Food Packaging Materials - BATANGAS STATE UNIVERSITY
Document Details
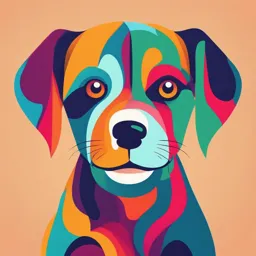
Uploaded by CherishedForest
Batangas State University
Tags
Related
- Anthocyanin-Based pH-Sensitive Smart Packaging Films for Monitoring Food Freshness (Journal Article PDF)
- Food Packaging and Labelling Introduction to Food Packaging PDF
- Food Packaging PDF
- Food Packaging PDF
- STM3163 Food Packaging PDF
- Sustainable Edible Packaging Systems Based on Active Compounds from Food (PDF)
Summary
This document, from BATANGAS STATE UNIVERSITY, explores different food packaging materials, focusing on edible films and coatings, as well as bio-based alternatives. It discusses various types of edible films, their advantages, and challenges, primarily from a chemical engineering perspective.
Full Transcript
Republic of the Philippines BATANGAS STATE UNIVERSITY The National Engineering University Alangilan Campus Golden Country Homes, Alangilan Batangas City, Batangas, Philippines 4200...
Republic of the Philippines BATANGAS STATE UNIVERSITY The National Engineering University Alangilan Campus Golden Country Homes, Alangilan Batangas City, Batangas, Philippines 4200 Tel Nos.: (+63 43) 425-0139 local 2121 / 2221 E-mail Address: [email protected] | Website Address: http://www.batstate-u.edu.ph College of Engineering - Department of Chemical Engineering I. Introduction Food-packaging materials have traditionally been based on nonrenewable materials, except for paper-based products. However, in the early 20th century, packaging materials were made from biologically-derived resources, such as inks, dyes, paints, medicines, chemicals, clothing, and plastics. Petroleum-derived chemicals replaced these resources during the 20th century. Today, there is increasing attention to sustainability and the replacement of nonrenewable resources with renewable sources, such as plant-derived products and by-products from fermentation. Biobased packaging materials are primarily derived from annually renewable sources, excluding paper-based materials. II. Edible Films and Coatings used to extend the shelf life of fresh food products and protect them from harmful environmental effects. The concept of using edible films or coatings to extend the shelf life of fresh food products is not new, but their advantages over traditional synthetic polymeric packaging materials include being consumed with the packaged product, contributing to environmental pollution reduction, enhancing the organoleptic properties of packaged foods, supplementing the nutritional value of foods, individual packaging of small portions of food, applying inside heterogeneous foods at the interfaces between different layers of components, functioning as carriers for antimicrobial and antioxidant agents, microencapsulating food flavoring and leavening agents, and using edible films in multilayer food packaging materials together with nonedible films. The advantages of edible films over traditional synthetic polymeric packaging: 1. Films can be consumed with packaged products, reducing residual packaging. 2. They contribute to environmental pollution reduction by degrading more readily than synthetic polymeric materials. 3. Films can enhance the organoleptic properties of packaged foods with incorporated flavorings, colorings, and sweeteners. 4. They can supplement the nutritional value of foods, especially proteins-based films. 5. Films can be used for individual packaging of small portions of food, such as peas, beans, nuts, and strawberries. 6. They can be applied inside heterogeneous foods to prevent moisture and solute migration. 7. Films can function as carriers for antimicrobial and antioxidant agents and control the diffusion rate of preservative substances. 8. They can be used for microencapsulation of food flavoring and leavening agents. 9. They can be used in multilayer food packaging materials with nonedible films. A. Polysaccharide-Based Coatings - Polysaccharides and their derivatives, such as alginate, pectin, carrageenan, starch, starch hydrolysates, and cellulose derivatives, have been used as edible films or coatings. These polymers have limited moisture-barrier properties due to their hydrophilic nature. However, Leading Innovations, Transforming Lives, Building the Nation Republic of the Philippines BATANGAS STATE UNIVERSITY The National Engineering University Alangilan Campus Golden Country Homes, Alangilan Batangas City, Batangas, Philippines 4200 Tel Nos.: (+63 43) 425-0139 local 2121 / 2221 E-mail Address: [email protected] | Website Address: http://www.batstate-u.edu.ph College of Engineering - Department of Chemical Engineering certain high-moisture gelatinous coatings can retard moisture loss from coated foods by acting as sacrificing agents. - Amylose, a linear fraction of starch, forms strong, free-standing films, while hydroxypropylated derivatives of high-amylase starch are poor moisture barriers but good oil and moisture barriers at lower relative humidity. - Alginates, extracted from brown seaweeds, are impervious to oils and greases but have high water vapor permeabilities. - Carrageenan, a complex mixture of at least five distinct polymers based on galactose, forms strong gels with melting points above the initial gelation temperature. - Agar, derived from various red seaweeds, forms strong gels with melting points above the initial gelation temperature. - Dextrans, microbial gums, and cellulose ethers are used to preserve food flavor, color, and freshness during refrigeration or frozen storage. B. Lipid-Based Coatings Lipid-based coatings are derived from lipids (fats) and have been used as protective barriers in food packaging. These coatings are effective because they prevent the movement of water, making them good at preserving moisture in food products. However, they aren't ideal for providing structural strength because lipids don't form coherent, strong films. Fat-based Coatings: These coatings are less resistant to moisture loss compared to more advanced synthetic coatings but are commonly used for fresh fruits and vegetables. One downside of wax-fat coatings is that they can give foods a greasy surface or a rancid taste over time. Glycerides: Mono-, di-, and triglycerides (types of fats) are used for their water and oxygen barrier properties. Acetylated glycerides are particularly effective but can suffer from brittleness and oxidation. Acetylation, the chemical process used to make these glycerides, makes them more resistant to water vapor than other materials but may cause issues during refrigeration or storage. C. Protein-Based Coatings Protein-based coatings are made from animal and plant proteins. Some common proteins used in these coatings include: Collagen: Collagen is a protein found in animals, especially used in sausage casings and coatings. Gelatin: Derived from collagen through partial hydrolysis, gelatin is also widely used for its film-forming ability. Milk Proteins (Casein): These proteins form good barriers and provide flexibility, making them valuable in food packaging. Cereal Proteins (Zein): Derived from corn, these proteins are used in edible coatings for their good barrier properties against oxygen and moisture. However, one challenge with protein-based coatings is that they are susceptible to proteolytic enzymes, which can break down the proteins, especially under high humidity conditions. Additionally, the use of certain proteins in coatings needs clear labeling because some individuals may have food allergies. Leading Innovations, Transforming Lives, Building the Nation Republic of the Philippines BATANGAS STATE UNIVERSITY The National Engineering University Alangilan Campus Golden Country Homes, Alangilan Batangas City, Batangas, Philippines 4200 Tel Nos.: (+63 43) 425-0139 local 2121 / 2221 E-mail Address: [email protected] | Website Address: http://www.batstate-u.edu.ph College of Engineering - Department of Chemical Engineering D. Multicomponent Films Multicomponent or composite films are made by combining different materials to achieve a balance between structural strength and protective properties. These films are typically made up of: - A lipid layer for moisture resistance. - A polysaccharide or protein layer for additional structural integrity and functionality. Key Points: Plasticizers such as sucrose, glycerol, sorbitol, and propylene glycol are added to improve the flexibility and durability of edible films. Multicomponent films may use lipids to act as moisture barriers while proteins or polysaccharides form a structural matrix. The films can be created using two basic techniques: - Coating: The lipid layer is coated onto a dried base layer to form a bilayer or laminate. - Emulsion: Lipid and film-forming solutions are emulsified together to create a uniform mixture. These films are often compared with synthetic films like LDPE (Low-Density Polyethylene), but their water and oxygen barrier properties vary depending on the materials used. Combining edible waxes or fatty acids with these films can significantly improve their water vapor and oxygen barrier properties. III. Biobased Packaging Materials Biobased packaging materials have been divided into three types, reflecting their historical development; First-generation materials consist of synthetic polymers such as LDPE with 5 to 20% starch fillers. Although these materials disintegrate or biofragment into smaller molecules when composted, they do not biodegrade. Second-generation materials consist of a mixture of synthetic polymers and 40 to 75% gelatinized starch, and some of these materials are fully biodegradable. Third-generation materials consist of fully biobased and biodegradable materials. Biodegradability Biodegradable polymers constitute a loosely defined family of polymers that are designed to degrade through the action of living organisms. Biodegradability and compostability are different concepts. Although biodegradation may take place as a result of the disposal of a material in landfill, composting usually requires a pretreatment of municipal solid waste (MSW) to remove bulky noncompostable items and inorganic waste Leading Innovations, Transforming Lives, Building the Nation Republic of the Philippines BATANGAS STATE UNIVERSITY The National Engineering University Alangilan Campus Golden Country Homes, Alangilan Batangas City, Batangas, Philippines 4200 Tel Nos.: (+63 43) 425-0139 local 2121 / 2221 E-mail Address: [email protected] | Website Address: http://www.batstate-u.edu.ph College of Engineering - Department of Chemical Engineering A. First Generation The first generation of commercial, biodegradable polymers consists of 5 to 20% starch together with LDPE and pro-oxidative and auto-oxidative additives. They are mixed during the extrusion process, resulting in the starch granules being uniformly dispersed in the LDPE matrix without chemical interaction. Higher levels of starch (up to 43%) can be incorporated if the surface of the starch granules are silylated to increase their hydrophobicity. Claims that these materials were biodegradable (it takes 3 to 5 years for them to degrade into dust). B. Second Generation The second generation consists of gelatinized starch (40 to 75%) and LDPE with the addition of hydrophilic copolymers such as EAA. PVOH and VA, which act as compatibility agents. Comples degradation of the starch takes 40 days, and degradation of the entire fifth ility of years. C. Third Generation These materials are entirely biobased and categorized into three main groups according to how they are produced: 1. Polymers directly extracted from biomass – These are natural polymers that can be taken directly from renewable sources. 