Phytoremediation: Phytoextraction, Phytostabilization & Rhizofiltration PDF
Document Details
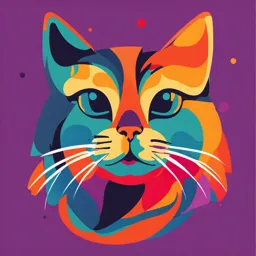
Uploaded by SuitablePelican
Tags
Summary
This document discusses phytoremediation, a method of using plants to remove contaminants from soil and water. It covers different types of phytoremediation, including phytoextraction, phytostabilization, and rhizofiltration, and the factors influencing their success. Specific examples of applying these techniques to metal and organic contaminants are provided.
Full Transcript
# Phytoremediation ## 5.1 Phytoremediation Phytoremediation is a general term used to describe various mechanisms by which living plants alter the chemical composition of the soil matrix in which they are growing. It is the use of green plants to clean up contaminated soils, sediments, or water. T...
# Phytoremediation ## 5.1 Phytoremediation Phytoremediation is a general term used to describe various mechanisms by which living plants alter the chemical composition of the soil matrix in which they are growing. It is the use of green plants to clean up contaminated soils, sediments, or water. The word "phytoremediation" is derived from the Greek prefix Phyto meaning "plant" and the Latin word remidium - meaning "to correct or remove an evil". The "evil" could be anthropogenic (man-made) contaminants such as organic solvents, heavy metals, pesticides or radionuclides. Phytoremediation is an innovative technology that utilizes the natural properties of plants in engineered systems to remediate hazardous waste sites. Phytoremediation takes advantage of plants' nutrient utilization process to take in water and nutrients through roots, transpire water through leaves and act as a transformation system to metabolize organic compounds, such as oil and pesticides or they may absorb and bioaccumulate toxic trace elements including heavy metals. In some cases, plants contain 1,000 times more metals than the soil in which they grow. Heavy metals are closely related to the elements plants use for growth. Phytoremediation has several advantages which include - the cost of phytoremediation is much less than traditional in-situ and ex-situ processes and plants can be easily monitored to ensure proper growth. Valuable metals can be reclaimed and reused through phytoremediation; the least destructive method of remediation because it utilizes natural organisms and preserves the natural state of the environment. Phytoremediation, in general is dependent on several factors: - Plants must produce sufficient biomass while accumulating high concentrations of metals. In some cases, an increased biomass will lower the total concentration of the metal in the plant tissue, but allow for a later amount of metal to be accumulated overall. ## Phytoremediation - Plants which are metal accumulating should be responsive to agricultural practices that allow repeated planting and harvesting of the metal-rich tissues. It is preferable to have the metal accumulated in the shoots as opposed to the roots. The metal in the shoot can be cut from the plant and removed which is manageable on a small scale, but impractical on a large scale. If the metals are concentrated in the roots, the entire plant needs to be removed. - The availability of metals in the soil for plant uptake is another limitation for successful phytoremediation. Phytoremediation methods offer significant potential for certain applications and permit a much larger site to be restored than would generally be possible using more traditional remediation technologies. Plant species are selected for phytoremediation based on the potential to transpire ground water, the degradative enzymes they produce, growth rates and yield, root-zone depth and ability to bioaccumulate contaminants. A large range of species from different plant groups can be used, ranging from pteridophyte forms, to angiospers like sunflowers and poplar trees which employ a number of mechanisms to remove pollutants. There are over 400 different species considered suitable for use as phytoremediators. Phytoremediation is the use of vegetation for in-situ treatment of contaminated soils, sediments and water. It is best applied at sites with shallow contamination or organics, nutrient or metal pollutants that are amenable to one of five applications: Phytotransformation, rhizosphere bioremediation, phytostabilization, phytoextraction, and rhizofiltration. ## 5.2 Metal Phytoremediation The natural ability of certain plant species for the remediation of sites contaminated with metals involves: - Phytoextractions - Phytostabilization - Rhizofiltration. ## 5.2.1 Phytoextraction Phytoextraction technologies use hyper accumulating plants to transport metals from the soil and concentrate them into the roots and above ground shoots that can be harvested. A plant containing more than 0.1% of Ni, Co, Cu, Cr or one percent of Zn in its leaves on a dry weight basis is called a hyper-accumulator, regardless of the concentration of metals in the soil. Almost all metal hyper-accumulating species known today were discovered on metal-rich soils, either natural or artificial, often growing in