NMD1000 Exam 2 Lecture Notes PDF
Document Details
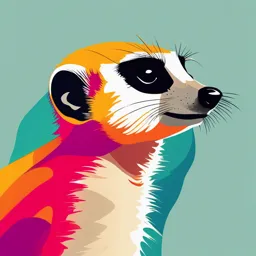
Uploaded by SpiritualHonor
College of Medicine
Tags
Summary
These lecture notes cover the basics of carbohydrates, including classification, monosaccharides, oligosaccharides, and polysaccharides. They detail the chemical structure and physical properties of these molecules. The notes also briefly discuss carbohydrate derivatives.
Full Transcript
17 Chemical Blocks of Life: CHO: ILOs By the end of this lecture, students will be able to 1. Describe how carbohydrates help us function and survive 2. Describe the building blocks of carbohydrates 3. Discuss carbohydrates’ role in health and disease Carbohydrates are generall...
17 Chemical Blocks of Life: CHO: ILOs By the end of this lecture, students will be able to 1. Describe how carbohydrates help us function and survive 2. Describe the building blocks of carbohydrates 3. Discuss carbohydrates’ role in health and disease Carbohydrates are generally hydrates of carbon containing hydrogen and oxygen in a ratio of 2:1, with general molecular formula (CH2O)n, where n > 3. ❖ Classification There are three major classes of carbohydrates - Monosaccharides (mono = one) - Oligosaccharides (oligo= few) 2-10 monosaccharide units. - Polysaccharides (Poly = many) >10 monosaccharide units. Monosaccharides: (Simple sugars) - Depending on the number of carbon atoms, the monosaccharides are named trioses (C3),tetroses (C4), pentoses (C5), hexoses (C6), heptoses (C7).The most abundant monosaccharides in nature are the 6- carbon sugars like glucose and fructose. - They either contain an aldehyde group and are called aldoses, or a keto group and are called ketoses. - The carbon atom that is double bonded to an oxygen atom forms a carbonyl group. Physical properties of Monosaccharides: - Asymmetric carbon atom is a carbon atom attached to 4 different groups or atoms. It gives the ability to show isomerism - Isomers are compounds that have the same chemical formula but have different structures as fructose, glucose and galactose that have the same chemical formula, C6H12O6. Oligosaccharides: The most abundant are disaccharides as: - Maltose: o It is the Malt sugar. Maltose is the major degradative product of Starch. It is hydrolyzed to two molecules of glucose - Lactose: o Lactose is composed of glucose and galactose subunits. It is found exclusively in milk of mammals (Milk sugar). Page 1 of 3 o It is the least sweet sugar. Although glucose can be obtained from several food types, Lactose is the only source of galactose which plays an integral role in various neural and immunological processes including the ABO blood types. o Moreover it was found that Lactose plays a role in absorption of calcium, copper and zinc especially in infancy. o Some patients suffer from “Lactose Intolerance”, they are unable to fully digest the lactose in milk. As a result, they have diarrhea, gas and bloating after eating or drinking dairy products. There are many Lactose free dairy products available now in the market for those patients. - Sucrose (Cane sugar): o Sucrose is composed of glucose and fructose subunits. It is obtained from cane sugar. It is also present in various fruits. o The fructose component gives it a very sweet taste. Therefore, sucrose derivatives are sometimes used by diabetics and obese patients as an alternative to glucose giving them sweet taste with less concentration aiming to lower their insulin and blood glucose levels. However, it was found that high levels of fructose increase hunger and sugar craves. Polysaccharides: Most of the carbohydrates found in nature occur in the form of high molecular polymers called polysaccharides. Important polysaccharides include: - Glycogen: Are the branched form of polysaccharides from animal sources and the main storage form in humans. - Starch: Are the plant storage form of CHO and the most abundant CHO in diet. Found in potatoes, rice and pasta. It consists of 2 main molecules Amylose and Amylopectin. Both consist of glucose molecules linked together while Amylopectin is the branched component in starch. - Dextrins: These are highly branched. Since they do not easily go out of vascular compartment they are used for intravenous infusion as plasma volume expander in the treatment of hypovolumic shock. - Unbranched cellulose: (Structural form of CHO in plants). Humans cannot digest cellulose. However, it is consumed in the diet as fiber. - Fiber helps the digestive system to keep the food moving through the gut and to avoid constipation. Examples of food containing cellulose include green leafy vegetables and some fruits as unpeeled apples. - Each of these polysaccharides is a polymer of glucose. ❖ Carbohydrate Derivatives: These are sugar molecules that have been modified with substituents other than hydroxyl groups. Most sugar derivatives occur naturally and have important biological functions. Examples include: - Deoxy-monsaccharides: In which The –OH is replaced –H as Deoxyribose sugar the DNA sugar - Amino Sugar: -OH is replaced by –NH2, the sugar is then designated as –osamaine as glucosamine in proteoglycans (see in Structure & Chemistry of C.T. Ground Substance) - Sugar Acids: In which the –CH2OH is oxidized to –COOH as Glucuronic acid which is synthesized in the liver and plays a major role in detoxification and elimination of toxic compounds. Page 2 of 3 - Immature babies have low levels of glucuronic acid this makes them more prone to jaundice due to accumulation of bilirubin without being detoxified by glucuronic acid in severe cases “kernicterus” this may lead to affection of the brain leading to mental retardation and vision problems. - Patients with liver failure are very prone to drug toxicity due to decrease in glucuronic acid synthesis and inability to detoxify the drug. - Sugar Alcohols: In which COOH is reduced to CH2OH. Examples include mannitol used as diuretic to help the body produce more urine in case of edema And sorbitol used to relieve constipation and as an artificial sweetner for patients following low caloric diets as it contains nearly 50% less calories than normal sugars and have the ability to highly stimulate the taste buds giving the same taste as sugar. Sorbitol is normally present in some fruits as Cherries giving them a sweet taste with low calories. However excess use of sorbitol leads to bloating and digestion problems. ❖ What is the importance of Carbohydrates? Carbohydrates have a wide range of functions, including providing a significant fraction of the dietary calories for most organisms, supplying and acting as a storage form of energy in the body, CHO also serve as cell membrane components that mediate some forms of intercellular communication. Although a common source of energy, where 1 gram of dietary CHO provides 4 Cal. However, carbohydrates are not essential nutrients, because