drawing in Exercise 2 of the Practical Activity notes at the end of this Unit. 2. Match the following: _____ a. Leaves 1. Contain sexual reproductive organs _____ b. Roots 2. In sugar cane much food is stored here _____ c. Stem 3. Most photosynthesis occurs here _____ d. Flowers 4. Seeds are often dispersed in this structure _____ e. Fruit 5. Absorb water and mineral nutrients. Plant Cells Although there are many similarities between plant and animal cells, plant cells have several unique features (see Figure 3). For instance, plant cells have some organelles that are not found in animal cells. These include cell walls, vacuoles, plastids and plasmodesmata. These unique features of plants are discussed below. A generalised plant cell has two components: the living protoplasm and the non-living cell wall. The protoplasm consists of the cytoplasm and the nucleus. The cytoplasm itself consists of numerous organelles and membranes that are suspended in the ground material of the cytoplasm. Looking at a plant cell from the outside in (Figure 3) the cell contents are surrounded by a cell wall. Inside the cell wall is the plasma membrane, also called the cell membrane. Cell Membrane The cell membrane separates the contents of the cell from the cell wall. In addition, it has several important functions. The most important of these are to: control the transport of substances in and out of the cell coordinate synthesis of the cell wall constituents and assembly of the wall translate environmental signals involved in growth and differentiation The cell membrane is described as semi-permeable because it allows only some substances to pass through. Since molecules of different sizes move through at different rates, the membrane is also considered to be differentially permeable. You can learn more about the fascinating structure and function of cells in another course you can take later called Introductory Cell Biology. Osmosis is the movement of water across a semi-permeable membrane from an area of high concentration (of water) to an area of low concentration. While osmosis is important in all living organisms, it is especially important in plants. When water enters the plant cell, it enters the vacuole. As the vacuole fills with water, it expands and presses against the cell membrane and then against the cell wall. This pressure, called turgor pressure, causes the 8 cell wall to stretch and the cell to grow. All increase in cell size occurs in this manner. In healthy cells there is a balance between the pressure from the vacuole (turgor pressure) and the resistance pressure the wall exerts inwards (wall pressure). When water leaves the vacuole and the cell, and the cell contents collapse. This collapse is termed plasmolysis. When a plant wilts because of lack of water, the water leaves the vacuole and the pressure of the wall inwards is greater than the pressure exerted by the vacuole outwards. The result is that the cell collapses. If water is then added, the plant might recover and regain its strength. On a cellular level, water will have re-entered the vacuole. You will have observed the results of plasmolysis many times – think about the way plants collapse when the ground is very dry. And think about why shop attendants and ladies in the market spray water onto their produce to keep it looking fresh and crisp! Figure 3: A generalized plant cell. Plastids Plastids are common components of plant cells. They are surrounded by two membrane layers. Plastids also contain their own DNA, RNA and ribosomes, as do mitochondria, and like mitochondria, they are able to reproduce by a dividing process called fission. New plastids are produced first when the cell is initially formed, and later, if the need arises, through fission. The most common plastids are chloroplasts, chromoplasts and leucoplasts. Chloroplasts are the most important and probably the most abundant of the plastids. In a leaf mesophyll cell there may be 40-50 chloroplasts, each being from 4 to 6 µm in size. Chloroplasts are the site of photosynthesis and this is where the green (chlorophyll) and yellow (carotenes) pigments are synthesised, stored, and used. 9 Chloroplasts (see Figure 4) contain a complex network of membranes called thylakoid membranes that are embedded in a matrix called the stroma. These membranes are folded into stacks called grana. The entire system of membranes is connected; the outer membrane to the stroma thylakoids and these to the grana thylakoids. The pigments used in photosynthesis, the chlorophylls and carotenes together with the enzymes and other chemical necessary to sunlight energy capture, are found embedded in these membranes. The different reactions of photosynthesis occur either on the membrane or in the stroma close to the membranes Figure 4: Structure of a chloroplast. Chloroplasts also contain starch grains and lipid (fat) droplets. These are the temporary storage products of photosynthesis. Chromoplasts are plastids that are not involved in photosynthesis and therefore lack both internal membranes and chlorophyll. Instead, these plastids synthesise and store carotenes, the yellow/orange pigments. It is chromoplasts that give colour to yellow and orange flowers, fruits, and leaves. Leucoplasts are colourless plastids that do not contain pigments. Amyloplasts are a type of leucoplast. These are clear colourless plastids that synthesise and store starch. The cells of English potato are composed largely of amyloplasts. (A note of exceptional interest: You may be wondering why mitochondria and chloroplast contain their own DNA, RNA and ribosomes that allows them to reproduce without direction from the nucleus? Why is the membrane surrounding these organelles different from the membrane surrounding the nucleus and other organelles? In fact there are other similarities between mitochondria and chloroplasts. In several ways these organelles are different from other organelles, but similar to bacteria. Other similarities include: their DNA is loose and in a circular form; the RNA and ribosomes are also loose in the matrix and the ribosomes of chloroplasts are similar in size to those of bacteria (smaller in size than those of eukaryotic ribosomes). Finally, protein synthesis in bacteria and chloroplasts is inhibited by similar antibiotics! This observations intrigued biologists for a long time. Today, the accepted theory is one put forth by Dr. Lynn Margulis. She believes that these organelles originated as 10 prokaryotic bacterial cells that entered into symbiotic relationships with larger cells and eventually evolved into the structures known today. Thus, if a large cell engulfed a smaller cell and then became dependent upon the energy produced by the smaller cell, this might be the first step in the process.) Vacuoles and other organelles Vacuoles are fluid-filled sacs surrounded by a single layer of membrane called a tonoplast. The immature cell has many small vacuoles that later fuse into one large vacuole as the cell matures. Eventually, the vacuole takes up most of the space in the plant cell. Vacuoles have many important functions. Increase in cell size is due to the growth of the vacuole. As the vacuole grows it presses outward against the cell membrane and cell wall, causing the cell wall to stretch. This pressure is known as turgor pressure. Turgor pressure and cell rigidity are due to the pressure of water in the vacuole pressing outward against the cell wall. When the vacuole loses water, the cell collapses. This is known as plasmolysis. When plants wilt and droop due to lack of water, the wilting is due to the loss of water in the vacuole, which leads to lack of pressure against the walls, and therefore to wilting as mentioned earlier in this Unit. The vacuole fluid contains sugars, salts, proteins and other cell products; sometimes these are in such large quantities that crystals can be observed in the vacuoles. These crystals sometimes make our food feel ‘itchy’ at the back of our mouth when we eat them! Active transport occurs across the tonoplast, moving the salts, sugars, proteins and other molecules into the vacuole, against the concentration gradient. This is an indication that vacuoles may be involved in water balance, since water enters the cell through osmosis, due to the high concentration of salts and sugars in the vacuoles. Vacuoles can also act as storage areas for metabolites, reserve protein in seeds, and anthocyanins (the blue-red pigments). Toxic secondary products of metabolism are stored and broken down in the vacuole, thereby protecting the rest of the cell. Vacuoles are also similar to lysosomes in animal cells in that they are believed to be involved in the breakdown and recycling of macromolecules (for example ribosomes, plastids and mitochondria may be degraded in the vacuole). Microbodies are very small organelles (less than 1.5µm) that occur in plant cells. They have a single membrane surround and are associated with the endoplasmic reticulum. Very little is known about them at this time because they are so small. However, we do know that there are two types. Glyoxosomes are organelles that play a role in seed germination. They contain enzymes that break down the fats stored in seeds into carbohydrates, which are then utilised by the growing embryo. Peroxisomes are another type of organelle that is known to be important in photorespiration. Plasmodesmata are strands of ER that extend between cells, through the pores in the cell wall. They connect the ER of adjacent cells (see Figure 3) allowing communication to take place between the cells. Note that the cell membranes of adjacent cells are also connected. 