communities with metal excluders. Actually, almost all metal-hyper accumulating plants are endemic to such soils, suggesting that hyper accumulating is an important ecophysiological adaptation to metal stress and one of the manifestations of resistance to metals. The majority of hyper-accumulating species discovered so far are restricted to a few specific geographical locations. Phytoextraction has been proven effective at a brown field's site in Trenton, New Jersey for remediation of lead-contaminated shallow soils. Nickel hyper accumulations are found in New Caledonia, the Philippines, Brazil and Cuba. Nickel and zinc hyper-accumulation are found in southern and central Europe and Asia. The use of trees can result in extraction of significant amounts of metal because of their high biomass production. The use of trees in phytoremediation requires long-term treatment and may create additional environmental concerns about falling leaves. When leaves containing metals fall or blow away, recirculation off-site by wind transport or leaching can occur. Some grasses accumulate surprisingly high levels of metals in their shoots without exhibiting toxic effects. However, their low biomass production results in relatively low yield of metals. Genetic breeding of hyper-accumulating plants that produce relatively large amounts of biomass could make the extraction process highly effective. For phytoextraction to be effective one needs vigorously growing plants (7 tons dry matter/acre-yr), an easily harvestable above ground and a plant that accumulates large amounts of metals (~1000 mg/kg) in above ground biomass. To achieve clean-up within three to five years, the plant must accumulate about ten times the level in soil, for example - if the level in soil is 500 mg/kg, then the concentration in the plant must be almost 5000 mg/kg to clean up the soil in a few years. Some sites have metals that are bioavailable while other does not. Generally, cadmium, nickel, zinc, arsenic and copper are relatively bio-available while lead, chromium and uranium are not taken up and translocated to the harvestable biomass. Plants which accumulate nickel, cobalt, copper, manganese, lead, zinc and selenium have been reported in the literature. Zinc and boron are phytotoxic to some plants at levels above 200 mg/kg in soil. Addition of EDTA (0.5 to 10 mg EDTA /kg soil) has greatly enhanced the bioavailability of lead, but the enhancement must be weighed against the increased probability of lead migration to ground water. Mathematical modeling of water movement and metals transport may be required to further understand the mechanism of phytoextraction. ## 5.2.2 Phytostabilization Phytostabilization uses plants to limit the mobility and bioavailability of metals in soils. Ideally, phytostabilizing plants should be able to tolerate high levels of metals and to immobilize them in the soil by eruption precipitation, complexation or the reduction of metal values. Phytostabilizing plants also should exhibit low levels of accumulation of metals in shoots to eliminate the possibility that residues in harvested shoot might become hazardous wastes. In addition to stabilizing the metal present in the soil, phytostabilizing plants also can stabilize the soil matrix to minimize erosion and migration of sediment. Since most sites contaminated with metals lack established vegetation, metal-tolerant plants are used to vegetate such sites to prevent erosion and leaching. The varieties of grasses commercially made available to phytostabilizantion are: - Agrosits tenuis, CV parys for copper wastes - Agrosas tenuis, CV coginam for acid lead and zinc wastes - Festuca rubra, CV merlin for calcareous lead and zinc wastes. However, this approach is containment rather than a remediation technology. Phytostabilization is a potentially effective remediation strategy for hydrophobic chemicals and metals at contaminated sites where removal or treatment is not practical. The study conducted at a mine tailings superfund site in South Dakota and an abandoned smelter in Kansas indicates arsenic phytostabilization upto 1,000 mg/kg and lesser amounts of cadmium at site (i) and phytostabilization of zinc and lead was 2,00,000 mg/kg each at site (ii). The goal at each site was to stabilize soils and to decrease vertical migration of leachate groundwater - using deep-rooted hybrid poplar trees. At the South Dakota site, the trees died due to harsh climatic conditions, deer browse and possible toxic stress. At the Kansas site, survival of the trees has been greater than 50% in the third year and the effort more successful later on. Sites with high concentrations of metals are difficult to phytostabilize due to soil toxicity, but it is inexpensive to excavate and treatment or disposal. Soil amendments such as phosphate, lime N/P/K, and organic matter (sewage sludge, compost, aged manure, straw, leaves, etc.) are usually required. Treatability and toxicity studies in small pots in a greenhouse are recommended for phytostabilization. ## 5.2.3 Rhizofiltration Rhizofiltration is the absorption into or the adsorption or precipitation onto plant roots of contaminants present in the soil