humans are able to obtain 100% of their daily energy requirement from proteins and fats. However, they are more readily converted than proteins or fats into the key energy monosaccharide: glucose. When digested, all carbohydrates are broken down to glucose, which is then transported in the blood to cells for energy production. This helps CHO to induce early but short term satiety when compared to proteins and fats. ❖ Do Carbohydrates play a role in disease? Carbohydrates may directly influence human diseases by affecting physiological and metabolic processes, acting as risk factors for many diseases. For Example: Abnormal metabolism (usage by the body) of glucose is related to many serious diseases with Diabetes Mellitus coming on top also certain types of cancers might be related. Inborn errors in CHO metabolism can cause wide range of abnormalities including mental retardation and hepatomegaly (Increase in the liver size). Some GIT (digestive system) diseases and disturbances are related to abnormal digestion or breakdown of CHO as vomiting, bloating and diarrhea. Ingestion of too much CHO (a very common dietary habit nowadays) is directly related to obesity and its dangerous health consequences as heart diseases. Page 3 of 3 18 Chemical Blocks of Life: Lipids: ILOs By the end of this lecture, students will be able to 1. Describe how lipids help us function and survive 2. Describe the building blocks of lipids 3. Discuss lipids’ role in health and disease Lipids are heterogeneous group of organic compounds related to fatty acids. They contain C, H, and O. They may also contain P, N, and S. Lipids are relatively insoluble in water, and soluble in in nonpolar solvents (fat solvents) as ether, chloroform, benzene and acetone. Classification of lipids: I. Simple lipids:- esters of fatty acids with different alcohols. True Fats:- These are esters of fatty acids with glycerol. If glycerol is attached to 1 fatty acid it is called Monoacylglycerol. If glycerol attached to 2 fatty acids it is diacyglycerol, If all the three hydroxyl groups of glycerol are esterified, fats are known as triacylglycerol, (Triglycerides). The 3 fatty acids linked to the glycerol are usually different. Waxes:- Esters of fatty acids with high molecular weight alcohols Figure(2-1): Structure of Triacylglycerol II. Complex lipids:- Esters of fatty acids and alcohols together with some other head groups. A. Phospholipids:- (see Lipid Structure & functions in bio-membranes) B. Glycolipids:- Lipids containing fatty acid, sphingosine and carbohydrate residues. C. Others:- Include sulfolipids, amino lipids and lipoproteins, which are modified forms of lipids. III. Derived lipids: include the hydrolytic products of the simple and complex lipids. Eg. Fatty acids, glycerol, cholesterol, Fat soluble vitamins: Vitamins A, D, E and K. ❖ Properties of Fatty Acids: Fatty acids are molecules consisting of carbon, hydrogen and oxygen, principally linked by non- polar carbon–hydrogen (C–H) bonds forming chain with a carboxyl group at one end. The terminal carboxyl group (–COOH) ionizes, becoming –COO−. This anionic group has an affinity for water, giving the fatty acid its amphipathic nature (having both a hydrophilic and a hydrophobic region). However, for long chain length fatty acids (LCFA), the hydrophobic Page 1 of 3 portion is predominant. These molecules are highly water insoluble and must be transported in the circulation in association with protein. Fatty acid saturation - Some Fatty acid are “Saturated” containing no double bonds others are “ Unsaturated” containing one or more double bonds ( mono- or polyunsaturated). - Double bonds in natural fatty acids are in the cis (functional group on the same side) configuration. The introduction of a cis double bond causes the fatty acid to bend or kink at that position. If the fatty acid has two or more double bonds, they are always spaced at three- carbon intervals. Cis double bonds are present in natural foods as salmon, nuts and olive oil. - Trans (functional group on opposite sides) double bonds predominate in artificially produced foods and, along with saturated fatty acids, they are associated with an increased risk of atherosclerosis. Figure (2-1): Saturated (top) and Unsaturated (bottom) Fatty Acids (Left) Cis bonds (top) and Trans bonds (bottom) (Right) - In humans, the majority are saturated or monounsaturated. In general, addition of double bonds decreases the melting temperature (Tm) of a fatty acid, whereas increasing the chain length increases the Tm. Because membrane lipids typically contain LCFA, the presence of double bonds in some fatty acids helps maintain the fluid nature of those lipids. Fatty acid chain length - According to chain length of fatty acids they can be: 1. Short chain fatty acids: Contain from 2 to 6 carbon atoms as: Acetic acid (2 C): CH3-COOH. High-fiber foods, such as fruits, veggies, legumes and whole grains, encourage the production of short-chain fatty acids by human body. 2. Medium chain fatty acids (MCFA): Contain 7-12 carbon atoms as capric acid (10C). MCFA are present mainly in coconut oil and dairy products. They are now gaining popularity as a healthy diet component and as a dietary supplement where it contains fewer calories compared to long chain fatty acids but they give higher satiety and reduce appetite. Moreover, they improve gut health by killing harmful bacteria without impacting good bacteria in the gut therefore preventing diarrhea and bloating. 3. Long chain fatty acids: Contain more than 12 carbon atoms as Palmitic (16 C). They are the greatest bulk of fatty acids in animal tissues and most animal diets. - In the human body, fatty acids with an even number of carbon atoms (16, 18, or 20) predominate, with longer fatty acids (>22 carbons) being found in the brain. Page 2 of 3 Rancidity: - Lipids exposed to light, heat, moisture and bacterial action change into rancid fat. Rancid fat has bad odour, taste and acidic in reaction. - Rancidity results from slight hydrolysis of fat leading to liberation of short chain FA volatile having bad odour (hydrolytic rancidity); at the same time oxidation of the unsaturated fatty acids occurs with the formation of peroxides, aldehydes and ketones (oxidative rancidity). - Rancidity can be inhibited by addition of antioxidant like vitamins A, E; and by keeping fat in cold clean place away from light and moisture. Fatty acids Numbering: - There are two systems of numbering the carbon atoms in a fatty acid Figure (2-2): Fatty acids numbering Essential fatty acids (EFA): - Lipids can be either derived from the diet or synthesized de novo. Exact concentrations vary considerably from person to person. Essential FA that must be provided in diet include: - Linoleic acid, the precursor of ω-6 fatty acids (ie: Fatty acids with the terminal double bond being six bonds from the ω end.) - α-linolenic