11 Cell Wall All plant cells are surrounded by a non-living cell wall. The wall acts to keep the cell from exploding and to give strength to the plant. The primary cell wall (see Figure 4) is formed while the cell is growing, and continues to grow as the cell grows. Its most important constituents are the carbohydrates cellulose and pectin. Cellulose gives strength to cell walls. It is composed of long chains of glucose molecules, bonded together and wrapped around each other. Cellulose is a strong as a chain of steel of similar size. Pectin, on the other hand, gives plasticity to cell walls, enabling them to stretch as the cells are growing. The middle lamella is an area shared by adjacent cell walls. The middle lamella is composed mainly of pectin, giving plasticity to the wall and allowing the adjacent cells to grow. Figure 4: General structure of plant cell walls. Cell walls are porous and allow water and dissolved substances to enter and leave. In addition, all primary cell walls have pores or pits. These are areas where the wall is thin. Strands of plasmodesmata go through these pores and connect adjacent cells. Plasmodesmata are the pieces of endoplasmic reticulum (ER), a network of membranes within all eukaryotic cells. In addition, some cells also have secondary walls. These secondary walls are laid down between the primary wall and the cell membrane, after the cell has completed its growth. These walls are very thick and strong, and contain lignin, a chemical that adds considerable strength to the wall. They do not contain pectin. Cells with secondary walls die at maturity, and actually function as dead cells, for example xylem and fibres. The cuticle is a layer of the chemical cutin on the outside of epidermal cells of leaves and green stems. Cutin is a waxy material, which helps prevent water loss through evaporation. The woody epidermis, or bark, usually contains the waxy or 'corky' material called suberin. 12 Activity 2 3. Describe the structure of a generalised plant cell and explain how it differs from an animal cell. 4. The cell wall of a plant is generally stretched by pressure generated from within the cell. This pressure is caused by water entering the: A. mitochondria B. vacuole C. plastids D. nucleus E. endoplasmic reticulum Cell and Tissue Types All cells in the plant body arise from meristematic tissue that is composed of parenchyma cells. These cells are perpetually embryonic, or undifferentiated. This means that they retain the ability to divide and differentiate, i.e. to become another cell type. When a meristematic cell divides, one cell moves into the plant body and differentiates. This cell is called the derivative. The other cell called the initial, remains in the meristem to divide again. Although plant growth occurs from several meristems, two meristems - the apical meristem and the vascular cambium (Figure 5) - are responsible for most plant growth. Plants grow in two main ways. Primary growth is due to apical meristems. These are located at the tips (the apex) of all stems and roots and are responsible for growth that increases the length of roots and stems. All growth from apical meristems is considered primary growth - the stems grow upward and the roots downward. Secondary growth is due to the vascular cambium; this is responsible for an increase in width or girth in both stems and roots. Whenever a vascular cambium is active, and a third cambium is also active called the cork cambium. The cork cambium is responsible for producing the periderm, or bark of a tree or shrub. Tissues are groups of cells that are structurally and/or functionally distinct from other groups of cells. Tissues may be simple (all one cell type) or complex (containing more than one cell type). Tissues, in turn, are organised into tissue systems. The three tissue systems in plants are: Ground (or fundamental) system The vascular system The dermal system It is important to understand that these systems are connected throughout the plant body. For instance, the vascular system in the roots is connected to the vascular system in the stem through to the leaves. It is somewhat like the circulatory system in the animal body. The ground tissue system is composed of the following tissues: Parenchyma Collenchyma Sclerenchyma 13 Figure 5: Major meristems in a plant. Figure 6 shows the derivation of the various tissue and cell types of roots and stems from the meristems. Basically, the ground system is composed of parenchyma cells. The other systems are embedded in the ground system.. FIGURE 6: Origin of primary tissues and specialised cells in the root and stem. 14 The vascular tissue system is composed of two tissues (Figure 7), the xylem and phloem. The xylem is the tissue system responsible for the uptake and movement of water and minerals throughout the plant. The main cells are either tracheids and vessels. These cells are dead when mature and active. Other cells in xylem are sclerenchyma (fibres) for strength and parenchyma cells. The phloem is the system responsible for moving the products of photosynthesis to the rest of the plant body. The main cells are sieve cells and companion cells. Phloem also has sclerenchyma fibres and parenchyma. The arrangement is shown in Figure 8. Figure 7: Representative cells of complex plant tissues. The epidermis is formed from the apical meristem. It is the outer covering of leaves, flowers, fruits, and green stems. Parenchyma cells are the main cell types. Interspersed among the parenchyma cells are guard cells and stomata. Stomata are pores, which function in gas exchange and transpiration. Guard cells surround the stomata. A waxy layer of cutin covers the epidermis. This functions to protect against water loss and to some extent against physical damage. The periderm, also called bark is a secondary tissue. This is formed whenever wood is formed. 15 Figure 8: Diagram of sieve tube and companion cell The dermal tissue system is composed of either epidermis or periderm Table below provides a summary of the different tissues and cell types in a plant. Activity 3 5. Match the following: _________________ a. Cells have thick, hard 1. parenchyma secondary walls and lack protoplasm at maturity _________________ b. Living tissue with active 2. Collenchyma protoplasm providing mechanical support _________________ c. Cells contain chlorophyll 3. Sclerenchyma and can manufacture food _________________ d. Capable of meristematic 4. Both parenchyma and collenchyma _________________ e. Principle function is storage 5. Parenchyma, of water and storage and collenchyma and manufacture of food sclerenchyma 6. What is the function of xylem and phloem? We now turn to look in a little more detail at the root and shoot systems. 16 TABLE 1: Basic tissue types and functions in angiosperms. TISSUE TYPE TISSUE LOCATION FUNCTION Apical meristems, Buds, cambia in roots Production of new cells Meristematic cambium and stems and tissues Storage and packing; Cortex and pith in roots support by turgidity; Ground tissues Parenchyma and stems; mesophyll in photosynthesis; leaves meristematic Support Collenchyma Cortex in stem and leaves Fibres in cortex of stems and leaves; sclereids in Sclerenchyma Support parenchyma Protection: cuticle to reduce water loss; stomata for gas exchange; Dermal tissues Epidermis Outer surface root hairs for mineral and water uptake Protection: water- Corky cover in woody proofing; lenticels for gas Periderm parts produced by exchange cambium Root: stele Primary stem: vascular Xylem: vessels, bundles Water and mineral Vascular tissues tracheids, fibres and Secondary stem: central conduction; support parenchyma cylinder Leaf: veins Root: stele Sieve tubes: conduction Phloem: sieve Primary stem: vascular of photosynthates tubes, companion bundles Companion cells: provide cells, fibres and Secondary stem: inside energy for sieve tubes; parenchyma bark support Leaf: veins Root Systems Roots have several important and diverse functions. These include the absorption of necessary water and minerals from the surrounding soil, anchorage to the substrate, and storage of carbohydrates (e.g. kaukau, cassava, and winged bean) and other substances. 