2. Polymers produced by classical chemical synthesis from biomass monomers – These involve more processed materials, synthesized from renewable feedstocks. 3. Polymers produced directly by natural or genetically modified organisms – These polymers are produced through biological processes, such as fermentation or genetic engineering. The schematic diagram presented in Figure 3.2 illustrates the different types of biobased polymers based on these categories: Category 1: Polymers directly extracted from biomass Polysaccharides: Includes starches (from potato, corn, wheat, rice), celluloses (cotton, wood), and gums (e.g., guar gum, alginate, pectin). Proteins: Derived from animal and plant sources, such as casein and whey (animal), and zein and soy (plant). Lipids: Includes cross-linked triglycerides like collagen/gelatin and gluten. These are mostly from marine and agricultural sources. Polysaccharides such as cellulose, starch, chitin, and proteins like casein, whey, collagen, and soy are typical examples. Cellulose-based paper and board are the most widely used food-packaging materials in this category and have been used successfully for decades due to their ease of processing and availability. Category 2: Polymers synthesized from bioderived monomers Examples include polylactates, which are polyesters produced by synthesizing biobased monomers. Leading Innovations, Transforming Lives, Building the Nation Republic of the Philippines BATANGAS STATE UNIVERSITY The National Engineering University Alangilan Campus Golden Country Homes, Alangilan Batangas City, Batangas, Philippines 4200 Tel Nos.: (+63 43) 425-0139 local 2121 / 2221 E-mail Address: [email protected] | Website Address: http://www.batstate-u.edu.ph College of Engineering - Department of Chemical Engineering This category focuses on polymers synthesized from biobased monomers through processes such as polymerization or chemical synthesis. Polylactic acid (PLA) is highlighted as the most commercially viable polymer in this group. PLA is a biodegradable, linear aliphatic polyester made from lactic acid, which can be produced from the fermentation of crops like corn or wheat. PLA is popular in food packaging, used in products like thermoformed packaging for bakery goods, films for wrapping, and biodegradable food-service containers. It offers key benefits such as a 50% reduction in fossil fuel use and a significant reduction in CO₂ emissions compared to petroleum-based plastics. PLA is fully compostable, making it ideal for environmentally friendly applications in food packaging, although production costs and technical factors still present challenges. Category 3: Polymers produced by organisms Includes PHAs (polyhydroxyalkanoates) and bacterial celluloses, which are synthesized by microorganisms either naturally or through genetic modification. This group is dominated by polyhydroxyalkanoates (PHAs), which are microbial polyesters produced by certain bacteria such as Alcaligenes eutrophus. Poly(hydroxybutyrate) (PHB) is the most common PHA. It is biodegradable, biocompatible, and produced intracellularly as an energy reserve by bacteria. PHAs are linear aliphatic polyesters that can be produced from sugars or co-polymers of different hydroxyalkanoic acids, offering versatility in their properties. By adjusting the ratio of the components, the resulting material can mimic common plastics like PP (polypropylene) or LDPE (low-density polyethylene). Despite their desirable properties, the high cost of production and the need for improved efficiency in synthesis have limited large-scale use. However, with advances in genetic modification and sustainable production, PHAs hold promise for future biodegradable plastic production. PHAs are biodegradable in various environments, including soil, compost, and marine ecosystems, where microorganisms break them down into their components. D. Properties of Biobased Packaging Materials 1. Barrier Properties Under the conditions of high Humidity biobased packaging materials have poor barrier properties. In order to achieve the desired barrier properties necessary for the packaging of food, biobased materials (Paper and Recycled Fiber Lines) are necessary to be coated with synthetic polymers. ○ The WVTRs (Water Vapor Transmission Rate) of starch-based films are four to six times greater than those of conventional films. ○ Poly Lactic Acid (PLA) has WVTRs four times higher that conventional films ○ Polyhydroxyalkanoates (PHA) has WTVRs similar to those of Low-Density Polyethylene (LDPE) The gas-barrier properties of many biobased materials depend on the ambient humidity; however, two notable exceptions are PLA and PHA. ○ Polyhydroxybutyrate (PHB) has better O2-barrier properties than Polyethylene Terephthalate (PET) and Leading Innovations, Transforming Lives, Building the Nation Republic of the Philippines BATANGAS STATE UNIVERSITY The National Engineering University Alangilan Campus Golden Country Homes, Alangilan Batangas City, Batangas, Philippines 4200 Tel Nos.: (+63 43) 425-0139 local 2121 / 2221 E-mail Address: [email protected] | Website Address: http://www.batstate-u.edu.ph College of Engineering - Department of Chemical Engineering Polypropylene (PP), and adequate fat-odor and odor-barrier properties for applications with short shelf life products. It is possible to improve the barrier properties of biobased materials using plasma deposition of SiOx or applying nanocomposites from natural polymers and modified clays 2. Mechanical Properties The mechanical properties of most biobased materials are similar to synthetic polymers, although some (such as PLA) are sensitive to heat and humidity. Orientation of PLA improves mechanical strength and heat stability, and varying crystallinity and MW results in films ranging from soft and elastic to stiff and high strength. PLA has a Tg of 60°C, a melt temperature of 155°C, a heat seal initiation temperature of 80°C and mechanical properties similar to those of PET. In general, PHB resembles isotactic PP with respect to mechanical behavior. E. Food Packaging Applications 1. Limited Use of Biobased Packaging: a. Despite extensive research and development, the application of biobased materials in food packaging remains limited. 2. Applications of PLA: a. One-Way Beverage Cups: i. Major use of PLA for one-way beverage cups in food-service applications. ii. Used at recent Olympic Games as either 100% PLA cups or paper cups coated with PLA to promote an environmentally friendly image. b. Niche Markets: i. Small niche markets for PLA trays and films identified in Europe, particularly for organically grown food. c. Short-Term Use of Biobased Materials: i. Suggested use for short shelf-life foods stored at chill temperatures due to biodegradability. ii. Potential applications include: 1. Fast-food packaging for salads. 2. Egg cartons. 3. Fresh or minimally processed fruits and vegetables. 4. Dairy products like yogurt. 5. Organically grown foods. d. COO Permeability: i. The high COO permeability ratio of certain biobased materials suggests potential for packaging respiring foods, such as fruits and vegetables. Leading Innovations, Transforming Lives, Building the Nation Republic of the Philippines BATANGAS STATE UNIVERSITY The National Engineering University Alangilan Campus Golden Country Homes, Alangilan Batangas City, Batangas, Philippines 4200 Tel Nos.: (+63 43) 425-0139 local 2121 / 2221 E-mail Address: [email protected] | Website Address: http://www.batstate-u.edu.ph College of Engineering - Department of Chemical Engineering IV. Environmental Aspects Development of Biobased Packaging: Predicated on the belief that biobased materials have lower environmental impacts than petroleum-based counterparts. Without this advantage, there is less incentive for industry adoption. Questioned the utility of biodegradable and biobased plastics based on energy and environmental analyses. Key statements: ○ "Biodegradability has a hidden cost": Biological breakdown releases CO₂ and CH₄, heat-trapping greenhouse gases. ○ Energy needed for PHA production is high: 2.39 pounds of fossil resources vs. 2.26 pounds for polystyrene. ○ Concluded that substituting renewable resources loses benefits due to energy requirements. Based on a single bioplastic technology (PHA), which is not yet commercial or optimized for scale. Analyzed only energy usage for production, ignoring the entire life cycle. B. Life Cycle Assessment studies New detailed Life Cycle Assessment (LCA) studies present a different picture. Of 20 reviewed studies: ○ 7 focused on starch polymers. ○ 5 on PHA. ○ 2 on PLA. ○ 3 on other biobased polymers and composites. C. Current Environmental Benefits: Starch polymers perform best environmentally, with variations among types. PLA shows smaller environmental benefits, with limited data (energy and CO₂). PHA has minimal advantages compared to conventional polymers (data limited to energy use). Production methods, scale, and waste management critically influence overall environmental balance. Leading Innovations, Transforming Lives, Building the Nation Permeability of Thermoplastic Polymer Introduction Packages composed of thermoplastic polymers are permeable to small molecules, including gases, water vapour, organic vapours, and other low MW substances, to various degrees, in contrast to materials constructed of glass or metal. Food packaging uses a variety of thermoplastic polymers. They offer varying degrees of barrier or protection against the transmission of low molecular weight compounds. The increasing usage of rigid plastics and polymer films for food packaging has made it more crucial than ever to understand the solution and transport behaviour of low molecular weight compounds in polymeric materials. The integrity of packages including their seals and closures as well as the permeability of the packing materials are key factors in protecting food from gas and vapour exchange with the environment. Gases and vapours can move through polymeric materials via two different processes: 1. a pore effect, in which vapours and gases enter the materials through tiny cracks, pores, and pinholes. 2. This is known as the solubility-diffusion effect, when gases and vapours dissolve at one surface of the polymer, diffuse through it under a concentration gradient, and evaporate at the opposite surface. True permeability refers to this "solution-diffusion" (or "activated diffusion") mechanism. Theory of Permeability Scottish chemist Thomas Graham made the first known observation of a gas passing through a membrane in 1826 when he wrote about CO2 passing through a polymeric membrane. 