acid, the precursor of ω-3 fatty acids. (ie: Fatty acids with the terminal double bond being three bonds from the ω end.) - Both ω-6 fatty acids and ω-3 fatty acids are important for growth and development. ω-3 fatty acids are present in salmon, sardines and flaxseeds, while ω-6 fatty acids are found in a wider variety of food as walnuts, peanuts, corn and sunflower oils. - Essential fatty acid deficiency occurs most often in infants fed diets deficient in EFAs. Signs include hair loss, low platelets and, intellectual disability. Dietary replenishment of EFAs reverses the deficiency. Lipids in health and disease: Lipids carry out a multitude of crucial roles where they act as: - Structural elements in biological membranes - Store and supply energy with 1 gram of dietary lipids providing about 9 Cal which is more than double the amount of energy provided by carbohydrates. - Lipids also function as signalling molecules in cellular response pathways and aid to solubilise and transport of fat-soluble vitamins. - Disruption of their levels whether by increased dietary intake or abnormal metabolism by the body is related to many diseases including obesity, diabetes, atherosclerosis, hypertension also abnormalities in fat-soluble vitamins and transport of lipids in blood. Page 3 of 3 19 Chemical Blocks of Life: Proteins ILOs By the end of this lecture, students will be able to 1. Describe how proteins help us function and survive 2. Describe the building blocks of proteins 3. Discuss proteins’ role in health and disease What are proteins? Proteins are the most abundant and functionally diverse molecules in living systems. They serve plenty of functions in the body such as: Enzymes and polypeptide hormones direct and regulate metabolism in the body, Contractile proteins in muscle permit movement. In bone, the protein collagen forms a framework for the deposition of calcium phosphate crystals. Collagen, elastin and keratin proteins are also very important in the structure of skin, hair and nails. In the bloodstream, proteins, such as hemoglobin and albumin, transport molecules essential to life, whereas immunoglobulins fight infectious bacteria and viruses. Essential for forming coagulation factors, which control bleeding Essential for growth and development Proteins form molecules (secondary messengers), that help transmit signals between and inside cells. What are the building blocks of proteins?(Figure 1) These are called amino acids and are characterized by the following: An amino acid (a.a) is an organic acid (contains the carboxylic group COOH), in which one hydrogen atom is replaced by an amino group (NH2). The amino group is usually attached to α- carbon atom next to the -COOH. In order to form proteins, amino acids are attached to each other through their carboxylic and amino groups, by a very strong bond called peptide bond There are 20 naturally occurring amino acids that cells use to make proteins These naturally occurring amino acids are called primary or common amino acids Figure 1. General structure of (They are the only a.a with codes on DNA) amino acids 1 Most amino acids have a central carbon atom (α-carbon) to which is attached a hydrogen atom, an α-amino group,( NH3 +), α-carboxyl group,( COO–.), and R group (side chain) (N.B. an R group is composed mainly of carbon and hydrogen atom +/- other chemical groups) The side chain or R group distinguishes each amino acid chemically. Some amino acids have unique groups in their side chains, for example: Sulfur group: in methionine and cysteine Hydroxyl group: in serine, threonine and tyrosine Imino group (NH instead of NH2): in proline Classification of aminoacids According to structure: amino acids can be aliphatic (open chain of carbon and hydrogen) or aromatic (contain benzene ring) According to charge: amino acids can be neutral (carry no charges), acidic (can donate hydrogen or proton and carry a negative charge in physiologic pH), or basic (can accept protons or hydrogen, and carry a positive charge in physiologic pH) According to biological importance: amino acids can be essential (have to be taken in diet), non-essential (can be synthesized in our bodies), or semi-essential (histidine and arginine ) which are synthesized in sufficient amounts in our body, but if their requirements increase such as in periods of growth, pregnancy, after surgeries and in chronic diseases such as liver and kidney diseases, they have to be taken in diet. According to polarity: Which is the ability of the amino acid side chain to gain or lose protons or participate in hydrogen or ionic bonds, amino acids can be non-polar (hydrophobic and always neutral) and polar (hydrophilic, can be neutral, acidic and basic) (Figure 3) Significance of nonpolar aminoacids (Figure 2) a) In proteins found in aqueous solutions (a polar environment), the side chains of the nonpolar amino acids (acting as hydrophobic) tend to cluster together in the interior of the protein where they are away from water. This phenomenon, known as the hydrophobic effect, help give the protein its three-dimensional shape. b) In proteins that are located in a hydrophobic environment, such as a membrane, the nonpolar R groups are found on the outside surface of the protein, interacting with the lipid environment Figure 2: Distribution of polar and nonpolar a.a 2 Significance of polar aminoacids (Figure 2) a) The polar side groups participate in hydrogen bond formation.(which helps stabilize the protein structure) b) The side chain of cysteine contains (−SH), which is an important component of the active site of many enzymes. It also participates in formation of the disulfide bond that stabilizes the protein structure. Clinical implication Sickle cell anemia, a disease of red blood cells that causes them to become sickle shaped rather than disc shaped, results from the replacement of polar glutamate with nonpolar valine at the sixth position in the β subunit of hemoglobin A. This change causes the RBCs to become sticky when there is insufficient oxygen and can block the blood vessel causing severe episodes of pain.(Refer to heart and circulation module) Charged polar side chains Figure 3: Classification of a.a according to polarity 3 Amphoteric property of amino acids: (Figure 4) Amphoteric property means that in solution, amino acids can behave as acids and alkalis due to the presence of both an acidic group (-COOH) and a basic group (-NH2). On complete ionization of neutral amino acids as dipolar ions (Zwitterions), they carry both negative and positive charges, which are equal. The pH at which an amino acid carries equal positive and negative charge (i.e. electrically neutral) is called its isoelectric point (IEP) or pI. When an electric current is passed in a medium where an amino acid occurs at its IEP, its ions do not migrate towards any of the electrodes., and it precipitates in its place Through their amphoteric property, plasma proteins can form an efficient buffer system and play an important role in maintaining the pH of the blood N.B. Zwitterion: It is the dipolar ion of an amino acid at its IEP in an aqueous medium Figure 4 Clinical implications 1-Some aminoacids are precursors to some important compounds ex: tyrosine is a precursor of thyroid hormone, adrenaline, nor-adrenaline and melanin (skin and hair pigment). Thus in tyrosine deficiency, patients may notice loss of their hair and skin color. 