17 Morphology and Function of Roots Figure 9: The root tip of a dicot plant. root cap is also thought to exude a 'slime' that contributes to the protection of the apical meristem. Above the apical meristem is the zone of cell division. In this area new cells are produced from the apical meristem, and they in turn divide to produce more cells. Above this is the zone of cell elongation. Remember that plant growth occurs from a combination of cell elongation as well as cell division. Cell elongation is due to the cell wall stretching as water enters the vacuole, allowing the cell to increase in size. Finally, above this area is the zone of cell differentiation and maturation. In this area the cells differentiate into the various cell types and reach maturity. Note that root hairs are only present in this area. Why do you think this is so? Think about what is happening to the root tip below the zone of cell differentiation and what might happen to root hairs if they occurred in these two zones. Figure 10 also shows a transverse section of the same root. Note the epidermis, cortex and the endodermis surrounding the vascular cylinder. The endodermis is composed of tightly packed parenchyma cells with a layer of suberin around them; this forms the casparian strip. The cortex is composed of parenchyma cells that have spaces between them (see Figure 11). This allows water and other materials to pass between the cells, moving along the cell walls. However, the casparian strip of the endodermis does not allow water to pass between these cells. Therefore, all substances must move through the cells of the endodermis to pass into 18 the vascular cylinder. In this way, the endodermis exerts a degree of control over substances entering the vascular cylinder. Figure 10: Transverse section showing the primary tissues in a dicot root. The first layer of cells within the endodermis is the pericycle and within that the xylem and phloem. The pericycle is meristernatic tissue that gives rise to lateral roots. Absorption of water and minerals The absorption of water and mineral salts are essential for all plant growth and activity. Water and minerals enter the root through the root hairs of young actively growing roots. Water enters by osmosis; this is a passive process and therefore does not require energy. Minerals are also absorbed through the root hairs, but energy (ATP) is required for minerals to enter the plant. As shown in Figure 11, the pathway of water and minerals is: soil → root hairs → epidermis → cortex → endodermis → pericycle → xylem Notice that water initially enters the root by osmosis. The water moves between the cells, along the cell walls (apoplastic movement). In this way it continues to move through the epidermis and cortex until it reaches the endodermis. Here, due to the casparian strip, the water must pass through the cells of the endodermis (symplastic movement) to enter the vascular cylinder. Once here, it may again move through the apoplastic pathway between the cells until reaching and entering the xylem. 19 Figure 11: Pathways for entry of water and minerals into the root. Minerals enter the roots in the form of dissolved ions. The initial movement into the roots uses energy and involves a form of active transport. After that, the ions move through the cells using the symplastic pathway. Another important function of roots is that of storage of energy-rich compounds. For instance, roots often store carbohydrates, which are then used by the plant to survive harsh conditions, such as winter in temperate areas or periods of drought in temperate and tropical areas (e.g. savannas). Humans have learned to take advantage of this and cultivate many plants based on the carbohydrates stored in their roots. Carrots and sweet potato are examples of this. Types of roots Roots are classified into three kinds, according to their origin. The primary root is the first-formed root, which develops directly from the embryonic root (the radicle) in the seed. Lateral roots that develop from the primary root. These roots originate in the pericycle, just within the endodermis. This type occurs in docotyledons. Fibrous roots consists of many roots of equal size that forms form a dense network from the base of the stem. They occur in monocotyledons. Adventitious roots which develop from nodes on stems; they can occur in both dicots and monocots. (see Figure 12). 20 Figure 12: Root system types: A. Tap root (primary) and B. Fibrous roots and C. Adventious roots. In all flowering plants, a primary root forms from the embryonic root. However, subsequent development is different in monocotyledons and dicotyledons. In dictots, the primary root goes on to develop into the tap root system (Figure 12), with lateral roots growing from the main tap root system. On the other hand, in monocots, the original root is lost as the plant grows, and all mature roots arise from nodes on the stem. Because there is no main root, they develop into a fibrous root system. Roots that grow from stems are termed adventitious roots. Dicots may also have adventitious roots, in addition to their tap root system. The ability of dicots to sprout roots at the nodes of stems is the basis for the propagation and cloning of many species. Edible and other uses of roots Many plant species store a large amount of nutritive material in their roots and we exploit these species as edible roots and harvest them at the end of their growth cycle. Recall that one of the functions of parenchyma is storage. Storage occurs in parenchymal cells of the roots, which enlarge in the vascular tissue (as in radishes or beets) or in the cortex (carrots and parsnips). Some tropical foods, like cassava as well as the sweet potato, are the roots of herbaceous perennials. Figure 13 shows various modified roots that are used for storage. Radishes, Raphanus sativus, also belong to the mustard (cabbage) family. In the radish, nearly the entire mass of the root is expanded xylem, with the phloem pushed out to a thin layer on the periphery. Radishes vary in morphology from the red globe-shaped radishes commonly seen in markets to the large white or purple-skinned daikon radish often used in Asian food preparations. The major root crop of the family Apiaceae is the carrot (Caucus carota). The "inner" portion of the carrot is the xylem and the outer portion is cortex. The original cultivated 21 carrot was purple; since the purple pigment (anthocyanin) is water soluble, soups and stews made from carrots resulted in purplish brown dishes—rather unappetizing in appearance. The yellow-orange form was a mutant, which occurred naturally, and ultimately became the form cultivated today. The pigment responsible for the orange colour of carrots is beta- carotene. When digested, it is broken down into two vitamin A molecules and absorbed in that form. Vitamin A supplies the precursor for retinol, the light absorbing pigment in the rods of the retina. Manihot esculenta is the manioc, or cassava plant. It is a very important tropical root crop. It is the staple food of over 500 million people worldwide. It is a storage root, and is composed almost entirely of starch. It is nearly an ideal plant, as it grows well in nearly any climate, poor soil, and is resistant to insects and fungal pests. Although it has high carbohydrate content, it is very low in protein and vitamins. The root also contains poisons and cyanogenic glycosides, which must be removed before eating by boiling. Some spices, such as sassafras, sarsaparilla, licorice, and angelica come from roots. Medicinal products obtained from roots include ipecac, ginseng, and reserpine (an anti- psychotic tranquilizer). Ipecac is derived from Cephaelis apecacuanha, a member of the coffee family. It is an emetic (induces vomiting), and is important in poison control—when some poisons are accidentally ingested, emetics are often used to expel the poison. Emetics are not used for ingestions of strong acids or bases (if your child drinks drain cleaner get to the hospital quiockly!). Reserpine is extracted from snakeroot (Rauwolfia serpentina). This compound is concentrated in the root and was first isolated in 1952. The use of reserpine revolutionized the treatment of mental illness. Before reserpine was used, treatments for schizophrenia involved shock treatment or injections of insulin. Both of these often resulted in violent reactions. Reserpine calms patients and acts as an antagonist to norepinephrine, a neurotransmitter of the sympathetic nervous system. Rotenone is an insecticide derived from the Derris plant. It also may be used to kill fish. When added to a stream or pond it kills the fish. It is relatively harmless to humans. Figure 13: Root storage modifications. Activity 4 7. List four functions of roots. 