water. The prevalent difference between two previous approaches is that rhizofiltration is typically used to deal with contamination in the water rather than within the soil. Rhizofiltration uses plant roots to absorb, concentrate, and precipitate metals from wastewater, which may include leachate from soil. Rhizofiltration uses terrestrial plants instead of aquatic plants because the terrestrial plants develop much larger, fibrous root systems covered with root hairs that have extremely large surface areas. This variation of phytoremediation uses plants that remove metals by eruption, which does not involve biological processes. Another type of rhizofiltration, which is more fully developed, involves construction of wetlands or reed beds for the treatment of contaminated waste water or leachate. The technology is cost effective for the treatment of large volumes of wastewater that have low concentration of metals. ## 5.3 Organic Phytoremediation Organic chemicals that are commonly encountered as environmental pollutants are pesticides, hydrocarbons, solvents, lubricants and oil contaminants. These pollutants are not especially mobile, tend to adhere closely to the soil particles themselves and are generally localized within 2 meters of the surface. The mechanism of organic phytoremediation involves phytotransformation, phytovolatilization and rhizosphere bioremediation. ## 5.3.1 Phytotransformation Phytotransformation is sometimes known by the alternative name of phytodegradation. It refers to the uptake of organic and nutrient contaminants from soil and ground water and the subsequent transformation by plants. Phytotransformation depends on the direct uptake of contaminants from soil water and the accumulation of metabolites in plant tissues. It involves the biological breakdown of contaminants either internally, having first taken up by the plants, or externally, using enzymes secreted by them. Hence, the complex organic molecules of the pollutants are subject to biodegradation into simpler substances and incorporated into the plant tissues. For environmental application, it is important that the metabolites which accumulate in vegetation be non-toxic or at least significantly less toxic than the parent compound. Potential applications include phytotransformation of petrochemical sites and storage areas, ammunition wastes, fuel spills, chlorinated solvents, landfill leachates (including BOD, COD, fertilizers and pesticides). The direct uptake of chemicals into the plant through roots depends on the uptake efficiency, transportation rate and the concentration of chemical in soil water. Uptake efficiency, in turn, depends on physio-chemical properties, chemical speciation and the plant itself. Transpiration is a key variable that determines the rate of chemical uptake for a given phytoremediation design; it depends on the plant type, leaf area, nutrients, soil moisture, temperature, wind conditions and relative humidity. ## 5.3.2 Phytovolatilization Another form of phytotransformation is phytovolatilization whereby volatile chemicals or their metabolic products are released into the atmosphere through plant transportation. Phytovolatilization generally relies on the transpiration pull of fast-growing trees, which accelerates the uptake of the contaminants in groundwater solution, which are then released through the leaves. Thus the pollutants are removed from the soil, often being transformed within the plant before being voided to the atmosphere. Many chemicals that are recalcitrant in the subsurface environment react rapidly in the atmosphere with hydroxyl radicals, an oxidant formed in the photochemical cycle. The transfer of contaminants from the soil or groundwater to the atmosphere is not as desirable as in-situ degradation, but it may be preferable to prolonged exposure in the soil environment and the risk of groundwater contamination. Once an organic chemical is translocated, the plant may store the chemical and its fragments into new plant structures via lignification (covalent bonding of chemical or its fragments into lignin of the plants), or it can volatilize, metabolize, or mineralize the chemical completely to carbon dioxide and water. Chlorinated aliphatic compounds such as trichloro ethylene (TCE) have been reported to be mineralized to CO₂ and less toxic aerobic metabolites (trichloro ethanol, trichloroacetic acid and dichloroacetic acid). These products are consistent with those found in the human liver for TCE destruction by cytochrome P450, which is an abundant enzyme in plants as well as humans. Thus, plants are sometimes viewed as "Green Livers" in terms of their enzyme biochemistry. Nitroreductase and laccase enzymes in plants can break down ammunition wastes such as TNT (2,4,6 trinitro toluene) and they may incorporate the broken ring structures into new plant material or organic detritus that becomes a path of sediment organic matter. Detoxification mechanisms may transform the parent chemical to non-phytotoxic metabolites that are stored in plant tissues. A thorough understanding of pathways and end products of enzymatic processes will simplify toxicity investigations in-situ phytoremediation. For example, in one experiment, a genetically modified variety of the yellow poplar, Tiriodendran tulipifera has been used and engineered by the introduction of mercuric reductase gene (mer A). This study confirms the ability to tolerate higher mercury concentration and to convert the metal's ionic form to the elemental and allows the plant to withstand contaminated conditions, remove the pollutant from the soil and volatilize it. In a number of studies, poplar species have been shown to be able to volatilize around 90% of the trichloroethylene they take up. The choice of a poplar species for this application is interesting since they have been found useful in similar roles elsewhere for phytovolatilization. ## 5.3.3 Rhizosphere Bioremediation Rhizosphere bioremediation is also known as phytostimulation or plant assisted bioremediation. Phytoremediation of the rhizosphere increases soil organic carbon, bacteria and mycorrhizal fungi- all factors that encourage degradation of organic chemicals in soil. Experiments showed that the number of beneficial bacteria increased in the root zone of hybrid poplar trees relative to unplanned reference site. Denitrifiers, Pseudomonas sp. BTEX degrading organisms, and general heretrophs were enhanced. Also, plants may release exudates to the soil environment that help to stimulate the degradation of organic chemicals by inducing enzyme systems of existing bacterial populations stimulating growth of new species that are able to degrade the wastes, and/or increasing soluble substrate concentration of all microorganisms. Leakage of sugars, alcohols, and acid from the plant and root turnover can amount to 10 to 20% of plant photosynthesis on an annual basis. Researchers have categorized the molecular weight distribution of organic exudates from root systems of hybrid poplar trees. Exudates include short chain organic acids, phenolics and small concentrations of high molecular weight compounds (enzymes and proteins). Research at the U.S. Environmental Protection Agency (EPA) Laboratory in Athens, Georgia has examined five plant enzyme systems in sediments and soils, i.e. dehalogenase, nitroreductase, peroxidase, laccase and nitrilase. - Dehalogenase enzymes are important in dechlorination reactions of chlorinated hydrocarbons - Nitroreductase is needed in the first step for degradation of nitroaromatics - Laccase enzyme serves to break aromatic ring structures in organic contaminants - Peroxidase and nitrilase are important in oxidation reactions. These enzymes are active in rhizosphere soils in close proximity to the root (1 mm) for transformation of organic contaminants that would occur in the absence of the plant. The addition of plant root systems creates an ecology in soils that is suitable for bioremediation. The EPA studies on TNT breakdown have demonstrated that plants like hornwort increase, soil water pH 3 to 7 and sorb high concentrations of metals that would usually inhibit bacteria, while the plants remain healthy and viable. Overall, plants and their root systems can accommodate mixed wastes (organic and metals) and other harsh conditions. It is interesting to note that fungi growing in symbiotic association with the plant have to degrade organic carbon that could not be transformed solely by bacteria. In addition to soluble exudates, the rapid decay of fine root biomass can become an important addition of organic carbon to soils which serves to retard organic chemical transport. Microbial materialization of atrazine is directly related to the fraction of organic carbon in the soil. Microbial assemblages are abundant in the rhizosphere and typical communities may comprise 5x105 bacteria, 9x105 actinomycetes and 2x10³ fungi per gram of air dried soil; bacteria live in colonies that cover as much as 10% of the root surface area. EPA studies have demonstrated the importance of biodegradation in rhizosphere. ## 5.4 Bioremediation Needs – R&D ### 1. Bioremediation needs Bioremediation is a natural process alternative to such methods as incineration, catalytic destruction, the use of adsorbents and the physical removal and subsequent destruction of pollutants. By integrating proper utilization of natural or genetically modified microbial capabilities with appropriate engineering designs to provide suitable growth environments, bioremediation can be successful of the field. The major factors responsible for lack of successful utilization of bioremediation technology i.e. a sufficient knowledge base to accurately predict pollutant degradation rates and fates and sites designated as field research centers for bioremediation and research and technology demonstrations. Laboratory and microcosm studies have documented the potential use of microorganisms for bioremediation. Successful application of bioremediation techniques must address both the heterogeneous nature of many contaminated waste sites and the complexity of using living organisms on genetically modified organisms in the actual environmental field. Bioremediation studies have documented the further research needs for successful application of this technology. Bioremediation will not advance as rapidly as