2-Glutamine is a precursor of GABA (neurotransmitter).Severe deficiency of glutamine can cause convulsions. 3-Hydroxy-conatining aminoacids play a role in signal transduction. (refer to signaling across biomembranes lecture) 3-Some aminoacids have been linked to obesity such as sulfer containing aminoacids 4- Intake of branched chain aminoacids (Valine, leucine, isoleucine) is useful for patients suffering from liver diseases and in bodybuilders exercises. 5-Intake of excessive over-the-counter aminoacids (As in athletes training in the gym), is very dangerous as this represents an overload on kidney and can lead to kidney failure. 4 Proteins in disease 1-Inborn errors of aminoacids metabolism can lead to several manifestations such as mental retardation and blindness. 2-Defects in the globin protein of hemoglobin can lead to serious conditions ranging from breaking of red blood cells (hemolysis) to inability of oxygen delivery to tissues. 3-Deficiency of immunoglobulins increases the susceptibility of infections and can lead to death from minor infections. 4-Defects in enzymes can lead to serious diseases (for example glycogen storage diseases that lead to liver enlargement and death). 5- Defects in structural proteins such as collagen can lead to skeletal deformities. 6-Defects in production of hormones can lead to several diseases such as diabetes mellitus (defects in insulin). 7-Deposition of abnormal proteins can lead to diseases such as Alzheimer’s disease. 8-Deficiency of coagulation factors can lead to uncontrolled wound bleeding. 5 20 Chemical Bonds ILOs By the end of this lecture, students will be able to 1. Differentiate between the different types of chemical bonds 2. Deduce how bonds can alter structure of biological molecules 3. Interpret the importance of bonds in health and disease What is a bond? A link or force between neighboring atoms in a molecule or a compound. Atoms might be connected by strong bonds and organized into molecules or crystals. Or they might form temporary, weak bonds with other atoms that they bump into or brush up against. Both the strong bonds that hold molecules together and the weaker bonds that create temporary connections are essential to the chemistry of our bodies, and to the existence of life itself. Why form chemical bonds? The basic answer is that atoms are trying to reach the most stable (lowest-energy) state that they can. Many atoms become stable when their valence shell is filled with electrons or when they satisfy the octet rule (by having eight valence electrons). If atoms don’t have this arrangement, they’ll try to reach it by gaining, losing, or sharing electrons via bonds Classification of bonds Bonds are classified to covalent and non-covalent bonds. They are of equal importance, however covalent bonds are stronger than non-covalent bonds. I) Covalent bonds (Figure 1) Covalent bonds are the strongest type of bonds. They form by the sharing of electrons between adjacent atoms; an example is the carbon–carbon (C–C) bond. More than one pair of electrons are shared between two atoms to form a double or triple covalent bond, for example C=C. Figure 1.Covalent bond formation 1 Covalent bonds can be either polar or non polar: Polar covalent bonds A polar covalent bond is much like a covalent bond, except that it occurs between atoms that have differing electronegativity. When this happens, the electrons are still shared, but they tend to spend more time around the more electronegative atom versus the other. Such an example is with water. Oxygen is very electronegative, while hydrogen is not. The electrons tend to favor oxygen and spend more time Figure 2.polar vs nonpolar covalent around it. bond Nonpolar covalent bonds Nonpolar covalent bonds form between two atoms of the same element, or between atoms of different elements that share electrons more or less equally. For example, molecular oxygen (O2) has a nonpolar covalent bond because the electrons are equally shared between the two oxygen atoms. Covalent bonds of biomedical importance These are usually the bonds binding the building blocks of macromolecules 1) Glycosidic bonds (Figure 3) These bonds join a carbohydrate molecule to another one (Whether the other molecule is a CHO or not). For building carbohydrates, monosaccharides are held together by glycosidic bonds in order to form oligo and polysaccharides. The bond is named after the number of the 2 carbons participating in it (for example, if C1 in one monosaccharide is joined to C4 in the other monosaccharide, the bond will be termed 1-4). The bond is also given a symbol α or β according to whether the hydroxyl group participating in its formation is looking upwards or downwards The position of glycosidic bond can determine how the body metabolizes it. For example, although maltose and cellulose have the same building units (2 glucose units joined by 1-4 glycosidic bond), the bond in maltose is of the α type while that of cellulose is of the β type. The effect of this is that maltose can be digested in the body due to presence of the enzyme α 1-4 glucosidase that digests the bond in maltose, while in Figure 3.Glycosidic bond cellulose ,the β 1-4 glycosidic link can’t be digested 2 2) Peptide bonds (Figure 4) These are one of the strongest covalent bond. They bind amino group in one aminoacid with the carboxylic group of another aminoacid. Formation of peptide bonds require a large amount of energy and so does its breakage. Denaturation of protein doesn’t involve breaking Figure 4.peptide bond formation down peptide bonds. Peptide bonds give the protein its primary structure. 3) Ester bonds (Figure 5) These form between OH of an alcohol and OH of a carboxylic groupwith removal of H2O. Esterification can be seen in the process of condensation between fatty acids and glycerol to form triacylglycerols, in the formation of some neurotransmitters such as acetylcholine, and in the structure of several drugs. These bonds are broken down by esterase enzymes. Acetylcholinesterase is responsible for breaking down acetyl choline and hence ending of nerve impulse. Figure 4.Ester bond formation Phosphodiester bonds result from interaction between sugar carbons of nucleotides in order to form DNA and RNA (refer to nucleotides and nucleic acids lecture) 4) Disulfide bond (Figure 6) Disulfide bonds are sulfur-sulfur bonds formed within a protein when the thiol (-SH) groups of two cysteine residues are each oxidized, resulting in the net loss of the hydrogen atoms and binding of sulfer atoms together. Cysteine moieties can be either adjacent or far from each other. Disulfide-bond formation is a reversible process with numerous biological functions, including stabilization of protein fold, enzyme catalysis, and protection against oxidative damage. Figure 4.Disulfide bond formation 3 5) Double bonds Covalent bond resulting from the sharing of two pairs of electrons; e.g., H2C==CH2 (ethylene). An example of double bond in our body is the unsaturated fatty acids which can have one or more double bonds. Unsaturated fatty acids (Ex. Linoleic and linolenic) that are present in olive oil, are an important part of a healthy diet. These fats help reduce the risk of heart disease and lower cholesterol levels (among other health benefits) when they replace saturated fats in the diet. Hydrogenation of oil involves changing the double bonds in unsaturated fatty acids in vegetable oil, to single bonds, hence saturated fatty acids are formed. This causes hardening of oil to form the artificial margarine, which has many risks on cardiovascular health. 6) High energy bonds (Figure 7) A chemical bond whose hydrolysis results in the generation of more than 7kcal of energy or, if coupled to an energetically unfavourable reaction, can drive that reaction forward. Energy released during metabolism of carbohydrates, oxidation of fatty acids, or aminoacids might not be used immediately. Hence, they have to be stored in the form of high energy compounds that can release the energy when needed. The main purpose of these molecules is to transfer either inorganic phosphate groups (Pi) or hydride (H-) ions. The inorganic phosphate groups are used to make high energy bonds with many of the intermediates of metabolism. These bonds can then be broken to yield energy, thus driving the metabolic processes of life. High energy bonds are represented by the symbol “~ “ Figure 7.High energy bond in ATP Examples of high energy compounds: 1. Phosphate compounds (ATP, ADP,GTP, GDP, UTP, UDP,UDP- glucose, Creatine phosphate, arginine phosphate, 1,3 bisphosphoglycerate, phosphoenol pyruvate, carbamoyl phosphate) ATP (Adenosine Triphosphate) contains high energy bonds located between each phosphate group. These bonds are known as phosphoric anhydride bonds. ATP is the main energy currency in the body. 2. Sulfer compounds (Acetyl CoA, Succinyl CoA, acyl CoA, HMG CoA) 4 II) Non-covalent bonds Non-covalent bonds are reversible and vital molecular interactions. These include: Ionic bonds Electrostatic interactions Hydrogen bonding 1) Ionic bond (Figure 8) Ionic bonding involves a transfer of an electron, so one atom gains an electron while one atom loses an electron. One of the resulting ions carries a negative charge (anion), and the other ion carries a positive charge (cation). Because opposite charges attract, the atoms bond together to form a molecule. Ionic bonds are usually found in dry forms such as salts and are found in compounds throughout the Figure 8.Ionic bond human body. Ionic compounds are generally water soluble. Biomedical importance of ionic bonds: Protein folding - Ionic bonds play an important role in shaping tertiary and quaternary proteins. As a protein undergoes its folding process, certain atoms on the protein will be attracted to one another and will from ionic bonds that hold the general shape of a protein together.(Refer to protein structure and modification lecture) Ionic bonds are also involved in catalytic reactions of enzymes. 2) Hydrogen bonds (Figure 9) Hydrogen bonds involves the electrostatic attraction between a hydrogen atom and an atom containing a lone pair of electrons in a substance. In order for a hydrogen bond to occur, the hydrogen must be bonded to an electronegative atom. Hydrogen bonds are considerably weaker than covalent bonds. Biomedical importance of hydrogen bonds: They have a pivotal role in the structure and properties of many molecules For example, in DNA, between nucleotide bases adenine–thymine and guanine–cytosine, there are two and three hydrogen bonds, respectively.(Refer to nucleotides and nucleic acids lecture) Breaking of hydrogen bonds is a must before DNA replication. 5 Hydrogen bonding also plays a role in protein folding.( Refer to protein structure and modification lecture) 3) Electrostatic interactions (Figure 10) Electrostatic interactions occur between positively and negatively charged atoms. They can be repulsive or attractive. The electric charge of an atom determines the extent of the electronic interaction between it and other atoms. Electrostatic interactions play a role in formation of the 3D structure of protein. Figure 9.Hydrogen bond Figure 10.Electrostatic interaction 6 21 Enzyme properties & their importance: ILOs By the end of this lecture, students will be able to 1. Describe the nature and properties of enzymes 2. Explain the importance of enzymes in metabolic reactions ❖ What are “Enzymes”? Enzymes are protein catalysts for chemical reaction in biological systems. They increase the rate of chemical reactions taking place within living cells without being changed or consumed. ❖ Why are Enzymes important in the human body? Many reactions required for the living cell would not proceed fast enough at the pH and temperature of the body without enzymes. All the cellular reactions have high energy barrier (high energy needed by the reaction to take place) and proceed too slowly. Enzymes lower the energy barrier of these reactions required for cell survival therefore it increases the rate of the reaction without changing the reaction itself. Unwanted reactions would never occur spontaneously because of high energy barrier. ❖ What is the Nature of these “Enzymes”? 1. Simple enzyme: It is made up of only protein molecules not bound to any non-proteins. As Pancreatic Ribonuclease enzyme. 2. Holo enzyme is made up of protein groups and non-protein component. - The protein component of this holo enzymes is called apoenzyme - If the non-protein component of the holo enzyme is an organic compound it is called a coenzyme. - If the non-protein component of the holo enzyme is an inorganic group it is called cofactor. (Fe 2+, Mn 2+, or Zn 2+ ions). If the non-protein part is bound so tightly to the apoenzyme and is difficult to remove without damaging the enzyme it is sometimes called a prosthetic group ❖ Important Definitions: Isoenzymes (Isozymes) These are enzymes having similar catalytic activity, act on the same substrate and produce the same product but originated at different site and exhibiting different physical and chemical characteristics such as amino acid composition and immunological behavior. Example: LDH (Lactate dehydrogenase) exists in five different forms each having four polypeptide chains. H= Heart and M=Muscle. LDH-1 H H H H LDH-2 H H H M LDH-3 H H M M LDH-4 H M M M LDH-5 M M M M Page 1 of 3 ____________ Rate Limiting Steps The rate limiting step in any reaction is its slowest step. It sets the pace for the entire reaction. It usually occurs early in the pathway. After all, a production line can only be as productive as its slowest worker. Therefore the rate of the entire enzymatic reaction depends on the rate of this reaction. ❖ What are the main properties of Enzymes? 