22 8. Why do plants have root hairs? Suppose you were an atom of calcium and that you were entering a dicotyledon root through a root hair. Trace your path from the soil to the centre of the root by listing the root parts in the sequence you would pass through them. Shoot Systems The shoot system, which comprises the aboveground portion of most plants, is composed of stems. The leaves, flowers and fruit are attached to the stem. Although each of these has their own function, they are connected and interact and work together. Support Stems of non-woody herbaceous dicots and monocots have primary growth (from the apical meristem) only. The overall tissue organisaton is shown in Figure 13. These stems are able to grow upwards due to the support offered by several types of cells contained in the vascular cylinder. These include tracheal elements, whose thick walls give strength and support, and sclerenchyma cells, commonly called fibres. Turgor pressure, due to water in the vacuole, provides additional support. If the cells of herbaceous plants lose sufficient water, the plant will wilt. Figure 13: The structure of the herbaceous dicot stem showing the main tissue organisation and the arrangement in the vascular bundle. The ascent of the xylem fluid or sap up the plant mainly depends on the physical properties of water and he transpiration pull. Water molecules have properties of cohesion and adhesion – the molecules cling to each other because of their chemical structure and the interaction between the water molecules. As water is lost by transpiration from the leaves the column of sap is pulled up the plant stem. We can think of the movement being the result of a transpiration-cohesion-tension mechanism that is powered by sunlight. Carefully examine Figure 14 which illustrates these processes. The movement of fluid in the phloem occurs by a different process. As you read through this paragraph examine Figure 15. Leaves and other photosynthetic parts of the plant are the main source of sugars. These are manufactured by photosynthesis and must be moved to other parts of the plant either for immediate use or for storage, for example in 23 Figure 14: Adhesion, cohesion and transpiration pull in xylem sap movement. the fruit, stems of roots. These storage sites can be thought of as sinks. Sucrose moves from the mesophyll cells (the source) of the leaf along symplast pathway to the sieve tubes of the phloem where it is actively pumped into the sieve tube by the activity of the companion cells. This increase in sugar concentration in the sieve tube results in water being drawn in from the surrounding tissues by osmosis. This movement of water into the sieve tube forces the sap to flow along the tube. The sugar is pumped out at the sink end of the system for storage or use. Water is recycled to the xylem. This pressure flow mechanism keeps the sap flowing through the phloem. 24 Figure 15: Pressure flow in a sieve tube. Storage Water, carbohydrates and other materials (e.g. latex) may be stored in the stem. For example, water is stored in the stems of cacti and other succulent plants, latex in stems of several species (i.e. rubber in Hueva sp.), and carbohydrates in the form of sugar (sugar cane) or starch (English potato is a tuber or swollen underground stem). In addition, hormones and proteins may also be stored in stems. Types of Stems There are many types of stems. For convenience, they can be grouped as below: Herbaceous dicotyledons stems: these stems only have primary growth. Examples include beans, tomatoes, kau kau, and pumpkin. Woody stems: These stems have both primary (from the apical meristem) and secondary (from the vascular cambium) growth. All trees, shrubs, and woody vines are in this category. All woody species are dicots. Woody herbs: these are dicots, which are essentially herbaceous, but have a small degree of secondary growth. Examples are many member's of the mint family. Monocotyledon stems: all monocots grow through primary growth only and are herbaceous. Palm trees, which may appear to be woody, also have only primary growth but they are able to grow tall due to sclerenchyma cells in their trunk, and other complex and not well understand mechanisms. Notice that palm trees do not branch. 25 Modified stems: there are many types of unusual stems. These are stems that have been modified to fulfil specific functions and are therefore often associated with specific habitats. For example, many of the plants that evolved in deserts have stems modified Succulent Stems: the thick succulent stems of cacti are an example of this type of stem. The tubers of the English potato are stems, which are modified to store starch and the 'eyes' of these potatoes are actually buds. Bulbs, such as onions, are stems (with modified leaves) that store water, minerals, and carbohydrates. Plants with bulbs can overwinter in the temperate zone, or help a plant survive through the dry season in deserts and savannas. Stems with Primary Growth Carefully examine Figure 16 below and note that the arrangement of the vascular bundles is different in monocots and dicots. In herbaceous dicots with primary growth only, the vascular bundles are normally arranged in a circle around a central pith. The pith is composed of parenchyma cells. Between the xylem and phloem a distinct cambium (meristematic area) is present. In monocots, on the other hand, there is no central pith, and the vascular bundles are randomly scattered. In addition, there is no obvious cambium-area. This can be seen in Figure 16. The epidermis may have trichomes (glandular hairs) as well as stomates for gas exchange. When the stem is green it means that chlorophyll is present in parenchyma cells and that the stem is a site of photosynthesis. Although the main site for photosynthesis is the leaf, many herbaceous stems also carry out this important energy producing process. Stems with Secondary Growth Secondary growth is the production of new cells from the vascular cambium. The vascular cambium occurs in all woody stems. It is a single layer of meristematic cells between the xylem and phloem and forms a complete circle in the stem. However because a single layer of cells is often difficult to distinguish, the vascular cambium and two or three layers of cells on each side are often called the vascular cambial zone. Epidermis Vascular bundles Cortex FIGURE 16: Transverse section of a monocotyledon stem with vascular bundles arranged in a complex manner throughout the ground tissue. 26 When a seed of a woody dicot germinates, the first growth is primary, i.e. from the apical meristem. If you look carefully at a vascular bundle at this stage, you can see that there is an area of meristematic cells between the xylem and phloem. As the plant grows this zone of cells divide and produce cells that link the cambial zones of the vascular bundles into a complete circle. This circle of meristematic cells is the vascular cambium. As the plant continues to grow from the apical meristem (primary growth), growth from the vascular cambium (secondary growth) adds girth to the stem. The vascular cambium produces phloem to the outside and xylem to the inside. This process is illustrated in Figure 17. As the stem grows taller (primary growth) and wider (secondary growth), the epidermis and cortex are replaced by a corky layer, the periderm. This is derived from yet another group of meristematic cells, the cork cambium. Cork cells contain a fatty substance called suberin in their cell walls, which is highly impermeable to water. Commercial cork comes from the bark of an oak tree, Quercus subefin. Trees can grow for many years and we can see the results of this growth by examining a cross section of a tree trunk (see Figure 18). At the centre the heartwood and sapwood are made up of secondary xylem. Heartwood is the oldest and no longer functions in water transport. Its lignified cells support the tree and often contain colourful resins that protect from insect and fungal attack; they also provide us with the wood we use. Sapwood, on the other hand, still functions in the movement of water and minerals. Each new layer of secondary growth has a larger circumference and enables the xylem to transport a greater volume of water and minerals to the larger tree canopy. The outer bark layer of a tree consists of all tissues external to the vascular cambium. Lenticels are found on the outer part of the bark. These are pores that function in gas exchange. Figure 18: Anatomy of a tree trunk. 27 Figure 17: Stages in the secondary growth of a stem. Activity 5 9. List several functions that stems perform. 10. Indicate whether the following statements are true of monocot stems (M), dicot stems (D) or both monocot and dicot stems (M/D) _______________ a. Vascular bundles scattered throughout the stem _______________ b. Vascular bundles possess a vascular cambium _______________ c. Stem has well-defined pith _______________ d. Stem does not increase in diameter by a large amount _______________ e. Stem covered with an epidermis. 28 Leaves Leaves are the major site of photosynthesis, gas exchange and transpiration. As with other parts of the plant, the morphology of the leaf reflects its major functions and physiology. In addition, leaf morphology reflects different environments. Just as monocot and dicot stems and roots differ, so do monocot and dicot leaves. Dicot Leaves The dicot leaf usually consists of a blade and a small stem-like structure, called the petiole. The leaf is attached to the stem by the petiole. The point of attachment is the node. In some species, a pair of stipules occurs at the base of the petioles. Leaves are arranged on the stem either opposite each other or alternate to each other. Leaves may be either simple or compound (see Figure 18). A simple leaf consists of one blade, whereas in a compound leaf the blade is broken into several leaflets. Axillary buds form in the axil of the leaves; i.e. at the base of the petiole, where it joins the stem. Figure 18: Simple and compound leaves. The internal structure of a dicot leaf is shown in cross-section in Figure 19. Starting from the top, there is the upper epidermis (note the layer of cuticle). The cells just below the epidermis are called palisade parenchyma (due to their elongated shape), and below this you can see the spongy mesophyll, which is another form of parenchyma. Note the black dots in the palisade layer(s) and the mesophyll