1831 saw J.K. The play rubber balloon was invented by American physician Mitchell, who also discovered that different gases caused his balloons to burst at varying rates. By drawing comparisons between Newton's law for momentum transfer, Ohm's rule for electrical conduction, Fourier's equation for heat conduction, and Ohm's law for heat conduction, German scientist Adolf Fick's law of mass diffusion was proposed in 1855. Fick was interested in quantifying the transport of oxygen in blood. Permeation Through Pores Although it is common to find pores in the region of plastic films next to the heat seal, pores in food packaging made of plastic are often frowned upon. In addition to facilitating mass transfer between a package's interior and the surrounding environment, pores, microholes, and cracks also allow microbes to enter the package; certain bacteria have been shown to pass through holes as small as 0.4 um in diameter. Polymer/Permeant Relationships The permeability ratio between two gases in noncondensable gases is essentially constant and unaffected by the type of polymer. As a general guide, Table 4.3 provides average values for a variety of polymers. It is evident that CO2 penetrates around six times faster than O2 and 24 times faster than N2, while O2 penetrates roughly four times faster than N2. The actual diffusion, solubility, and permeability coefficients for LDPE are provided in Table 4.4. In this particular instance, CO2 permeates at a rate that is almost four times faster than O2 and nearly thirteen times faster than N2, indicating that the values in Table 4.3 are averages among a number of distinct polymers. For a particular polymer, the selectivity ratios are not constant; rather, they rise with decreasing temperature. For instance, Tolle27 observed that when the temperature rose from 0 to 20°C, the CO2:O2 for LDPE varied from 5.08 to 3.45. According to Others8, as the temperature rose from 5 to 40°C, the ratio for PVC films varied from 6.0 to 4.0. The largest of the three gas molecules, CO2, has the highest permeability coefficient, which may seem odd. As may be predicted given its relative size, it has a low diffusion coefficient; but, as Table 4.4 for LDPE shows, its solubility coefficient S is significantly higher than that of the other gases, giving it the highest permeability coefficient. Values for one gas can be estimated using the appropriate values from Table 4.3 if a value for one gas is known. To a lesser extent, the D and S values likewise correspond to these relative values. Factors Affecting The Diffusion And Solubility Coefficients A. Pressure In the case of the permanent gases, the permeability coefficient is independent of the pressure of the diffusing gas. This is also true in many instances for other gases and vapors, provided there is no marked interaction between the polymer and the diffusing material. However, where there is strong interaction, the permeability coefficient is found to be pressure dependent and, in general, it increases as the pressure increases. This is due to an increase in the diffusion coefficient D promoted by the plasticizing effect of the sorbed permeant, and an increase in the solubility coefficient S. B. Sorption The term sorption is generally used to describe the initial penetration and dispersal of permeant molecules into the polymer matrix and includes both adsorption and absorption as well as cluster formation. Sorption behavior has been classified on the basis of the relative strengths of the interactions between the permeant molecules and the polymer, or between the permeant molecules themselves within the polymer. C. Temperature The permeability coefficient of a specific polymer-permeant system may increase or decrease with increases in temperature depending on the relative effect of temperature on the solubility and diffusion coefficients of the system. Generally, the solubility coefficient increases with increasing temperature for gases and decreases for vapors, and the diffusion coefficient increases with temperature for both gases and vapors. For this reason, permeability coefficients of different polymers determined at one temperature may not be in the same relative order at other temperatures. Transmission Rate Of considerable interest in many food packaging applications is the transmission rate of various organic compounds such as flavors, aromas, odors and solvents through polymers. This is particularly so where the package contents have to be protected against contamination from foreign odors, or where there is a requirement to ensure that volatile flavoring materials are not lost from the food. The significant off-flavors found in some food products may result from the packaging material itself, or may permeate through the packaging material from the outside environment. In other situations, foods may contain highly desirable but volatile flavor compounds whose loss from the packaged food would detract from its quality. In both situations, suitable tests must be undertaken to select materials that have the desired odor barrier properties. Migration Migration is the release of substances initially present in the package into the food. Plastics contain numerous low MW substances that can migrate including monomers, oligomers and various additives such as plasticizers and antioxidants. These substances diffuse through the material until they reach the inside surface of the package where they are partially transferred to the headspace or dissolved in the food. This may result in a loss of food quality due to flavor or color changes, or it may make the food toxic without perceptibly altering the organoleptic properties of the food. For this latter reason, regulations have been promulgated that establish maximum migration limits for substances found in plastics which are used to package food. Measurement of Permeability A. Gas Permeability There are many methods for measuring gas permeability; four major methods will be considered here. For a complete understanding of the principles behind permeability measurements, it is important that the meaning of two terms which constantly use total and partial pressure of gases in a mixture is clearly appreciated. In a constant volume, the total pressure exerted by the gases present is the sum of the partial pressures of each of the gases, a discovery made by English scientist John Dalton in 1801 and known as Dalton's law. The partial pressure of any one of the constituent gases is the pressure that would result if that particular gas occupied the same volume by itself. That is, each of the gases of a gas mixture behaves independently of the others. The rate of permeation of a specific gas through a polymeric material is a function of the partial pressure differential of that gas across the material and not of the total pressure difference between the two sides. 1. Pressure Increase Method The ASTM manometric method for measuring gas transmission rates and permeabilities of flat films is designated D 1434. Test gas (normally at 1 atm) is introduced on one side of the flat film or sheet, which is supported with a filter paper and sealed with an O-ring. The pressure in the receiving chamber is measured with an open-ended mercury manometer. Provided that the pressure on the high-pressure side remains much larger than that on the low-pressure side, the pressure difference remains essentially constant. Through equations relating the geometry of the cell with the rate of pressure rise in the manometer, the gas transmission rate can be calculated. 2. Volume Increase Method In the ASTM standard volumetric method (also designated D 1434), the change in volume (at constant pressure), due to the permeation of gas through the film, is measured. Variable volume permeation cells are used for rapid measurement of relatively high steady-state permeation rates. Although the volume increase method is generally simpler to implement, it is less sensitive than the pressure increase method. Volumetric methods are used relatively infrequently compared with the use of the pressure increase or concentration increase methods. 3. Concentration Increase Method In the ASTM D 3985 method (also known as the quasi-isostatic coulometric method because the total pressure on both sides is approximately equal), a partial pressure difference across the film with respect to the test gas is created without a difference in total pressure, thus obviating the need for rigid support of the film. A partial pressure difference is maintained by sweeping one side continuously with the test gas, and maintaining an inert gas on the other side into which the test gas diffuses. The concentration of the diffusing gas can be measured by chemical analysis, gas chromatography, thermal conductivity or special electrodes. A variation of this method (ASTM F 1307) can be used to determine the O2 transmission rate through dry packages. 4. Detector Film Method A relatively new method for measuring permeabilities of films requires little equipment and is both rapid and accurate. 14 The basis of the method is a plastic detector film impregnated with a reagent that is sensitive to the gas being measured. The film has an absorption spectrum that changes as the gas or vapor is absorbed, and is thus suitable for spectrophotometric monitoring. The detector film is sealed between two pieces of test film in a simple cell so that the permeation rate of the penetrant gas or vapor can be readily measured. The detector film can measure much less than the minimum detectable quantity of O2 determined by most other methods, and therefore permits the use of either smaller film samples or more rapid permeability determinations. B. Water Vapor Permeability The standard method to determine water vapor transmission rates (ASTM E 96) is to place a quantity of desiccant in an aluminum dish, which is covered with a sheet of the material being tested and sealed in position with wax. The dish is then placed in a closely controlled atmosphere (typically either 25±0.5°C and 75 ± 2% RH for temperate conditions, or 38±0.5°C and 90±2% RH for tropical conditions), and the increase in weight noted as a function of time. These methods have several disadvantages, including the length of time needed to make a determination (between 2 and 14 days) and the lower limit of the useful range (about 1gmday for a typical packaging film). A further disadvantage is that, depending on the desiccant, Ap may not remain constant during the test period. In the case of anhydrous CaCl₂, the partial pressure of water vapor in the dish remains below 2% of the vapor pressure of water at the test temperature during the test, whereas in the case of silica gel, the partial pressure of water sorbed on it increases with coverage. WVTR tests on flat sheets of film sealed across aluminum dishes do not always correlate closely with actual performance of the film when made up into complete packages. Therefore, it is often preferable to carry out WVTR tests where the desiccant is contained in a finished package that has been closed and sealed in the conventional manner. C. Permeability Of Organic Compounds Although the permeabilities of permanent gases and water vapor through many packaging materials are well known, there is limited data for the permeation of organic compounds. It was shown earlier in this chapter that, for gases, the permeability coefficient is independent of concentration. Thus, permeability measurements made at high concentrations can be reliably extrapolated to predict permeation rates where there are low concentration gradients across the barrier. However, in the case of many organic compound/plastic package combinations, the permeability coefficient is highly dependent on concentration. This effect occurs because the organic compound interacts with and swells the polymer, increasing the permeation rate. Processing and Converting of Thermoplastic Processing and Converting of Thermoplastic refers to the various techniques and methods used to transform raw thermoplastic materials into functional