1- Active site Enzyme molecules contain a special pocket or cleft called the active site. The active site, formed by folding of the protein, contains amino acid side chains that participate in substrate binding and catalysis. The substrate (The molecule or reactant that the enzyme acts upon to form products) binds the enzyme non-covalently, forming an enzyme–substrate (ES) complex, where the substrate attaches itself to the specific active sites on the enzyme molecule by reversible interactions formed by Hydrogen bonds and Hydrophobic interactions. ES is then converted to an enzyme–product (EP) complex that subsequently dissociates to enzyme and product. How does the substrate bind to the enzyme? The enzyme substrate binding is no longer viewed as the rigid picture of a key lock binding, a more flexible model of the binding is provided by the “induced-fit model” in which the binding and active sites are not fully pre-shaped. The essential elements of the binding site are present to the extent that the correct substrate can position itself properly. Interaction of substrate with enzyme induces a conformational change in the enzyme, resulting in the formation of a stronger binding site and the repositioning of the appropriate amino acids to form the active site. Figure (5-1): Induced fit Model Page 2 of 3 2- Efficiency Most enzyme- catalyzed reactions are highly efficient proceeding from 10 3 to 108 times faster than uncatalyzed reactions. Typically each enzyme molecule is capable of transforming 100 to 1000 substrate molecule into product each second. 3- Specificity Enzymes are highly specific, interacting with one or a few substrates and catalyzing only one type of chemical reaction. Specificity of enzymes include: - Absolute specificity:- this means one enzyme catalyzes or acts on only one substrate. For example: Glutamate Dehydrogenase will catalyze only the removal of the nitrogen group from glutamate- NOT any other amino acid. - Bond Specificity: Enzymes that are specific for a bond or linkage such as Esterases- acts on ester bonds, Peptidases-acts on peptide bonds, Glycosidases- acts on glycosidic bonds. 4- Regulation Enzyme activity can be regulated, that is, increased or decreased, so that the rate of product formation responds to cellular need. This tight regulation helps in controlling several metabolic functions as the storage and release of energy and maintaining blood glucose levels in fed and fasting states, Digestion and absorption of nutrients, also the formation of complex molecules from the small, simple ones to produce cellular constituents 5- Location within the cell Many enzymes are localized in specific organelles within the cell. Such compartmentalization serves to isolate the reaction substrate or product from other competing reactions. This provides a favorable environment for the reaction and organizes the thousands of enzymes present in the cell into purposeful pathways. Page 3 of 3 22 Mechanism of Enzyme Action: ILOs By the end of this lecture, students will be able to 1. Describe how enzymes work as catalysts 2. Apply the action of enzymes on varied biological processes 3. Classify enzyme families according to their action on different bonds. 4. Predict and appraise the clinical impact of pharmacological activation or inhibition of enzyme activities. ❖ What is the mechanism of action of Enzymes? Enzymes enhance the rate of reaction by lowering free energy of activation A chemical reaction will take place when a certain number of Substrate (S) molecules posses enough energy to attain an activated condition called the “Transition state” Transition State (T*) is an unstable state in which the probability of making or breaking a chemical bond to form the product is very high. The transition state is the top of the energy barrier separating the reactants and products. The rate of a given reaction varies directly with the number of substrate molecules in the transition state. The “energy of activation” (Ea), is the amount of energy required to bring all the molecules in 1 gram-mole of a substrate to the transition state. In the absence of an enzyme, only a small proportion of a population of molecules may possess enough energy to achieve the transition state between reactant and product. Thus the reaction would be extremely slow. Reactions required to digest food, send nerve signals, or contract a muscle simply do not occur at a useful rate without catalysis. An enzyme circumvents these problems by providing an alternative pathway for the reaction with a lower Ea. In general, the lower the Ea, the more molecules have sufficient energy to pass through the transition state and, therefore, the faster the rate of the reaction. Page 1 of 4 Figure (6.1) Changes in energy during the conversion of a molecule of reactant A to product B as it proceeds through the transition state. The enzyme combines transiently with the substrate to produce a transient state having a lower energy of activation than that of substrate alone. This results in acceleration of the reaction. Once the products are formed, the enzyme is free to combine with another molecule of the substrate and repeat the process. ❖ Classification of Enzymes: Enzymes are classified to 6 main classes as follows: Page 2 of 4 Page 3 of 4 Clinical implications: Enzymes are important targets for drug action by inducing either 1. Enzyme activation, e.g., ▪ Tissue plasminogen activators, as alteplase, are drugs that activate plasminogen to its active form, plasmin (that degrades fibrin in the formed blood clot). This class is used for emergency lysis of a blood clot that blocks the blood flow in case of acute myocardial infarction or ischemic stroke. 