cells. These represent chloroplasts and it is in these cells that photosynthesis occurs. Also, note the spaces between the mesophyll cells. This is to allow free movement of gases between cells and in and out of the leaf through the stomata. There is a vein, or vascular bundle, in the mesophyll area. A form of parenchyma called the bundle sheath surrounds the vascular tissue (xylem and phloem). These cells enclose and protect the vascular tissue from the surrounding environment. The bottom most layer of cells is the lower epidermis. Like the upper epidermis it is covered with a cuticle, which protects against water loss and physical injury. But, here we see several stomates. The stomates themselves are pores and their opening and closing is regulated by the two guard cells that surround it. Although some plants have stomates on both the upper and lower epidermis, in most plants the stomates are usually found only on the lower epidermis. Why is this? The upper epidermis faces the sun and is exposed to wind, whereas the lower epidermis is shaded by the leaf itself and somewhat protected from the wind. Therefore, there will be less evaporation and less water loss from stomates located on the bottom of the leaf 29 than those located on top. Figure 9 shows a stomate and the guard cells. Notice the chloroplasts in the guard cells. The opening and dosing of stomata is due to the degree of turgor pressure. When the guard cells are turgid the stomata open. When water leaves the vacuole in the guard cells, then those cells collapse and the stomate close. Figure 19: Distribution of primary tissues and arrangement of cell in a leaf. Monocot Leaves Monocot leaves normally consist of a leaf blade and leaf sheath. Like dicot leaves, monocot leaves may also be either simple or compound. Most (but not all) monocot leaves have parallel venation. The internal structure of a monocot leaf is similar to a dicot leaf. The main difference is the lack of palisade parenchyma. In most cases, the stomata are on the underside of the leaf. Modified Leaves Many leaves are modified to perform functions other than those discussed above. We often find that these modifications are correlated with stresses of difficult environments. For example, the leaves of plants growing in arid, dry environments are often thick and succulent and store water. However, in extremely arid conditions such as we find in deserts, plants may not have leaves at all. The thorns of cacti are actually modified leaves, which help shade the stem. Photosynthesis occurs on the green stem. Kalanchoe is another genus of plants with succulent leaves. These species are often called ‘mother of millions' because new plants are produced through mitosis at the edges of the leaves. These new plantlets are clones and genetically identical to the mother plant. 30 Other examples of leaf modification can be seen in carnivorous plants. Carnivorous plants often grow in nitrogen poor soil. Through a variety of methods these plants trap insects. They then release enzymes that digest the insects, allowing the plants to absorb the nitrogen once stored in the insect body. Some carnivorous plants trap insects through leaves modified into pitchers, and others have special glands to which the insect sticks. The leaves of Drosera, the 'Venus fly trap' actually snap closed on small flies, opening only after the fly is digested. Activity 6 11. What are the two main functions of leaves? 12. Match a numbered leaf part with each lettered description. _______________ a. Cylinderical cells just below 1. epidermis upper epidermis 2. xylem _______________ b. Leaf cells containing 3. spongy mesophyll chloroplasts 4. petiole _______________ c. Cell layer containing 5. parenchyma stomata 6. cuticle _______________ d. Cells making up a 7. collenchyma bundle sheath 8. palisade mesohyll _______________ e. A leaf vein is made up 9. phloem principally of these two 10. mesohyll kinds of tissue. Differeneces between monocotyledon and dicotyledon plants Flowering plants (Angiosperms) are divided into two main groups, the monocotyledons (monocots) and dicotyledons (dicots). The cotyledon is the first leaf to emerge from the seed. Monocots have one and dicots have two. The diagramatic representation below summarises the characteristics that separate these two groups and make them distinct. Although many of these characters are difficult to see in the field, the sheathing growth habit of monocots and the branching habit of dicots, make it easy to distinguish monocots from dicots. Figure 20 summarises the most conspicuous differences between monocot and dicot plants. 31 Figure 20: General differences between monocot and dicot plants Glossary Some more new terms introduced in this Unit are listed below. Also remember to keep your dictionary beside you so that you can look any words that you might be unfamiliar to you. Also make sure that you add any additional words to your glossary as you read through these notes. Angiosperm A flowering plant. Generally characterized by embryos with two cotyledons, net-veined leaves, and floral parts arranged in fours, fives, or multiples of these. Bark All tissues external to the vascular cambium in older woody stems. Bud Undeveloped shoot, mainly meristematic. Casparian strip In roots, a waxy, impermeable band in abutting cell walls of endodermis or exodermis where uptake of water and minerals is controlled. Cohesion-tension Idea that evaporation from plants exerts tension in xylem, which theory pulls columns of water molecules that are hydrogen-bonded to one another upward from roots to leaves. Collenchyma A type of simple plant tissue that imparts flexible support during primary growth, as in lengthening stems. Companion cell Specialized parenchyma cell that helps load sugars into sieve tubes in phloem. 32 Cork Component of bark, produced by divisions of cork cambium; its cells have thickened walls. Cork cambium Type of lateral meristem; replaces epidermis with cork on woody plant parts. Cotyledon Seed leaf. Part of a plant embryo. Two form in dicot seeds, one forms in monocot seeds. Cuticle Covering layer of plant surfaces, a layer of waxes and cutin. Endodermis Cell layer around a root's vascular cylinder; helps control water and solute uptake. Epidermis Outermost tissue layer of plants (and all animals) Exodermis Cylindrical sheet of cells just inside the root epidermis of most flowering plants; helps control uptake of water and solutes. Fibrous root system All the lateral branchings of adventitious roots that formed on a young stem. Flower Reproductive shoot of an angiosperm. Growth ring One of the alternating bands of early and late wood in trees showing extensive secondary growth. Guard cell One of a pair of specialized parenchyma cells that define a stoma (one of the tiny openings across leaf or stem epidermis). Heartwood Dry core tissue of older stems and roots; functions in support, storage of metabolic wastes. Leaf Modified plant shoot that intercepts sunlight energy and functions as a photosynthetic factory. Meristem Region of dividing cells in plants; some of their descendants give rise to mature tissues. Mesophyll A type of photosynthetic parenchyma. Monocot Monocotyledon; flowering plant with one cotyledon in seeds, floral parts usually in threes (or multiples of three), and often parallel veined leaves. Mutualism Symbiotic interaction that benefits both partners. Mycorrhiza, A mutualistic interaction between a fungus and root. plural mycorrhizae 33 Nitrogen fixation Conversion of nitrogen gas to forms that plants can take up from soil. Parenchyma Type of simple tissue that makes up the bulk of a plant; has roles in photosynthesis, storage, secretion and other tasks. Phloem A complex tissue that conducts sugars, other solutes through a vascular plant. Pressure flow Organic compounds flow through phloem in response to pressure theory and concentration gradients between source regions (e.g., leaves) and sinks (e.g., regions where the compounds are being used or stored). Root Plant part, typically belowground, that absorbs water and dissolved minerals, anchors aboveground parts, and often stores food. Root hair Thin extension of a root epidermal cell; collectively, root hairs greatly increase the surface area for absorbing water and dissolved ions. Root nodule Localized swelling on a root that contains mutualistic nitrogen-fixing bacteria. Sapwood Functioning secondary xylem of an older woody plant. Sclerenchyma Simple plant tissue that supports mature plant parts and often protects seeds. Most of its cells have thick, lignin-impregnated walls. Shoot A stem, leaf, or flower. Sieve tube Conducting tube of phloem. Stoma, A gap between two guard cells in leaf or stem epidermis; allows plural stomata the diffusion of water vapour and gases across the epidermis. Taproot system A primary root together with all of its lateral branchings; typical of dicots. Tracheid One of two types of water-conducting cells in xylem. Translocation Distribution of organic compounds through phloem. Transpiration Evaporative water loss from the aboveground parts of a plant. Turgor pressure Internal fluid pressure exerted against a cell wall when water moves into the cell by osmosis. Vascular bundle A strand-like array of primary xylem and phloem that threads through a plant's ground tissue system. 