an alternative to other treatment methods unless biotechnologists get involved in the early stages of R&D for field application. Based on the documented research in the area of bioremediation, the following needs more R&D efforts in making this a successful applied technology. ## 2. General Approaches Scientists and engineers reviewed the current state of bioremediation and suggested approaches for resolving the problems in making bioremediation a successful applied technology. - Bioremediation assessment and implementation need more integrated cross-discipline effort. - To evaluate or employ bioremediation there is a need for initial site characterization of site heterogeneity. - To assess biotreatability, widely accepted methods and criteria need to be established. - Bioremediation application needs adequate identification of factors responsible for limiting degradation rates. - The full range of treatment options need to be considered before ensuring bioremediation. - Application of bioremediation techniques from laboratory to field need the establishment of more adequate information and enhanced modeling principles. - Techniques for monitoring progress and utilizing mass balance concepts in the field need to be further developed. - Bioremediation needs an accessible, expanded and well-documented database. ## 3. Research Programme Areas In 1992, EPA issued a report recommending five key research programme areas that would provide a balanced transition of bioremediation research from the laboratory bench to technology application in the field. The following areas are documented: - Improve site characterization technology. - Create a "Bioremediation Field Initiative" to expand field experiments and collect and disseminate bioremediation performance data. - Develop new approaches to performance evaluation in order to better determine the extent and rate of clean-up. - Research process to identify microbial capability of biotransformation and develop method for delivery. - Develop better models of bioremediation through exploitation of field results to predict and guide future applications. ## 4. Microorganism Behavior in Bioremediation Process The National Research Council, 1993 reports "In-situ bioremediation" recommended the factors needed to be studied as to understand how microorganisms behave in the field, the difficulties associated with stimulating microbial growth in the field and making the containment bioavailable to the microbial community. These factors include to: - Understand microbial processes in nature such as anaerobic versus aerobic reactions and how they are interrelated within a micro niche - Examine the effect of stimulating materials for increased microbial growth such as gas sparging - Promote more efficient contact between the contaminant and the microorganism through high-pressure fracturing of the subsurface matrix or solubilization of the contaminants via steam injection, addition of surfactants, or through improved dispersal methods - Evaluate protocols for the range of chemical contaminants and site characteristics in the field and document the loss of these contaminants from the field - Develop innovation site characterization techniques that are rapid, reliable and inexpensive - Improve mathematical models to link the understanding of chemical, physical and biological activities occurring in the field - Demonstrate that bioremediation is working in the field at enough rates in multiple locations to ensure that clean-up goals are met. ## 5. Phytoremediation – A Viable Technology In 1993, the American Chemical Society sponsored a symposium exuded "Bioremediation through Rhizosphere Technology". The findings of the papers published documented that the rhizosphere provides a complex and dynamic microenvironment where bacteria and fungi associated with plant roots can degrade, mineralize and stabilize toxicants, thus offering a potentially important treatment technology for bioremediation of numerous classes of hazardous wastes in the environment. In 1994, the Department of Energy held a workshop to discuss the current status and the basic applied research needs for phytoremediation. These include: - Understanding the fundamental biochemical, physiological and ecological interactions in the rhizosphere that facilitate the successful use of vegetation to remediate chemically contaminated soils. - Understanding the mechanisms of uptake, transport and accumulation of pollutants. - Evaluation of the plant species which are hyperaccumulaters of metals and other pollutants. - Understanding the mechanism of interactions occurring in the rhizosphere between plant root system and surrounding biota. - Implementing field evaluations and validation of phytoremediation technologies. ## 6. Role of Microorganisms in Bioremediation In 1994, a workshop of the European Community and the U.S. Government was held to focus on the potential of microorganisms to solve environmental problems. Three areas of research were discussed: aerobic bio-transformation of pollutants, biodegradability testing using microcosm and mesocosm approaches, and tailor-made microorganisms for the treatment of toxic wastes. The