2. Enzyme inhibition (more common in drug therapy), e.g., ▪ Non-steroidal anti-inflammatory drugs, as ibuprofen, are a class that inhibit the cyclooxygenase enzyme (COX), responsible for the formation of mediators of pain, fever and inflammation. Therefore, they are used as analgesic, antipyretic and anti- inflammatory. (Refer to drugs combating acute inflammation). Page 4 of 4 23 Factors affecting rate of Reactions: ILOs By the end of this lecture, students will be able to 1. Interpret how the rate of reaction can be altered according to enzyme dynamics 2. Correlate factors affecting rate of reaction with normal metabolic processes 3. Link factors affecting rate of reaction with abnormal conditions and diseases What are the factors affecting enzyme activity? Several physical and chemical factors affect the enzyme activity. These include: 1- Temperature The reaction velocity increases with temperature until a peak velocity is reached. The optimum temperature for most human enzymes is 37°C. Optimum temperature is the temperature in which a maximal rate of enzyme activity is achieved. At zero °C the enzyme is inactive. Between 0° and 40°C, the enzyme activity doubles with each 10°c rise in temperature. Further elevation of the temperature causes a decrease in reaction velocity as a result of temperature-induced denaturation of the enzyme. For this reason; fever and hypothermia might be life threatening conditions affecting the function of body enzymes. Figure (7-1): Effect of temperature on enzyme activity 2- PH: The concentration of protons (H+) affects reaction velocity where the catalytic process usually requires both enzyme and substrate to have specific chemical groups in either an ionized or unionized state in order to interact. For example, catalytic activity may require that an amino group of the enzyme be in the protonated form (−NH3+). This means that it will be active in the acidic medium and declines in alkaline medium. Page 1 of 4 Variable pH optimum: The pH at which maximal enzyme activity is achieved is called the “Optimum PH”. Optimum PH varies from one enzyme to another and often reflects the [H+] at which the enzyme functions in the body. For example, pepsin, a digestive enzyme in the stomach, is maximally active at pH 1-2 which is the normal PH of the stomach. Altering stomach acidity as that occurring with aging, some medications and surgeries lead to inappropriate functioning of the pepsin enzyme and maldigestion. Whereas other enzymes, designed to work at neutral pH, are denatured by such an acidic environment. For example the salivary amylase which has an optimum PH of (6-8), its action stops as soon as it reaches the stomach. Extremes of pH can lead to denaturation of the enzyme and loss of its function. PH Figure (7-2): Effect of PH on enzyme activity 3- Substrate concentration At fixed enzyme concentration, pH and temperature the activity of enzymes is influenced by increase in substrate concentration. The rate or velocity of a reaction (v) is the number of substrate molecules converted to product per unit time. Velocity is usually expressed as μmol of product formed per minute. An increase in the substrate concentration increases the enzyme activity and the rate of the enzyme-catalyzed reaction till a maximum (maximal velocity) is reached. Further increase in substrate concentration does not increase rate of reaction (Plateau). Maximal velocity (Vmax): The maximum rate of reaction when all enzyme active sites are saturated with substrate The observed ”Plateau” in the reaction rate occurs because at high substrate concentrations, the substrate molecules combine with all available active sites till the available enzymes becomes saturated (no more active sites are available). At this state the enzyme has reached its V max. Page 2 of 4 Zero Order First Order Figure (7-3): Effect of substrate concentration on enzyme activity Km is the affinity of the enzyme for its substrate, numerically it is the concentration of the substrate at which a given enzyme yields one-half its max. Velocity (½ Vmax) Km is constant and characteristic for a particular enzyme & its substrate under standard conditions. A numerically small (Low) km reflects a high affinity of the enzyme for substrate because a low concentration of substrate is needed to half saturate the enzyme to reach a velocity of ½ Vmax. On the other hand, a numerically large (high) Km reflects a low affinity of enzyme for substrate, a high concentration of substrate is needed to half saturate the enzyme. If the substrate concentration is much less than km, the velocity of the reaction is directly proportionate to substrate concentration. The rate of the reaction is said to be “First order” (figure 7-3). If the substrate concentration is much greater than Km, the velocity is constant (plateau) and equal to Vmax as all the enzymes are saturated with substrate. The rate of reaction is independent of substrate concentration and said to be “Zero order” (Figure 7-3). Variants of the same enzyme in different organs can act on the same substrate but with different Km values. For instance Glucokinase (enzyme needed for initial glucose breakdown) acts on glucose with high Km in the liver while Hexokinase (same function as glucokinase) acts also on glucose but with low Km in extrahepatic tissues. 4- Enzyme Concentration The rate of the reaction is directly proportional to enzyme concentration provided that the substrate concentration is unlimited until a maximum rate is reached. At this point any increase in enzyme concentration does not affect the rate of the reaction. Page 3 of 4 Figure(7-4): Effect of enzyme concentration on enzyme activity 5- Product concentration As product accumulates throughout the reaction, the enzyme activity decreases, this may be due to: - Change in the pH of the medium. - The product is somehow similar to the substrate, so it may compete with it to the catalytic site of the enzyme. - The product may bind to the enzyme at the allosteric site (in case of allosteric enzyme). Page 4 of 4 24 Regulation of Enzyme Activity: ILOs By the end of this lecture, students will be able to 1. Differentiate between the different regulators of enzyme activity 2. Correlate regulation of enzyme activity with body needs How can Enzyme activity be regulated? There are several means by which the activity of a particular enzyme is specifically regulated: 1- Zymogen activation Some enzymes are secreted in an inactive form that can be activated when they are required these are known as “Proenzymes” or “Zymogens”. At the site of action of these enzymes, specific peptide bonds are hydrolysed. Many of the digestive enzymes and enzymes concerned with blood coagulation are in this group e.g. Pepsinogen to pepsin, Trypsinogen to trypsin, plasminogen to plasmin. After hydrolysis when the enzyme is activated, it cannot be reconverted into proenzyme form. Zymogens are considered a protective mechanism to prevent auto-digestion of tissue producing the digestive enzymes and to prevent intravascular coagulation of blood. 