34 Vascular cambium A type of lateral meristem that gives rise to secondary xylem and phloem, which increases stem or root diameter. Vascular cylinder Arrangement of vascular tissues as a central cylinder inside a root. Vein In plants, a vascular bundle inside a leaf. Vessel member One of two cell types in xylem. Xylem A complex tissue that conducts water and solutes through pipelines of interconnected walls of cells, which are dead at maturity. 35 UNIT 10: PLANT NUTRITION, HORMONES, REPRODUCTION, RESPONSES & DEFENCES. 36 U NI T 1 0 : P L AN T N U T RI TI O N, R E P RO D U CTI O N , H O RM O N E S , RE S P O NS E S AN D D E FE NC E S Objectives After you have completed the work of this unit you should be able to: 1. List the basic nutritional requirements of plants. 2. State two basic characteristics of flowering plants. 3. Explain what is meant by ‘alternation of generations’. 4. Distinguish and state the function of the various parts of a flower. 5. Describe the development of male and female gametes. 6. Define pollination. 7. List some differences between insect and wind pollination flowers. 8. Define fertilisation and describe how the sperm nuclei reaches the egg cell. 9. Describe the structure of the fruit. 10. List the chief agents of fruit and seed dispersal. 11. Draw in detail the life cycle of a typical flowering plant. 12. Explain how temperature and daylength may affect flowering. 13. Define a tropism 14. Outline experimental evidence for the hormonal control of phototropism in coeleoptiles. 15. Describe the role of auxin in positive phototropism in plants. 16. Comment on negative geotropism in roots. 17. Describe geotropism in roots and shoots and state the possible roles of abscisic acid and statocytes. 18. Briefly describe apical dominance. 19. List the functions of the following hormones: gibberillins, cytokinins, abscisic acid, ethylene. 20. Define photoperiodism and distinguish between short-day plants, long-day plants and day-neutral plants. 21. Outline the role of phytochromes in the flowering response. 22. Define and give examples of each of the following: nastic movement, nyctinasty, photonasty, thermonasty and haptonasty. 23. List some of the economic uses of plant hormones. 24. Describe some first line defences used by plants. 25. Discuss how the immune system of plants works. A comment on the use of the Unit notes Read through these Unit notes to gain an overview of what is covered in this section of the course. You should then review the PowerPoint presentations on this topic. You will also undertake further practical activities related to this Unit. These are covered in the Practical Activities. And do remember your glossary building! 37 Introduction This Unit covers plant nutrition, reproduction and plant hormones and defences. Plants, like animals, need various nutrients in order to remain alive, healthy and reproduce. They require carbon dioxide and water in order to produce simple carbohydrates via photosynthesis. In addition a few macronutrients and several micronutrients are also required. Most of these are obtained from the soil and enter the plant via the roots. Lack of these in the soil may slow plant growth or make it more susceptible to disease. Many plants are able to reproduce both asexually and sexually. Asexual reproduction is much more common in plants than in animals. Virtually every part of one plant or another can be involved in asexual reproduction. This mode of reproduction can be advantageous to plants in that it enables rapid coverage of the ground in a suitable environment. However, the genetic uniformity of the offspring can be a problem for example, if a parasite or other pest is able to infect these plants. Sexual reproduction is required to maintain genetic variation within the population. Sexually reproducing plants have alternating haploid and diploid stages in their life cycles. In flowering plants the haploid stage is much reduced. The flower is the organ specialised for sexual reproduction. The male gametes are produced inside the pollen grains formed in the anthers. The female gametes are produced inside structures called the embryo sacs which develop inside the ovules. These are inside the ovaries. When the pollen grains are fully formed, the anthers split open and pollen is transferred to the female part (stigma) of the flower. Many species of plants have mechanisms to ensure that the pollen is transferred to a different individual of the same species, ensuring cross- pollination. Animals such as insects, birds, bats and other small animals along with wind may be the agents bringing about the transfer of pollen from one plant to another. The pollen deposited in the stigma germinates and a pollen tube grows down the style to the ovule. Fertilisation of the egg cell takes place forming diploid zygote that divides to form an embryo plant. The ovule develops as the seed. Plants, like animals, use chemicals to carry information from one place to another within the plant body to control a wide variety of physiological processes. They are not made in endocrine glands but usually manufactured within cells close to the place where they act. For example, auxin is made by cells at the tips of many shoots, and has its effects further down the shoot. Movement is diffusion, active transport or mass flow in the solutions in the phloem or xylem. We generally recognise five groups of plant growth substances: auxins, which stimulate cell elongation in stems, amongst many other processes; gibberellins, promote growth of stems and the germination of seeds; cytokinins promote cell division; abscisic acid inhibits growth and seed germination and ethylene stimulates fruit ripening. Phytochrome is another substance that is found in all green plants and acts as a photoreceptor pigment. It is involved with two events in the plant life cycle – seed germination and the initiation of flowering. Plants must also withstand attack from a large range of pathogens (viruses, bacteria, fungi, nematode worms, and parasitic plants) as well as predation by herbivores such as insects. In their efforts to resist these attacks it is not surprising that plants have developed a complex 38 array of defence mechanisms. They have resistance (R ) genes that trigger a variety of defence responses in the face of avirulence (avr) genes expressed by pathogens. In the case of herbivore attack the response includes the formation of protease inhibitors that interfere with gut digestion in insects and the formation of a range of chemicals that provide protection to other parts of the plant. Plant nutrients Carbon dioxide taken up by the plant and converted via photosynthetic activity into sugars forms the major source of plant nutrition. However plants also require a number of inorganic nutrients. These mineral nutrients are essential chemical elements absorbed from soil in the form of inorganic ions. For example, plants acquire nitrogen mainly in the form of nitrate ions (NO3-). However, mineral nutrients from the soil make only a small contribution to the overall mass of a plant. About 80 - 85% of an herbaceous plant is water. Because water contributes most of the hydrogen ions and some of the oxygen atoms incorporated into organic atoms, one can consider water a nutrient too. Only a small fraction of the water entering a plant contributes to organic molecules since over 90% is lost by transpiration. Most of the water retained by a plant functions as a solvent, provides most of the mass for cell elongation, and helps maintain the form of soft tissues by keeping cells turgid. Figure 1: An overview of plant nutrition. By weight, the bulk of the organic material of a plant is derived not from water or soil minerals, but from the CO2 assimilated from the atmosphere. Of the 15-20% of a herbaceous plant that is not water, about 95% of the dry weight is organic substances and the remaining 5% is inorganic substances. Most of the organic material is carbohydrate, including cellulose in cell walls. Thus, carbon, hydrogen, and oxygen are the most abundant elements in the dry weight of a plant. Because some organic molecules contain nitrogen, sulphur, and phosphorus, these elements are also relatively abundant in plants. Carefully examine Table 1 which shows the essential nutrients of plants and details their functions. We learnt in Unit 2 that animals can suffer from nutrient deficiencies and that these can cause disease. The same applied to plants. The symptoms of a mineral deficiency depend on the function and mobility of the element - for example, magnesium, an ingredient of chlorophyll, causes yellowing of the leaves, or chlorosis when it is deficient in the soil (see Figure 2 ). You will examine the effects of mineral deficiencies on plant growth as part of your practical activities for this Unit. 39 Figure 2: This photograph shows sweet potato leaves on a plant suffering a magnesium deficiency. Table 1: The essential nutrients of plants. The soil environment The texture and chemical composition of soil are major factors determining what kinds of plants can grow well in a particular location. Plants that grow naturally in a certain type of soil are adapted to its mineral content