products. Thermoplastics, renowned for their thermoplasticity, are polymers capable of undergoing repeated cycles of softening and hardening without undergoing significant chemical changes. The process encompasses multiple stages, including extrusion, coextrusion, calendering, coating, laminating, and metallization, and many more. These techniques are instrumental in producing a diverse array of films, sheets, and structures. Thermoplastics, renowned for their versatility, durability, and recyclability, are widely used in industries like packaging, construction, and manufacturing. I. Extrusion It is emphasized as a fundamental method where thermoplastic materials are melted and formed into sheets or films, with screw extrusion being the primary mechanism. This process uses an Archimedean screw to melt and pump plastic through a die, producing films through either the flat film or tubular film methods. The flat film process, or cast film extrusion, involves cooling the molten polymer on chilled rollers, while the tubular film process inflates a tube of polymer into a bubble, producing films with varying properties depending on the polymer and processing conditions. Both methods have distinct advantages, such as the higher optical properties of flat films and the mechanical superiority of tubular films. COEXTRUSION MONOLAYER EXTRUSION A.Monolayer Extrusion Monolayer extrusion is a manufacturing process that extrudes a single type of polymer through a die to create a continuous film. This method is used to produce films with uniform properties and is typically simpler and less expensive than multilayer extrusion. Monolayer extrusion includes the use of only one type of polymer as the feedstock, resulting in a film that has consistent properties throughout its thickness. Additionally, the process is generally less complex than multilayer extrusion, making it more cost-effective, as it often requires lower capital investment and operating costs. B.Coextrusion Builds upon extrusion by combining multiple polymers through several extruders to form multilayer films. These films benefit from the unique properties of each polymer, such as barrier protection or strength, that may not be possible with single-layer films. The document highlights different coextruded structures, such as five-layer or nine-layer films, commonly used in food packaging, providing flexibility and cost-efficiency over traditional laminates. Single Screw Extruder Blown Tubular Film Extrusion II. Calendering It is described as a complementary process to extrusion, primarily used for forming uniform films or sheets by squeezing heated plastic between rollers. It offers greater control over thickness and surface finish compared to extrusion and is widely employed for processing materials like PVC. III. Coating And Laminating These are presented as essential for enhancing the functional properties of films, particularly in food packaging. Coating involves applying liquid or melted material to a substrate, while laminating bonds multiple layers together. A. Coating Processes Extrusion coating, also known as extrusion lamination, emerged in the 1950s with the production of LDPE-coated paperboard for milk cartons, replacing traditional wax coatings. LDPE offers superior strength, seal integrity, and moisture resistance, effectively protecting paperboard from water damage. While any thermoplastic can theoretically be coated onto substrates, lower density polyethylenes are primarily used, with materials like PET also applied in applications such as dual-ovenable trays. To ensure strong adhesion between polyethylene and various substrates, surface pretreatments are essential. Two main methods have been developed: corona discharge and flame treatment. Corona discharge involves applying high voltage to ionize the surface, enhancing adhesion by introducing polar groups. Conversely, flame treatment creates an oxidized layer but requires careful control to prevent film degradation. Additionally, thin primer coatings can improve bonding by ensuring compatibility between the polyethylene and the substrate, preventing deep penetration and ensuring effective adhesion during the extrusion coating process. B. Laminating Processes Methods that join two or more layers by bonding them together are known as laminating processes. Bonding typically occurs through thermal or chemical methods involving adhesives and curing systems. Once the adhesive is properly dried or cured, the coated layer is fused with an uncoated layer using heat or pressure in a nip. Various materials can be laminated together, and the process can continue until the laminate achieves the desired protective qualities. Five types of laminating process includes: 1. Thermal laminating involves joining two layers with an adhesive that is applied to one layer and allowed to cool and dry. The layers are then heated and pressed together between two rollers to create a strong bond. Common adhesives used in this process are polyolefins like EVA, with typical materials including plastic films and heat-seal-coated aluminum foil. 2. Wet bond laminating uses solvent or water-based adhesives and is limited to permeable materials, making it less effective for plastic films. In contrast, dry bonding offers greater versatility, allowing any two materials to be laminated once an appropriate adhesive system is developed. 3. Dry bonding is a versatile method that can laminate any two materials using an appropriate adhesive. While both water-based and solvent-based adhesives can be used, solvent-based adhesives have been limited due to environmental regulations. Complete solvent drying is essential to prevent delamination and contamination. 4. Solventless laminating has become the preferred method in commercial applications due to regulations restricting VOC emissions, using a reactive chemical system to cure the adhesive without releasing solvents. 5. Extrusion laminating is a specialized technique where a hot extruded film is sandwiched between two layers and then cooled, primarily used to create laminates of materials like paper, aluminum foil, and PET with LDPE acting as the bonding agent. IV. Metallization It is introduced as a process where plastic films are coated with a metal layer, typically aluminum, to improve barrier properties and achieve decorative or reflective surfaces. Metallization is commonly used in food packaging, where it provides durable and aesthetically pleasing materials, though it cannot match the barrier properties of traditional foils. V. Orientation Orientation is a process that enhances the strength and durability of polymer films, making them suitable for a wider range of applications. It involves stretching the film to align the molecular chains in a specific direction. This alignment improves properties like tensile strength, impact resistance, flexibility, clarity, stiffness, and toughness. Gas and water vapor permeability can also be reduced, especially in crystalline polymers. However, orientation can negatively affect elongation, tear propagation, and heat sealability. To overcome these challenges, surface coatings or radiation cross-linking can be used. For example, OPP can be coated with a thermoplastic like PVdC copolymer to improve heat sealability. Radiation cross-linking is applied to LDPE to enhance its tensile strength and shrink tension without melting. Orientation techniques are commonly used in the production of films like PET, PA, PVdC copolymers, PP, and LDPE. Crystalline polymers like PP show significant improvements in clarity due to the alignment of their crystalline structure. To prevent shrinkage, films can be annealed, which involves heating and cooling them while maintaining their stretched state. A. Orientation Process Orientation is a method used to strengthen thermoplastic films by stretching them after heating. There are two processes for orientation: 1. Flat Sheet Process: A thick film is stretched in both directions using a system of rollers and a frame that holds the edges of the film. 2. Tubular or Bubble Process: Molten polymer is extruded into a tube, which is stretched by inflating it like a bubble and pulled by rollers at different speeds. Figure Orientation process using a tenter. Figure Orientation process by the tubular or bubble process. The film’s stretching temperature, rate, and quenching (cooling) affect its final properties. Lower temperatures and faster stretching usually improve orientation. Films can shrink when reheated due to the elastic memory of stretched molecules. B. Shrink Films Shrink films are made of materials like polyolefins, PVC, and PVdC. To shrink, most films need temperatures above 100°C (except PVdC, which can shrink in hot water). Key properties of shrink films include: 1. Shrink temperature range: Lower shrink temperatures are easier and cheaper to handle. 2. Degree of shrinkage: Different films shrink between 15-80%, and precise temperature control is needed to manage shrinkage. 3. Shrink tension: This is the force exerted by the film when restrained from shrinking. Higher tension creates tighter packages. Balanced shrinkage is essential for printed films to avoid print distortion. C. Stretch Films Stretch films, introduced in the 1970s, are used for wrapping large items like pallet loads. The film is stretched around the item, with its adhesive properties helping it stick to itself. Common materials include LDPE, LLDPE, PVC, EVA copolymer, and PP. Stretchability varies by film type: LDPE stretches about 30%, while LLDPE can stretch up to 400%. Additives like cling agents, antioxidants, and anti-block agents improve performance. Stretch films relax over time, losing tension, but some retain their stretch better than others. VI. Cross-Linking Cross-linking in crystalline thermoplastics reduces crystallization, leading to softer and less strong polymers. There are three methods for cross-linking polyethylene: radiation, peroxide, and vinyl silane cross-linking. Here, we focus on radiation cross-linking. Radiation cross-linking uses electron beams to alter C-C bonds, allowing films to maintain orientation even at high temperatures. Cross-linked LDPE film is stronger, with better heat resistance, flexibility, and toughness, making it ideal for shrink films. VII. Microperforation Microperforation is used to create breathable films for packaging fresh produce with high respiration rates. There are three main ways to increase film permeability: 1. Inorganic fillers like ceramic powder, which increase gas permeability, though they reduce clarity. 2. Porous patches with membranes, which can be tailored for different products. 3. Perforations or microperforation create small, precise holes (40-200 microns in diameter) to control gas flow. Microperforation is often done using laser technology and is commonly applied to OPP films, though it works on various polymers. VIII. Injection Molding Injection molding is a process for shaping thermoplastic materials into items like yogurt tubs, caps, and PET preforms. The process has three main steps: 1. Feeding and melting plastic granules using a rotating screw. 2. Injecting the melted plastic into a cold mold at high pressure. 