2- Covalent Modification Many enzymes are regulated by covalent modification, most often by the addition or removal of phosphate groups to serine or tyrosine residues on the enzyme chain. Enzymes maybe activated by phosphorylation and inactivated by dephosphorylation or vice versa. This means that the enzyme is present in two inter-convertible forms (phosphorylated and dephosphorylated -only one of them is the active form). Figure (8-1): Covalent modification by phosphorylation and dephosphorylation 3- Allosteric Modulation Some enzymes do not only bind to substrates and inhibitors, but they also bind to “Effectors”. These Enzymes are known as “Allosteric enzymes”. Page 1 of 4 “Effectors” are small, regulatory physiologically important molecules that lead to catalytic modification by binding to the enzyme at distinct “Allosteric sites”. “Allosteric sites “are sites of the enzyme other than the active site that binds the substrate. The Allosteric sites bind to effectors and not to substrates. Allosteric effectors that increase the catalytic activity of enzyme are known as “Positive effectors”, while those that reduce it are “Negative effectors”. 4- Feedback inhibition Allosteric regulation in which end products inhibit the activity of the enzyme is called “ feedback inhibition”. Feedback regulation generally occurs at the “Committed-Step Enzyme” Committed-Step Enzyme: is the first irreversible enzyme unique to the pathway, it is not necessarly the rate limiting step. 5- Enzyme synthesis Cells can also regulate the amount of enzyme present by altering the rate of enzyme synthesis. The increase (induction) or decrease (repression) of enzyme synthesis leads to an alteration in the total number of active sites. This usually occurs under selected physiologic or pathological conditions. For example, elevated levels of insulin as a result of high blood glucose levels causes an increase in the synthesis of key enzymes involved in glucose metabolism. The induction-repression regulation is a slow long term regulation (hours to days), compared with the short term allosterically or covalently regulated changes in enzyme activity, which occur in seconds to minutes. Substances that increase the expression of the gene coding for the enzyme are called ”Inducers”, while those that decrease the gene expression are called “Repressors”. Enzymes that are in constant use are usually not regulated by altering the rate of enzyme synthesis. Inhibitors Inhibtors: Any substance that can decrease the velocity of an enzyme-catalyzed reaction is called an inhibitor A. Competitive inhibition: It is the type of inhibition occuring when the inhibitor binds reversibly to the same site that the substrate would normally occupy and, therefore competes with the substrate for that site. The Inhibitor is structurally similar to the substrate. The inhibitor binds to enzyme & forms an [EI] complex at the active site. No product is formed Page 2 of 4 In this type of inhibition the Vmax of the enzyme is not decreased, but the apparent Km is increased i.e the affinity of the enzyme to the substrate is decreased. This type of inhibition is reversible and the inhibition is removed by increasing the substrate concentration Clinical Implications: Statin drugs competitively inhibit the rate-limiting step in cholesterol biosynthesis as they are structurally similar to the natural substrate for this enzyme B. Non-Competitive inhibition: In non-competitive inhibition the inhibitor binds at different site other than the substrate- binding site leading to conformational changes in the enzyme and reversible inactivation of the catalytic site. Non-competitive inhibitors bind reversibly either to the free-enzyme or the ES complex to form the inactive complexes EI and ESI (Enzyme substrate Inhibition). Inhibitors often have no structural similarity to substrate and the inhibition is not reversed by increasing the amount of substrate. The most important non-competitive inhibitors are naturally occurring metabolic intermediates that can combine reversibly with specific sites on enzymes inhibiting it. It lowers the Vmax of enzyme but does not affect the Km as the inhibitor does not interfere with the binding of substrate to enzyme. Thus, the enzyme shows the same Km in the presence or absence of the noncompetitive inhibitor. Clinical Implications: Lead may non-competitively inhibit important enzymes in the heme synthesis pathway leading to anemia. Reducing agents as vitamin C and vitamin E can regain the activity of the enzyme. Routine supplementation of these vitamins is therefore advised for people living or working in areas of high pollution or near factories. C. Irreversible Inhibition (Enzyme poison) The Inhibitor combines covalently tightly and irreversibly to the functional group of the enzyme destroying it. At any given concentration the inhibitor will destroy the enzyme permanently, thus it’s called “Enzyme Poison”. This type of inhibition decreases the Vmax while the Km stays the same unchanged. No competition between substrate and inhibitor because the inhibitor has no structural resemblance to the substrate. Clinical Implications: Organophosphates found in most pesticides when accidently ingested or inhaled lead to irreversible inhibition of enzymes controlling nerve signals in the body. Also cyanide leads to irreversible inhibition of enzymes responsible for ATP synthesis. Therefore, low doses of cyanide present in cigarettes are responsible for many of smoking side effects. ❖ Enzymes in clinical use: A- Diagnosis of Diseases: Page 3 of 4 Plasma enzymes can be classified into two major groups: 1. Functional Plasma Enzymes: Those, relatively, small group of enzymes secreted into the plasma by certain organs and performing a certain function in the plasma For example: - the liver secretes zymogens involved in blood coagulation cascade. 2. Non-functional plasma enzymes: Those large enzyme species released from cells during normal cell turnover. These enzymes are normally intracellular and have no physiologic function in the plasma. In healthy individuals the levels of these enzymes are fairly constant and represent steady state in which the rate of release from cells into the plasma is balanced by an equal rate or removal from the plasma. Many diseases that cause tissue damage result in an increased release of intracellular enzymes into the plasma which correlates with the extent of tissue damage. Thus, the degree of elevation of a particular enzyme in plasma is often useful in evaluating the diagnosis and prognosis for the patient. For example, the enzyme Alanine aminotransferase (ALT) is abundant in the liver. The appearance of elevated levels of ALT in plasma signals possible damage to hepatic tissue. Therefore it is part of the liver function test panel. Also Alkaline phosphatase enzyme present in bone increases with diseases affecting bone as osteoperosis, rickets and bone tumors. Increases in plasma levels of enzymes with a wide tissue distribution provide a less specific indication of the site of cellular injury and limits their diagnostic value. B- Treatment of diseases: Enzymes are used in treatment of some diseases e.g. 1. Some digestive enzymes are now supplied as treatment for maldigestion. 2. Alteplase in treatment of myocardial infarctions. It enhances the activation of enzymes needed for degradation of thrombus. Page 4 of 4