and texture and are able to absorb water and extract essential nutrients from that soil. Plants, in turn, affect the soil. The soil-plant interface is a critical component of the chemical cycles that sustain terrestrial ecosystems. It takes centuries for a soil to become fertile through the breakdown of soil and the accumulation of organic material or humus. However, human mismanagement can destroy soil fertility within just a few years. Soil mismanagement has been a recurring problem in human history. To understand soil conservation, we must begin with the premise that agriculture is unnatural. In natural ecosystems, mineral nutrients are usually recycled by the decomposition of dead organic material. In contrast, when we harvest a crop, essential elements are diverted from the chemical cycles in that location. In general, agriculture depletes minerals in the soil. To grow a ton of wheat, for example, the soil gives up 18.2 kg of nitrogen, 3.6 kg of phosphorus, and 4.1 kg of potassium. The fertility of the soil diminishes unless replaced by fertilizers. In addition, most crops require far more water than the natural vegetation for that area. 40 Nitrogen fixation in the soil It is amazing that plants sometimes suffer nitrogen deficiencies, for the atmosphere is nearly 80% nitrogen. Plants cannot use nitrogen in the form of N2. It must first be converted to ammonium (NH4+) or nitrate (NO3-) by bacterial mediated processes in the soil (see Figure 3). In the short term, the main source of nitrogen is the decomposition of humus by microbes, including ammonifying bacteria. All life on Earth depends on nitrogen fixation, a process performed only by certain bacteria. In the soil, these include several species of free-living bacteria and several others that live in symbiotic relationships with plants. The reduction of N2 to NH3 is a complicated, multi-step process, catalysed by one enzyme complex, nitrogenase: N2 + 8e- + 8H+ + 16ATP → 2NH3 + H2 + 16ADP + 16Pi Nitrogen-fixing bacteria are most abundant in soils rich in organic materials, which provide fuels for cellular respiration that supports this expensive metabolic process. Symbiotic nitrogen fixation Many plant families include species that form symbiotic relationships with nitrogen-fixing bacteria. These plants characteristically have root nodules or swellings in the roots of the plant that contain nitrogen-fixing bacteria of the genus Rhizobia. These nodules provide the plant roots with a built-in source of fixed nitrogen for assimilation into organic compounds. Much of the research on this symbiosis has focused the agriculturally important members of the legume family, including peas, beans, soybeans, peanuts, alfalfa, and clover. Our yar trees (Casuarina spp.) also fix nitrogen by having symbiotic bacteria which is why they are so valued by subsistence gardeners. Figure 4 shows the stages in the formation of a root nodule. Figure 3: The processes of nitrogen fixation by bacteria in the soil. 41 Figure 4: Root nodule formation. Mycorrhizae and plant nutrition Mycorrhizae (“fungus roots”) are modified roots, consisting of symbiotic associations of fungi and roots. These symbioses are mutualistic, benefiting both partners. The fungus benefits from a hospitable environment and a steady supply of sugar donated by the host plant. The fungus increases the surface area for water uptake and selectively absorbs phosphate and other minerals in the soil and supplies them to the plant. The fungi also secrete growth factors that stimulate roots to grow and branch. The fungi can also produce antibiotics that may help protect the plant from pathogenic bacteria and pathogenic fungi in the soil. Almost all plant species produce mycorrhizae. This plant-fungus symbiosis may have been one of the evolutionary adaptations that made it possible for plants to colonize land in the first place. Fossilized roots from some of the earliest land plants include mycorrhizae. Mycorrhizal fungi are more efficient at absorbing minerals than roots, which may have helped nourish pioneering plants, especially in the nutrient poor soils present when terrestrial ecosystems were young. Today, the first plants to become established on nutrient- poor soils are usually heavily colonised with mycorrhizae. Activity 1 Two groups of tomatoes were grown under carefully controlled conditions. One group had humus added to the soil and the other (control) group was without humus. The leaves of the plants grown without humus were yellowish (less green) than those of the plants growing in the humus-enriched soil. The best explanation for this difference is: 42 A. the healthy plants used the food in the decomposing leaves of the humus for energy to make chlorophyll. B. the humus made the soil more loosely packed, so the plant’s roots would grow with less resistance. C. the humus contained minerals such as magnesium and iron needed for the synthesis of chlorophyll. D. the heat released by the decomposing leaves of the humus caused more rapid growth and chlorophyll synthesis. E. the plants absorbed chlorophyll from the humus. 2. Choose three chemical elements and explain: a) how each is assimilated from the environment by the plant; and b) how he element is used by the plant. 3. The atmosphere is full of nitrogen yet it is inaccessible to plants. Why is that so? What solution has evolved in legumes? We now turn to focus our attention on the reproduction and development of flowering plants. Plant reproduction Plants reproduce by both asexual and sexual methods. We are all familiar with many of the methods of asexual reproduction in plants – our ancestors have been planting roap kaukau for hundreds of years. And all of us have also marvelled at the spectacular beauty of flowers. These flowers are the reproductive organs of plants. Successful sexual reproduction in plants requires adaptations to ensure pollination, fertilisation and seed dispersal and germination. We briefly mentioned the structure of the flower in the previous Unit. Let’s examine a typical flower in a little more detail and see what the main parts are (see Figure 5). The flower is a short shoot that carries specialised ‘floral leaves’; these are arranged on a swollen tip of the flower stalk called the pedicel. The swollen tip is called the receptacle. The parts of the flower are arranged in four separate rings or whorls around the receptacle. 43 Figure 5: The structure of the flower. The outer ring is called the calyx and is made up of sepals. In many flowers these are green; in others they are dull in colour and function to protect the other parts of the flower whilst it is still a bud. The next ring is the corolla, made up of petals. The third ring is made up of the male parts and is called the androecium; it contains the stamens. Each stamen has a stalk or filament supporting an anther. Inside the anther the male gametes are formed inside pollen grains. Finally in the centre of the flower are the female parts or carpels. These make up the gynaecium that contains one or more ovaries, each containing one or more ovules. The female gametes develop inside an embryo sac contained in the ovule. Each ovary has a style supporting a stigma that traps the pollen grains. Plant biologists distinguish between complete flowers, those having all four organs, and incomplete flowers, those lacking one or more of the four floral parts. A bisexual flower is equipped with both stamens and carpals. All complete and many incomplete flowers are bisexual. A unisexual flower is missing either stamens (therefore, a carpellate flower) or carpels (therefore, a staminate flower). A monoecious plant has staminate and carpellate flowers at separate locations on the same individual plant. For example, maize and other corn varieties have ears derived from clusters of carpellate flowers, while the tassels consist of staminate flowers. A dioecious species has staminate flowers and carpellate flowers on separate plants. For example, papaya has female carpellate trees that produce the fruit and male staminate trees that produce the pollen. 44 Figure 6: Corn, a monoecious plant and the dioecious papaya plant. In many animal-pollinated flowers the petals are brightly coloured and scented as they have to attract the pollinators. The petals advertise or signal the presence of the flower and it is interesting to note that there is often a close relationship between the colour, markings and chemical scents produced by the flower and the type of pollinating animal. They have coevolved together. Plants pollinated by insects (particularly bees) are often blue or yellow, as these insects are able to see colours of that wavelength. Insects can also see in the ultraviolet range and the flowers may also have markings in this range as well. Insects also have a well-developed sense of smell and insect pollinated flowers are often strongly odorous. We find many tropical flowers are pollinated by birds and these often have bright red flowers. On the other hand, bat pollinated flowers are often white and bloom at night, smell like ripe or rotten fruit and produce much nectar. Many flowers have nectaries that secrete a sugar-rich nectar, again to attract insects or birds for pollination. Some flowering plants depend on wind to disperse pollen and these produce many small flowers with little nectar or odour but large quantities of pollen. The life cycle of angiosperms and other plants is characterized by an alternation of generations, in which haploid (n) and diploid (2n) generations take turns producing each other. The main features of the life cycle are (see Figure 7): - The diploid plant, the sporophyte, produces haploid spores by meiosis. - These spores divide by mitosis, giving rise to multicellular male and female haploid plants - the gametophyte. - The gametophytes produce gametes - sperm and eggs. - Fertilization results in diploid zygotes, which divide by mitosis and form new sporophytes. 