3. Cooling and ejecting the solidified part from the mold. It is widely used in mass production due to its efficiency, though it requires expensive molds. Common materials include LDPE, HDPE, PP, and PET, the latter being used for making stretch-blown bottles. IX. Blow Molding Blow molding is a process to produce hollow objects. It was practiced with glass in ancient times, and the basic techniques used by the plastics industry have been derived from those developed by the glass industry. Currently, a wide range of blow molded bottles and containers are produced for use in food packaging. In blow molding, a molten tube of thermoplastic (known as a parison) is surrounded by a cooled mold having the desired shape. A gas (usually air but occasionally N₂) is introduced into the tube, causing the molten mass to expand against the walls of the mold where it solidifies on cooling. The mold is then opened and the bottle or jar ejected. Generally, the process for manufacturing plastic bottles and jars consists of three stages melting the resin, forming the parison and blowing the parison to produce the final shape. The blowing step may take from a few seconds to more than a minute for large shapes; the rate limiting step is cooling of the molded shapes. There are two techniques of plasticizing resin (i.e., making the material flow) and forming the parison: 1. Extrusion, which produces a continuous parison that has to be cut; this is the most common method used. 2. Injection molding, where the parison is formed in one mold and then transferred into another mold for blowing. A. Extrusion Blow Molding Extrusion blow molding (EBM) uses many arrangements for making and forming the parison. In the simplest method, a mold is mounted under an annular die and the parison extruded between the open halves of the mold. When the parison reaches the proper length, the extruder is stopped and the mold closes around the parison. A blow pin mounted inside the die head allows air to enter and blow the parison into the final container shape. The shape of the bottle or jar is defined, but the distribution of material (and thus wall thickness) is less well controlled. The cycle restarts after the part has cooled and the mold open. Because the extrusion of the parison is a continuous process, numerous systems have been developed to use the full capacity of the extruder. One uses more than one mold, moving the filled mold away to cool, while another is moved into position to receive the next section of extruded tube. The molds can be reciprocating ones, or can be mounted on a rotary table. In several food packaging applications (e.g., fresh milk), the bottles are blow molded and filled on-line in a continuous operation. EBM is widely used with the following resins: HDPE, PP, PVC and AN copolymers. The common grades of PET cannot be extrusion blown. A related process is the production of coextruded bottles, where two or more extruders, each handling different plastic materials, produce a multilayer parison with the desired properties. For example, a high barrier, high cost material might be sandwiched between layers of a relatively low cost material to give a bottle with the desired barrier properties at an economical price. Coextruded structures with up to seven layers, of which one or more can be a barrier layer, are common today. B. Injection Blow Molding Injection blow molding (IBM) is a noncontinuous cyclic process shown in Figure 5.8, and most closely resembles the blowing of glass bottles. The parison is formed in one mold and then, while still molten, is transferred to a second mold where blowing with compressed air forms the final shape. After cooling, the mold is opened and the bottle ejected. Several molds must be available if the injection molding machine is to operate near full capacity. The major advantage of IBM over EBM is that the process is virtually scrap-free, the finished parts usually requiring no further trimming, reaming or other finishing steps. In addition, the dimensions of the bottle (including the neck finish) show very little variation from bottle to bottle, and with some materials, improved strength and clarity are obtained due to the effect of a limited degree of biaxial orientation (see Section V). The resins most commonly used for IBM are HDPE, PP, PS, PVC and PET. PET has replaced PVC in many countries, especially in Europe where PVC has a poor image among many consumers. Coinjection blow molding has been developed using two or three injection units working with one mold to produce a preform, which is later blow-formed using compressed air inside a mold to make a bottle or jar. 10 The various component materials are metered into cavities in such an order that the barrier material flows through the main structural material to create a multilayer structure. This process is used to produce five layer retortable containers from three materials, typically PP as a structural layer and EVOH copolymer as a barrier layer with tie layers in between. C. Stretch Blow Molding Stretch blow molding (SBM) is a process where bottles with appreciable orientation in both longitudinal and transverse directions are produced; it is sometimes known as biaxial orientation blow molding. Orientation in the transverse direction only is produced in normal EBM, while appreciable transverse, and some longitudinal orientation, is produced in IBM. True biaxial orientation produces bottles with improved properties including increased tensile and impact strength, improved surface gloss, reduced creep, improved gas and water vapor barrier properties and a reduction in haze in transparent bottles. As a result, lighter weight and lower cost bottles can be produced. To produce a biaxially molded bottle, a preform or parison (produced either by injection molding or extrusion of a continuous tube which is then cut to the required length and closed at one end) is stretched longitudinally under heat and blown into a bottle shape with consequent transverse orientation. The process of SBM is particularly important in the field of carbonated beverage packaging using PET. For best results, the resin molecules must be conditioned, stretched and oriented at just above the T, where the resin can be moved without risk of crystallization. Although PET is the major stretch blown resin, PVC, PP and AN copolymer resins are also stretch blow molded. Two different stretch blow techniques are available for SBM. In the one stage method, parison injection molding, temperature conditioning and blow molding take place in the same machine. This method is commonly used for widemouth jars and bottles with unusual cross-sectional shapes. In the two stage method (also known as the "reheat and blow" method), the parisons are first injection molded in a completely separate stage and stored at ambient temperature until required. They are then reheated to between 90 and 110°C and blown to their final shape. No stretch occurs at the top and bottom of the bottle. Typical stretch ratios for a 2-L PET bottle are 2.3:1 in the axial (longitudinal) direction and 3.9:1 in the hoop (transverse) direction. Often, production of preforms and bottles are physically separated in different facilities, with the SBM often being conducted on-site by the filler or the packaging supplier in a hole-through-the-wall (HTW) operation. For hot fill applications, heat resistant PET bottles are required, since a normal PET SBM bottle cannot be filled much above 65°C without causing bottle shrinkage. Manufacture of a heat resistant bottle is called the "heat set process" and involves increasing the crystallinity to about 30% or more as opposed to 25% in a container produced by the conventional blow molding process. 13 Two methods are used. In the first, a single mold method, the preform is stretch blow molded into a hot mold where the mold halves are 125 to 145°C and the mold base is 90 to 95°C, resulting in the preform being heated to between 100 and 110°C, about 10°C hotter than in the conventional blow molding process. Heat setting takes place as the blown container encounters the surface of the hot mold while being constrained by high pressure against the mold. 13 The container must then be cooled down below its T, before it can be removed from the mold. In the second (dual mold) method, the preform is stretch blow molded into a first mold, followed by reheating in an oven to relieve stresses and shrivel the shape, followed by reblowing in a second mold to produce the final bottle shape. To avoid unsightly bottle appearance as a result of vacuum formation inside the bottle after hot filling, hot fill bottles are molded with sidewall panels and base designs that move inwards as the product cools and ensure that the vacuum-induced forces do not distort the bottle. Production of multilayer coinjection preforms is the most economical way to achieve enhanced properties in a rigid PET container. Multilayer coinjection is the process by which one or more interior layers of material are totally encapsulated by outer virgin PET layers. It is the only technology able to provide any combination of clarity, gas barrier, gas scavenger and recycled PET (RPET) in a single process. 12 molding techniques. Multilayer parisons can be produced by either extrusion or injection Coinjection SBM was a major breakthrough, enabling longer shelf lives for beverages to be achieved. It has been used to produce three-layer (PET-MXD6-PET) and five-layer (PET- RPET-PET-MXD6-PET) bottles, mainly in smaller sizes where the lack of a barrier layer would XI. Foamed Cellular Plastics Foamed or cellular plastics are defined as plastics whose apparent density is decreased substantially by the presence of numerous cells dispersed throughout their mass. The terms foamed plastic, cellular plastic, expanded plastic and plastic foam are used interchangeably. These materials have been used widely since the 1940s, largely due to their desirable properties, which include a high strength to weight ratio, and good insulating and cushioning properties. A wide range of plastic polymers can be foamed, including LDPE, HDPE, PP, EVA, PS and polyurethane. The market is dominated by the latter two polymers, with PS being used in preference to polyurethane for food contact applications. Plastic foams are classified as either flexible or rigid, and may have an open or closed cell structure; in the former, the cells are interconnected, whereas in the latter, most cells are closed and separate. Cell formation is initiated by foaming agents. Physical foaming agents are compounds that change their physical state during cell growth and the most important are volatile liquids (typically aliphatic hydrocarbons and CO₂) with boiling points below 110°C at atmospheric pressure. Chemical foaming agents decompose under heat to at least one gaseous decomposition product, commonly N2. PS foams can be produced by two processes: injection molding and extrusion. With injection molded foam, machines similar to normal injection molding machines are used, except that steam is injected to heat the beads that contain a foaming agent. Extruded PS foam is produced by free expansion of hot PS, blowing agents and additives through the slit orifice of a high L/D ratio extruder to about 40 times the pre-extrusion volume. The amount and type of blowing agent control the density of the foam produced. The major food packaging uses for extruded PS foam sheet are egg cartons and meat and poultry trays. XII. Heat Sealing The heat sealability of a packaging film is one of the most important properties when considering its use, and the integrity of the resultant seal is of paramount importance to the ultimate integrity of the package. A number of factors are involved in determining