research needs identified are : - Examine bioremedial catalytic systems of microorganisms that have not previously been well studied - Focus on the diverse metabolic pathways exhibited by microorganisms when grown in the absence of oxygen - Explore use of combined aerobic/anaerobic or anaerobic/aerobic systems for biodegradation of pollutants - Assess the bioavailability of contaminants and catalysis in the non aqueous phase of contaminant biotransformation in multiple-phase systems - Develop biological-based methods for the containment, control and monitoring of microbial populations in reactors and field sites - Further explore inoculation for bioremediation in contained and uncontained systems. ## 7. Bioremediation Research Priorities In 1995, the National Science and Technology Council's Biotechnology Research Subcommittee reported that several priorities for research in bioremediation are needed to further advance the field. These research priorities are: - Develop an understanding of the structure of microbial communities and their dynamics in response to normal environmental variation and novel anthropogenic stresses - Develop an understanding of the biochemical mechanisms, including enzymatic pathways involved in aerobic and particularly anaerobic degradation of pollutants - Extend the understanding of microbial genetics as a basis for enhancing the capabilities of microorganisms to degrade pollutants - As a standard practice, conduct microcosm/mesocosm studies of new bioremediation techniques to determine a cost-effective manner, whether they are likely to work in the field, and establish dedicated sites where long-term field research on bioremediation technologies may be conducted - Develop, test and evaluate innovative biotechnologies such as biosensors for monitoring bioremediation in-situ, models for the biological processes at work in bioremediation, and reliable, uniform methods for assessing the efficacy of bioremediation technologies. ## Bioremediation processes can often provide clean, natural and cost effective methods for treatment of pollutants. Yet, bioremediation is often not even considered as an alternative technology by field scientists or technologists. Bioremediation is an innovative and unproven technology till date. A systematic long-term integrated research, innovation and improvement and establishment of standard methods/technologies will enhance the potential of bioremediation as and a major "Environmental Technology". ## References ### Phytoremediation - Adhya, T.K. et.al. (1995), Leaching of Hexachlorocyclohexane Isomers in a Flooded Field Unplanted or Planted to Rice. Water, Air, and Soil Pollut., 81:305-313. - Ahuja, L.R. et.al. (1996), a Field-Test of Root-Zone Water-Quality Model: Pesticide and Bromide Behavior., Pest. Sci.. 48:101-108. - Anderson, T.A. (1992), Comparative Plant Uptake and Microbial Degradation of Trichloroethylene in the Rhizosphere of Five Plant Species - Implications for Bioremediation of Contaminated Surface Soils. University of Tennessee, Ph.D Dissertation. - Anderson, T.A., Guthrie, E.A. and Walton, B.T. (1993) Bioremediation, Environ. Sci. Technol, 27:2630-2636 - Anderson, T.A., and Walton, B.T. (1995), Comparative Fate of 14 Trichloroethylene in the Root Zone of the Plants from a Former Solvent Disposal Site. Environ. Toxicol. Chem. 14:2041-2047. - Aprill, .W.S., Sims, R.C. (1990), Evaluation of the Use of Prairie Grass for Cleaning Up PAH Treatment in Soil, Chemosphere, 20:253-265. - Banuelos, G.S. et.al. (1996), Accumulation of selenium by different plant-species grown under increasing sodium and calcium-chloride salinity, Plant and Soil, 183:49-59. - Barkovskli, A.L., Boullant, M.L. and Balanbreu, J. (1994), Polyphenolic Compounds Respired by Microorganisms in: Bioremediation Through Rhizosphere Technology, American Chemical Society. - Barona, A. and Romero F. (1996) Study of metal accumulation in wild plant using principal component analysis and ionic impulsions, Toxicol. and Environ. Chem., 54:75-86. - Bedell, G.W. (1992), Phytoremediation, The World & I, 12,260-263. - Berti, W.R., and Jacobs, L.W. (1996) Chemistry and Phytotoxicity of Soil Trade Elements from Repeated Sewage Applications. J. Environ. Qual. 25:1025-1032. - Blaylock, M.J., Muhr, E., Page, D., Montes, G., Vasudev, D., Kapulnik, Y. (1996) Phytoremediation of lead contaminated soil at a Brownfield site in New Jersey. Proceedings of Am. chem.. Soc., Birmingham, AL., Sept 9-11. - Blum, U. et.al. (1991), Phenolic acid Content of soils from wheat-no. till, wheat-conventional till, and fallow conventional till soybean cropping systems, J. Chem. Ecology, 17:1045-1068. - Bowen, G.B. and Rovira, A.D. (1976), Microbial colonization of plant roots. Ann. Rev. Phytopathology, 14:121-144. - Briggs, G.G., Bromilow, R.H. and Evans, A.A. (1982), Relationships between lipophicity and root uptake and translocation of non-ionized chemicals by barley. Pestic. Sci., 13:495-504. - Brock, T.D. and Madigan, M.T. (1991), Biology of Microorganisms, Prentice-Hall, Inc., Englewood Cliffs, NJ. - Brown, K.S. (1995) The Green Clean: The emerging field of phytoremediation takes root. BioScience, 45:579-582.