45 Figure 7: The life cycle of an angiosperm plant. Development of the gametes in flowering plants The development of the male and female gametes is illustrated in Figure 8. Each anther contains four pollen sacs and within each pollen sac each microspore parent cell undergoes meiosis to produce haploid cells that ten divide many times to produces thousands of microspores. The nucleus in each microspore divides by mitosis giving rise to two haploid nuclei called the tube nucleus and the generative nucleus. These microspores together develop into pollen grains. The pollen grain has a tough, protective wall. The whole pollen sac contains many thousands of pollen grains. The walls of the pollen sacs eventually rupture and release mature pollen grains. When the pollen is deposited on the stigma and germinates, it grows a tube down the style with the tube nucleus leading the way. At this time the generative nucleus, following along behind, divides by mitosis, producing two haploid sperm. Thus, the pollen grain for a short time can be considered an independent little organism, structurally and functionally quite separate from the flower that produced it. The pollen grain is the male gametophyte. Each ovary within a flower contains one to many ovules. Within each ovule is a tissue called the nucellus. The nucellus contains a megaspore parent cell that undergoes meiosis to produce four haploid nuclei. Three of these nuclei die. One, the megaspore divides by mitosis to produce eight haploid nuclei. Three migrate to one end, two remain in the middle and the other three move to the other end of the cell. One of the eight nuclei is larger and forms the egg cell. The two central nuclei are called polar nuclei or collectively the 46 endosperm mother cell. These cells and the cells formed from the other nuclei are collectively called the embryo sac. This is the female gametophyte. Two membranes called integuments almost completely enclose the nucellus except for a tiny hole called the micropyle. The whole ovule is attached by a stalk to the placenta. Pollination begins the process by which the male and female gametophytes are brought together so that their gametes can unite. Pollination occurs when pollen, released from anthers, is carried by wind or animals to the stigma. Each pollen grain produces a pollen tube which grows down into the ovary via the style (see Figure 9). The two nuclei of the pollen grain move into the pollen tube. The generative nucleus divides mitotically into two sperm nuclei. Once the pollen tube enters the micropyle the tube nucleus degenerates and the two sperm nuclei are released into the embryo sac. These two sperm nuclei cause a double fertilisation (characteristic of the Angiosperm plant group): one sperm fuses with the egg cell to produce the zygote which is now diploid. Future mitotic divisions of this will form the embryo plant. The second sperm nucleus fuses with the two polar nuclei to form the triploid endosperm nuclei. The endosperm helps in the nutrition of the developing embryo plant. The other five nuclei in the embryo sac die. The ovule develops into a seed and the entire ovary develops into a fruit containing one or more seeds. Fruits carried by wind or by animals disperse seeds away from the source plant where the seed germinates. Plants have various mechanisms that prevent self-fertilization Some flowers self-fertilize, but most angiosperms have mechanisms that make this difficult. The various barriers that prevent self-fertilization contribute to genetic variety by ensuring that sperm and eggs come from different parents. Dioecious plants cannot self-fertilize because they are unisexual. In plants with bisexual flowers, a variety of mechanisms may prevent self-fertilization. For example, in some species stamens and carpels mature at different times. Alternatively, they may be arranged in such a way that it is unlikely that an animal pollinator could transfer pollen from the anthers to the stigma of the same flower. The most common anti-selfing mechanism is self-incompatibility, the ability of plant to reject its own pollen and that of closely related individuals. If a pollen grain from an anther happens to land on a stigma of a flower on the same plant, a biochemical block prevents the pollen from completing its development and fertilizing an egg. The self-incompatibility systems (see Figure 10) in plants are analogous to the immune response of animals. The key difference is that the animal immune system rejects non-self, but self-incompatibility in plants is a rejection of self. Recognition of “self” pollen is based on genes for self- incompatibility, called S-genes, with as many as 50 different alleles in a single population. If a pollen grain and the carpel’s stigma have matching alleles at the S-locus, then the pollen grain fails to initiate or complete the formation of a pollen tube. Because the pollen grain is haploid, it will be recognized as “self” if its one S-allele matches either of the two S-alleles of the diploid stigma. Although self-incompatibility genes are all referred to as S-loci, such genes have evolved independently in various plant families. As a consequence, the self- recognition blocks pollen tube growth by different molecular mechanisms. In some cases, the block occurs in the pollen grain itself, called gametophytic self-incompatibility. In some species, self-recognition leads to enzymatic destruction of RNA within the rudimentary pollen tube. The RNAases are present in the style of the carpel, but they can enter the pollen 47 tube and attack its RNA only if the pollen is of a “self” type. In other cases, the block is a response by the cells of the carpel’s stigma, called sporophytic self-incompatibility. In some species, self-recognition activates a signal transduction pathway in epidermal cells that prevents germination of the pollen grain. Germination may be prevented when cells of the stigma take up additional water, preventing the stigma from hydrating the relatively dry pollen. Figure 8: The development of the gametes (pollen and egg cell) in an angiosperm plant. 48 Figure 9: Double fertilisation in angiosperm plants. Figure 10: Incompatability mechanisms in flowering plants. Seed survival The length of time that a dormant seed remains viable and capable of germinating varies from a few days to decades or longer. This depends on species and environmental conditions. Most seeds are durable enough to last for a year or two until conditions are favourable for germinating. Thus, the soil has a pool of non-germinated seeds that may have accumulated for several years. This is called a seed bank and is one reason that vegetation reappears so rapidly after a fire, drought, flood, or some other environmental disruption. 49 Asexual reproduction in plants Sexual and asexual reproductions are complementary in the life histories of many plants. Many plants are capable of both sexual and asexual reproduction, and each offers advantages in certain situations. Sex generates variation in a population, an asset in an environment where evolving pathogens and other variables affect survival and reproductive success. Seeds produced by sexual reproduction can disperse to new locations and wait for favourable growing conditions. One advantage of asexual reproduction is that a plant well suited to a particular environment can clone many copies of itself rapidly. Moreover, the offspring of vegetative reproduction are not as fragile as seedlings produced by sexual reproduction. Seeds, the product of sexual recombination, may lie dormant under an extensive clone produced by asexual reproduction. After a major disturbance (fire or drought, for example) kills some or all of the clone, the seeds in the soil can germinate as conditions improve. Because the seedlings will vary in their genetic traits, some plants will succeed in competition for resources and spread themselves as a new clone. Asexual reproduction in flowering plants may involve modified stems, leaves or roots. For example, rhizomes are underground stems that have nodes and leaf traces. Branching or the splitting of the rhizome may result in the development of separate, identical plants. Tubers (e.g. potatoes) are also enlarged underground stems that may also break apart in asexual reproduction. Bulbs are short underground buds to which fleshy leaves are attached; daughter bulbs may form new plants, e.g. lilies and onions. Stolons are horizontal, above-ground stems that have long internodes. Buds on the stolons can form new plants. Some plants also produce new plantlets along leaf margins. Acti