the quality of a heat seal. They can be conveniently summarized under three headings: Machine factors: dwell or clamp time, temperature and pressure Resin factors: density, MW and additives in the resin Film factors: gauge, style or form (e.g., whether gusseted or not) and treatment for printing All of these factors tend to interact in a complex way. For example, the amount of heat available may be limited by the capacity of the heating elements, by the rate of heat transfer of the sealing bar and its coating or by the type of product being packaged. Increasing the dwell time (i.e., the time during which heat is applied) will increase the heat available, but this may prove to be uneconomical since fewer units will be able to be handled per minute. Heat sealable films are considered to be those films that can be bonded together by the normal application of heat, such as by conductance from a heavy heat-resistant metal bar containing a heating element. Nonheat-sealable films cannot be sealed this way, but they can often be made heat sealable by coating them with heat-sealable coatings. In this way, the two coated surfaces A. Conductance Sealing Conductance (also known as resistance or bar) sealers are the most common type of heat sealers in commercial use and typically consist of two metal jaws (often patterned or embossed to give the seals extra strength), one of which is electrically heated, the temperature being controlled thermostatically. The second or backing jaw is often covered with a resilient material such as rubber to distribute pressure evenly and aid in smoothing out the film in the sealing area. Frequently, the unheated jaw is water cooled, although, in some situations, it may be heated to the same temperature as the first jaw to enable sealing through sheets of film in exactly the same way each time. A simple heat sealer is shown in Figure 5.9. B. Impulse Sealing In the impulse sealing method, the films (usually unsupported materials) are clamped between two cold metal bars and then fused by the effect of a short, powerful electrical impulse; cooling occurs under pressure. Much lighter jaws than for conductance sealers are used. A high current is sent for a short period through a nichrome resistance wire or ribbon covered with PTFE tape. The current heats the wire to the desired temperature, where the temperature is controlled with a transformer. Dwell time of the heating impulse must also be controlled, as must the length of the cooling period. Thus, two timers are often found on impulse sealers, with the second timer controlling the cooling of the resistance wire to allow the film to harden under pressure and prevent deformation of the film in the sealed area. Sometimes, one of the jaws is water cooled to prevent excess heating and promote rapid cooling. If very heavy films are to be sealed, then both jaws may contain resistance wires. To prevent the film from sticking to the heated jaws, it is usual to cover them with a slip sheet such as glass cloth impregnated with PTFE. Generally, the seals produced by impulse sealing are of excellent quality. C. Induction sealing Induction sealing is a noncontact method of heat sealing accomplished by exposing an aluminum foil coated with a thermoplastic adhesive substance to a magnetic field. The field induces eddy currents, which generate precise amounts of localized heat in the aluminum foil, melting the adhesive material without any physical contact between the coil and the package. Modern advances in solid-state technology have made induction heating a remarkably simple and cost-effective sealing method. D. Ultrasonic sealing In this method of sealing (which is similar to high frequency induction heating), a generator feeds a 20 kHz signal into a transducer, which transforms electrical energy into mechanical vibrations of the same frequency. The mechanical energy is converted into heat at the interface, producing an almost instantaneous weld. Little overall heating is produced, thus enabling oriented films to be sealed without any change in dimensions. A conical welding head acts as a focusing tool for the vibrations that pass through the film to an anvil. The pressure, along with temperature and dwell time (expressed in terms of the rate at which the film is fed between the welding head and anvil), are adjusted to obtain optimum seal quality. E. Dielectric Sealing In the dielectric sealing method, dielectric heating is used in which a high frequency current (typically 50 to 80 MHz) is passed through two or more layers of film. The electrodes (usually made of brass) are the top and bottom jaws, where the latter is the ground of the circuit. When the layers of film are in place between the closed jaws, a high frequency current is passed between the jaws, heating and liquefying the films. The pressure the jaws exert on the films helps to bring about thorough fusion and bonding.This method of sealing is used principally with PVC and nylon 6/6 films as they are difficult to heat seal by direct means because they tend to degrade at temperatures close to their softening point. It is only applicable to materials that are polar and capable of forming a dipole moment. F. Hot-wire sealing Hot-wire sealing uses a thin wire or strip of metal with a radiussed edge, heated with a low voltage current. This method has found application for the manufacture of polyethylene bags and pouches in tubular form, with the cutting and sealing operations carried out in the one step. It is only applicable to thermoplastic films that can tolerate high temperatures for a short time and also have a low viscosity in the fused stage. When unsupported films are trim sealed by this method, they tend to form a strong bead in their seal areas due to surface tension and orientation. This method is also used to a limited degree with laminated constructions. G. Testing of Heat seals The strength of heat seals is often determined by measuring the force required to pull apart the pieces of film that have been sealed together, either in a dynamic load test or a static load test. The latter test tends to be qualitative whereas the dynamic test (usually performed on some type of tensile testing equipment) is quantitative. The ASTM F 88-00 standard test method for determination of the seal strength of flexible barrier materials describes the procedures to be followed, along with the equipment to be used and the methods to be employed, thus enabling useful comparisons to be made between the seal strength of different materials or different sealing methods. However, many other less formalized methods of testing heat seals are widely used in the food industry. For example, polarized light can be used with transparent materials, the birefringence patterns providing a visual guide to the consistency of the seal and highlighting any gaps or stresses. Key Points Thermoplastic Polymers in Packaging, Thermoplastic polymers are commonly used in food packaging and are permeable to small molecules such as gases, water vapor, and organic compounds, unlike materials like glass or metal. Mechanisms of Permeability: Pore Effect: Gases and vapors move through tiny cracks or pores in the polymer. Solubility-Diffusion Effect: Gases dissolve in the polymer, diffuse through it, and evaporate on the other side. This is the primary mechanism for true permeability. Permeation of Gases: Gases move through polymers at different rates. For example, CO2 permeates much faster than O2 and N2 due to its higher solubility in the polymer. Permeability varies with temperature and pressure, and interactions between the gas and polymer also play a role. Factors Affecting Permeability: Pressure: Higher pressures generally increase permeability. Temperature: Permeability changes with temperature, often increasing with rising temperatures. Sorption: The process by which permeant molecules enter and disperse through the polymer. Migration: Certain substances in plastics, like additives, can migrate into the packaged food, potentially affecting its quality or making it unsafe. Measurement of Permeability: Various methods, such as the pressure increase, volume increase, and concentration increase methods, are used to measure gas permeability in polymers. Water Vapor Transmission: This is often tested using desiccants and controlled humidity, though results from flat films don't always match real-life packaging performance. Organic Compound Permeability: Organic compounds can interact with and swell polymers, increasing the permeability rate. This is particularly important for packaging volatile food flavors and aromas. Processing and Converting of Thermoplastics This refers to different ways of turning raw thermoplastic materials into useful products. Extrusion is the main method where thermoplastic materials are melted and shaped into sheets or films using a machine with a screw. There are two types: Flat Film (Cast Film): The melted plastic is cooled on rollers to make smooth films. Tubular Film: The plastic is inflated into a bubble to make stronger films. Monolayer vs. Coextrusion Monolayer Extrusion: Uses one type of polymer to make a simple, uniform film. Coextrusion: Combines different polymers to make layered films that are stronger or more protective. The Calendering process presses melted plastic between rollers to make smooth, even sheets. It gives better control over thickness and surface finish. Coating and Laminating, These processes add layers to improve the film's performance. Coating: A thin layer of plastic is applied to a surface, like paper or aluminum. Laminating: Two or more layers are bonded together to create stronger or more protective films. Metallization, A thin layer of metal (usually aluminum) is applied to the plastic film to improve its barrier properties and give it a shiny look. Orientation makes plastic films stronger by stretching them, aligning the molecules. This improves strength, flexibility, and clarity, but can make the film less stretchy. Shrink Films are heated to shrink and wrap tightly around products, providing protection. Common shrink materials are PVC, PE, and PP. Stretch Films are pulled and wrapped around large items like pallets to hold them together. These films stick to themselves and can stretch up to 400%. Cross-Linking makes thermoplastic films stronger by chemically bonding molecules together, improving heat resistance and durability. Microperforation, Tiny holes are made in plastic films to allow gas and moisture to pass through, which is useful for packaging fresh produce. Injection Molding, This process is used to create solid plastic items like containers and caps. It involves melting plastic and injecting it into a mold, where it cools and hardens. Blow molding is a process used to produce hollow objects, primarily bottles and containers, by expanding molten plastic inside a mold using air pressure. The process typically involves three steps: 1. Melting the resin 2. Forming the parison (a molten plastic tube) 3. Blowing the parison to the desired shape. Types of Blow Molding: 1. Extrusion Blow Molding (EBM): ○ A continuous tube of plastic is extruded, and air is blown into it inside a mold to form the final shape. 2. Injection Blow Molding (IBM): ○ The parison is injection-molded in one mold, then transferred to another for blowing. 3. Stretch Blow Molding (SBM): ○ Parison is stretched in both longitudinal and transverse directions under heat to form a biaxially-oriented container. Foamed Cellular Plastics Foamed plastics are lightweight materials with numerous cells dispersed throughout the polymer. These materials include: Polystyrene (PS): Widely used for food packaging like egg cartons and trays. Polyurethane: Common for cushioning applications. Heat Sealing Heat sealing is a critical process in packaging, ensuring the integrity of the package by bonding materials together using heat. Different sealing methods include: 1. Conductance Sealing: Heated metal bars press together two layers of film. 2. Impulse Sealing: A short burst of electrical energy heats metal bars to fuse the films. 3. Induction Sealing: Non-contact sealing using electromagnetic induction to heat and bond materials with aluminum foil. 4. Ultrasonic Sealing: Vibrations generate heat at the sealing interface to bond films quickly without overall heating. 5. Dielectric Sealing: Uses high-frequency currents to heat and seal films, mainly applied to polar materials like PVC. Testing of Heat Seals: Seals are tested for strength through dynamic and static load tests to ensure package integrity. Reference Federation, B. P. (n.d.). Thermoplastics. British Plastics Federation. https://www.bpf.co.uk/plastipedia/polymers/polymer-thermoplastics.aspx#formula ting Siracusa, V. (2012). Food Packaging Permeability Behaviour: a report. International Journal of Polymer Science, 2012, 1–11. https://doi.org/10.1155/2012/302029 Robertson, G. L. (2005). Food Packaging. CRC Press. https://doi.org/10.1201/9781420056150 I. INTRODUCTION The commercial packaging of foods in metal containers began in the early 19th century, following on from the discovery in the 1790s by the French confectioner Nicolas Appert of a method of conserving all kinds of food substances in containers, a method to which the term canning is now applied indiscriminately, whether the container is made from tinplate, aluminum, glass or plastics. The earliest can makers were tin-smiths who turned out tin plate containers with skill and imagination, in various sizes and shapes. Both ends were soldered to the body, with a hole about 25mm in diameter at the top. After the can was filled through this hole a metal disc was soldered into place. Mechanization of the can-making process was made possible by the development of a method called double seeming, to attach ends to the soldered can body. Even though many of the fundamental manufacturing processes, such as double seaming and body forming were developed in the late 19th century, the evolution of can-making continues. Related technologies such as metallurgy and food engineering are also advancing, creating new applications for metal packaging materials. Out of the total estimated world market of metal cans, about 78% is for drink cans and about 18% for processed food cans. The remainder are aerosol and general line cans. Drink cans may be divided into those for non-carbonated drinks (liquid coffee, tea, sports drinks, etc.) and carbonated beverages (soft drinks and beer), many of which pass through a pasteurization process. II. TYPES OF METAL PACKAGING MATERIALS Four metals are commonly used for the packaging of foods: steel, aluminum, tin and chromium. Tin and steel, and chromium and steel, are used as composite materials in the form of tinplate and electrolytically chromium-coated steel (ECCS), the latter being somewhat unhelpfully referred to as tin-free steel (TFS). Aluminum is used in the form of purified alloys containing small and carefully controlled amounts of magnesium and manganese. Copper is used as a wire electrode during the welding of three-piece tinplate containers but does not become part of the finished container. A. STEEL Steel is a popular choice for food packaging due to its excellent combination of strength, durability, and recyclability. Its strength and durability are derived from its composition, which typically includes iron and carbon, often with other alloying elements. These elements contribute to the metal's structural integrity, making it resistant to deformation and breakage. Additionally, steel's recyclability is a result of its magnetic properties, which allow it to be easily separated from other materials in recycling facilities. This makes it a more environmentally friendly option compared to other packaging materials that may be difficult to recycle. Advantages of Steel for Food Packaging: 1. Steel cans are robust and can withstand rough handling during transportation and storage. 2. Steel provides a good barrier against oxygen, light, and moisture, helping to preserve the quality and freshness of food. 3. Steel can be used for a wide range of food products, from canned fruits and vegetables to soups and pet food. 4. Steel is one of the most recycled metals, making it an environmentally friendly option. 5. Steel is often more cost-effective compared to other metal packaging materials like aluminum. B. ALUMINUM Aluminum is important for environmentally friendly packaging since it has many benefits, including being lightweight, durable, barrier-like, and recyclable. These characteristics support resource efficiency, lessen food waste, and lessen the impact on the environment. 1. Durability and Lightweight. The lightweight nature of aluminum reduces the overall weight of packaged goods, leading to lower transportation costs and reduced fuel consumption. It is easier to handle, both for consumers and during the manufacturing process. Despite being lightweight, aluminum is robust and can withstand significant pressure and impact without breaking or deforming. This makes it ideal for packaging items that need to be transported over long distances. 2. Excellent Barrier Properties. It helps to preserve items and prolong their shelf life by offering a strong barrier against light, moisture, and oxygen. 3. Recyclability. Aluminum's quality is unaffected by endless recycling. Because of this, it is a sustainable choice that lessens the impact on the environment and the demand for aluminum. 4. Versatility. Aluminum is appropriate for a broad range of packaging applications, including foil wraps and cans, because it can be shaped and sized to fit different needs. It is easy to shape, stable and resistant to organic acids, and a good conductor of electricity and heat. C. TIN Tin is well-known for its long life and capacity to give retail packaging structure and solidity, and it is frequently used to package a variety of goods, such as food, high-end wine, and cosmetics. Because of its adaptability, which enables it to be tailored to match different box sizes and requirements, it is a popular option for businesses wishing to mark their goods and make them stand out on store shelves. Tin packaging has become very popular because of its various advantages as well as its capacity to present and protect goods in an elegant and appealing manner. 1. Durability. Tin is a strong substance that offers superior protection for the contents, preventing external forces and physical harm. 2. Versatility. It can be molded into a variety of shapes, including boxes, cans, and containers, which makes it appropriate for a broad range of goods. 3. Aesthetic Appeal. Tin packaging is appealing for high-end goods since it has a classic charm and may be enhanced with elaborate features. 4. Barrier Properties. Tin provides effective defense against gasses, light, and moisture, extending the products' shelf life and quality. 5. Recyclability. Tin is more environmentally friendly than certain other materials since it can be recycled. D. CHROMIUM COATED STEEL Packaging materials frequently contain chromium, especially electrolytic chromium coated steel (ECCS), sometimes referred to as tin-free steel. The following are some essential properties of chromium as a material for packaging: 1. Corrosion Resistance: Chromium has a high level of corrosion resistance, which helps shield the package's contents from outside influences. 2. Durability: Because of its hardness and sheen, this metal is resilient to physical strain. 3. Thin Coating Efficiency: ECCS is more affordable while still offering sufficient protection because it needs a far thinner layer of chromium than tin. 4. Adhesion Properties: Chromium coatings increase the lacquers' and other protective layers' adherence, improving the packaging's overall functionality. 5. Thermal Conductivity: Packaging that needs thermal treatments like pasteurization or sterilization benefits from chromium's strong thermal conductivity. III. METAL RECYCLING PROCESS Figure 1. Process of Recycling Metal 1. Collection. It is the first step of the metal recycling process. Metals are gathered and put into designated containers during the collection process. End users, like industrial or construction workers, put scrap metal in bins that are marked for collection by a contracted recycling facility. In order to get the scrap metal ready for recycling, the metal owner may occasionally need to move it to the contractor's yard. 2. Separation. It separated recyclables from non-recyclables in the incoming scrap stream using specialized magnetic equipment. In the recycling process, separating or sorting is essentially the quality-control stage. The quality of the final product is influenced by the type of metal that is recycled, which makes this process especially crucial. 3. Preparation. Metal recyclables are sent to a shredder machine after being identified as recyclable, at which point the recycling process formally starts and the metal is crushed and broken down. By using this method, the amount of scrap metal in the batch is reduced and recycling becomes more effective. 4. Melting. It entails applying extremely high heat to burn the shredded metal. Metal is transformed from solid into liquid through this process. Recycling facilities typically place various metal types in separate furnaces for quality control purposes; the amount of heat in each furnace is determined by the alloying elements found in the scrap metal. 5. Purification. Smelted metal is purified in metal recycling plants using a variety of techniques. Chromatography, zone refining, distillation, and electrolysis are examples of common methods. Every purification method has pros and cons of its own, and different refining techniques yield different levels of quality in the final product of a metal. 6. Cooling and Solidifying. Cooling and solidification are the next steps for the pure melted steel. In order to move hot, molten metal from the heating and purification chambers to the cooling containers, recycling plants employ advanced mechanisms. In order to maintain the desired qualities of the finished product, such as weight, density, heat conductivity, and corrosion resistance, additional chemical compounds are incorporated to help stabilize the molten metal. Additionally, structural metal is formed by recyclers using specialized containers to transform molten metal into a variety of sizes and shapes that are used in a variety of industries. 7. Manufacturing. The solidified metal can be picked up and shipped to a manufacturer to be transformed into new products after it has been made to order. IV. MANUFACTURE OF TINPLATE The term tinplate refers to low-carbon, mild steel sheet varying in thickness from around 0.15-0.5 mm with a coating of tin between 2.8 and 17 gsm (gm-2) (0.4-2.5 µm thick) on each surface of the material. The combination of tin and steel produces a material that has good strength, combined with excellent fabrication qualities such as ductility (the capability to undergo extensive deformation without fracture) and drawability (these attributes arise from the grade of steel selected and the processing conditions employed in its manufacture) as well as good weldability, nontoxicity, lubricity, lacquerability and a corrosion-resistant surface of bright appearance (these latter properties are due to the unique properties of tin). Furthermore, the tin coating adheres sufficiently to the steel base so that it will withstand any degree of deformation that the steel is able to withstand without flaking. Continuous demand for improved quality and more economic production has led to the development of highly sophisticated manufacturing techniques. These are outlined in this section, together with their effects on the metallurgical and mechanical characteristics. The chemical composition of the base steel has a very significant effect on the subsequent corrosion resistance and mechanical properties of the tinplate. Figure 2. Structure of Tinplate Tin Coating The role of tin coating is an essential component of the can construction and plays an active role in determining shelf life. The most significant aspect of the role of the tin coating is that it protects the steel base-plate which is the structural component of the can. Without a coating of tin, the exposed iron would be attacked by the product and this would cause serious discoloration and off-flavors in the product and swelling of the cans; in extreme cases the iron could be perforated and the cans would lose their integrity. The second role of tin is that it provides a chemically reducing environment, any oxygen in the can at the time of sealing being rapidly consumed by the dissolution of tin. This minimizes product oxidation and prevents color loss and flavor loss in certain products. V. MANUFACTURE OF ECCS The production of electrolytic chromium/chromium-oxide-coated low-carbon steel sheet (to give ECCS its full name) is very similar to electro tinning, the only essential differences being that, in the former case, flow melting and chemical passivation are not involved. The initial development work was carried out in Japan in the 1960s when tin was on occasions in short supply, and the price was extremely variable. The process involves cathodic deposition in a dilute chromium plating electrolyte (e.g., 50 g L−1 CrO3 and 0.5 g L−1 H2SO4) at a temperature in the range of 50°C–70°C. As shown in Figure 7.1, ECCS consists of a duplex coating of metallic chromium and chromium sesquioxide. The ideal range of coating weights for ECCS is between 0.07 and 0.15 gsm chromium metal and between 0.03 and 0.06 gsm trivalent chromium present as oxide, giving a total coating weight of approximately 0.15 gsm. This is much thinner than the lowest grade of electrolytic tinplate which has a tin thickness of 5.6 gsm. ECCS is coated equally on both sides of the steel. Unlike tinplate, ECCS is not subject to a passivation treatment. Finally, an oil coating of either DOS or butyl stearate oil (BSO) is applied. Recently Li et al. (2011) showed that the different modes of annealing and rolling have remarkable effects on the surface morphology and grain size of ECCS products. In the compact surface chromium coating of batch-annealed (BA) plate with low porosity, the grains were larger and coarser in comparison with that of continuously annealed (CA) plate. Evidence of microcracks was found on the surface of chromium-coated DR plate. The chemical composition of ECCS oxide film on steel consisted of CrOOH or Cr(OH)3 as the main components, plus Cr2O3 and a small amount of H2O. Cr(OH)3 is mainly formed from cathodic films generated in the electroplating process. CrOOH was an intermediate product formed in process of Cr(OH)3 losing water to generate Cr2O3. The surface of ECCS is more acceptable for protective enamel coatings or printing inks and varnishes than tinplate, and the lack of a low melting point (232°C) tin layer means that higher stoving temperatures and consequently shorter stoving times can be used for the enameling of ECCS. Unlike flow-brightened tinplate, ECCS is a dull bluish color, which necessitates modification of decoration processes to allow for its poor reflection. ECCS is less resistant to corrosion than tinplate as it has no sacrificial tin layer and, therefore, must be coated on both sides with an enamel or polymer coating. Polymer coatings are applied either by laminating film or extruding polymer directly onto the substrate; this is normally done on both sides. ECCS containers cannot be soldered and, therefore, bonding of ECCS components must be by welding or the use of organic adhesives. If welded, then ECCS must be edge-cleaned prior to welding to remove the chromium layer. This is a slow, costly and mechanically inefficient process. Typically, ECCS is used for components that do not have to be welded, such as ends for tinplate bodies, lids, crown corks, twist-off caps and aerosol bottoms and tops. VI. MANUFACTURE OF ALUMINUM Figure 3. Aluminum Foil Aluminum Foil Aluminum foil is produced from aluminum ingots by a series of rolling operations down to a thickness in the range of 0.15-0.008 mm. Foil is a bright, attractive material, tasteless, odorless, and inert with respect to most food materials. For contact with acid or salty products, it is coated with nitrocellulose or some polymer material. It is mechanically weak, easily punctured, torn, or abraded. Examples of foods packaged in this way include dried soups, sauce mixes, salad dressings, and jams. Aluminum Alloy Most commercial uses of aluminum require special properties that the pure metal cannot provide. Therefore, alloying agents are added to impart strength, improve formability characteristics, and influence corrosion characteristics. Hard-temper aluminum alloy, containing 1.5-5.0% magnesium, is used in food can manufacture. It is lighter but mechanically weaker than tinplate. It is manufactured in a similar manner to aluminum foil. It is less resistant to corrosion than tinplate and needs to be lacquered for most applications. A range of lacquers suitable for aluminum alloy is available, but the surface of the metal needs to be treated to improve lacquer adhesion. VII. MANUFACTURE OF CANS Figure 4. Three-Piece Cans Three-Piece Cans Three-piece cans are mainly used for food but may be used for some non-carbonated beverages, particularly fruit juices. They were the original cans, consisting of a bottom (end), walls (body), and lid. The components of the three-piece can are cut from steel after coating (if used). The wall of the can is rolled to form a cylinder and the seam (joint) is welded. A protective lacquer is then applied to this seam. It can be either liquid or powder and is known as a side seam stripe. A bottom (either a classic or easy open end) is then attached. After filling, a lid (classic end) is attached to close the can. Both the bottom end and the lid have a gasket applied to ensure a hermetic seal. Within three-piece category cans, there are further four classifications determined by the method used to join the side seam of the body cylinder. The methods are soldering, welding, cementing and double seaming. Figure 5. Two-Piece Cans Two-Piece Cans The widest use of these is in the beer and soft drink markets. Two-piece cans have technical, economic, and aesthetic advantages in comparison with soldered or welded three-piece cans. There are two types of Two-piece cans: Draw and iron (D&I) and Drawn and re-drawn Cans (DRD). Two-Piece Drawn and Iron Cans The drawn and wall-ironed can (D&I) is made from a disc of metal 0.30-0.42 mm thick. DWI cans are mainly used for packaging carbonated beverages. The internal pressure supports the thin wall. Two-Piece Drawn and Re-drawn (DRD) Cans These cans are used for fruits, vegetables, tuna, and ready meals. Figure 6. Can Seamer Can Seamer A Can seamer is a machine used to seal the lid (End) to the can body such as in paint or food cans. The lid (End) should be of metal while the body can be of metal (beverage, soup, etc.), paper or plastic. Seam formed is generally leak-proof but this depends on the product being canned. The seam is made by mechanically overlapping the two layers to form a hook. Different parameters of the hook are measured and monitored to check the integrity of the seam under different conditions. Figure 7. Can Lacquers Can Lacquers Lacquer is a resin coating of cans that protects the inside of the can from acids in food which would otherwise attack the metal causing it to corrode. If the can is going to be stored in poor conditions the outside of the can, can be lacquered as well, to protect the metal from corrosion. The lacquering seals and protects the tin from chemical reactions without affecting the food flavor, it also prevents the dissolution of tins into the products. Internal Coatings Protection of the contents from the metal - e.g. iron pick-up in beer or discoloration or some dark-colored fruits, such as plums and strawberries, due to metal contact. Protection from the contents of the can - e.g. acidic soft drinks (which may corrode uncoated metal) or some fish, meats, and soups (which may cause sulfur staining). External Coating Protection from the atmospheric corrosion environment. Support decoration, labeling and consumer information. Influence mobility of the article during filling operations-e.g. beverage cans can only be filled with an external decoration, which provides the necessary friction (mobility) to pass through the filling head. Lacquering Method of Application Lacquers can be applied in the following way: ❖ Electrophoretic Lacquers ❖ Dip Applied Lacquers ❖ Brush Lacquers ❖ Spray Lacquers VII. APPLICATIONS OF METAL PACKAGING MATERIALS Beverages Carbonated Beverages typically use two-piece drawn and iron (D&I) cans, which are highly suitable due to their seamless structure. This type of can ensures strength and pressure resistance, essential for holding carbonated drinks like soda or beer. Non-carbonated Beverages usually use three-piece cans. These are often employed for drinks that don't require as much pressure resistance, such as juices or tea. The three-piece design consists of a cylindrical body with two end pieces (top and bottom). Aerosol Cans: These are used to package food items like canned whipping cream. Aerosol cans are either three-piece or two-piece, depending on the manufacturing process and the type of product being packed. These cans need to be sturdy to withstand the pressure of the aerosolized content. Lids: Metal lids are typically used on jars and bottles, often for products like jam jars and ketchup bottles. These lids provide a strong seal that helps preserve the product's freshness while ensuring safety and ease of use. Drums and Pails: Three-piece cans are also used to manufacture drums and pails. These are larger containers used for transporting bulk food products like oils and other edible fats. The design and material ensure durability and protection of the contents during transit. Food Cans Variety: Food cans come in a broader range of sizes and shapes compared to beverage cans due to the diverse nature of food products. This includes both three-piece welded cans and two-piece steel cans. ○ Three-piece welded cans feature high-integrity welding, which creates a robust, lead-free seal, making them safe for food storage. Shallow Drawn Aluminum Cans: These are commonly used for packaging processed foods lik