Nuclear Medicine Physics Module Lecture 1 PDF
Document Details
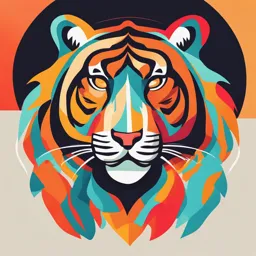
Uploaded by GloriousRhodochrosite
Dr Mohammed Saeed Alqahtani
Tags
Related
Summary
This document introduces nuclear medicine physics, covering topics like stable nuclei, isotopes, radionuclides, and their production, decay mechanisms, and applications in medical imaging. It explains the role of cyclotrons, nuclear reactors, and generators in producing radioisotopes.
Full Transcript
Nuclear Medicine Physics Module Dr Mohammed Saeed Alqahtani Lecture 1: Introduction Nuclear Medicine Physics Module Dr Mohammed Saeed Alqahtani Lecture 1: Introduction Introduction I ▪ The technologies used in nuclear medicine for diagnostic imaging have improved over the last century, starting with...
Nuclear Medicine Physics Module Dr Mohammed Saeed Alqahtani Lecture 1: Introduction Nuclear Medicine Physics Module Dr Mohammed Saeed Alqahtani Lecture 1: Introduction Introduction I ▪ The technologies used in nuclear medicine for diagnostic imaging have improved over the last century, starting with Röntgen’s discovery of X rays and Becquerel’s discovery of natural radioactivity. ▪ Each decade has brought innovation in the form of new equipment, techniques, radiopharmaceuticals, advances in radionuclide production and, ultimately, better patient care. ▪ All such technologies have been developed and can only be practised safely with a clear understanding of the behaviour and principles of radiation sources and radiation detection. 4 Radioactivity 5 2 Stable nuclei ▪ Nearly, all the nuclides extant in the world are stable. 2 ,6)8% yes%) ▪Apart from the nucleus of ordinary hydrogen, which consists of a single proton, all the stable lighter nuclei contain nearly equal numbers of protons and neutrons. →For example: Helium atom 6 #3 Isotopes ▪ Isotopes of an element are nuclides that have the same number of protons (atomic number), position in the periodic table, and chemical and metabolic properties, but a different number of neutrons, mass number (protons + neutrons), density and other physical properties. isotope = F 7 As Isotopes For example: (Carbon-12) → stable → 6 neutrons + 6 protons t 14C (Carbon-14) → unstable → 8 neutrons + 6 protons (a neutron excess) & 11C (Carbon-11) → unstable → 5 neutrons + 6 protons (a neutron deficit) & 12C - & T ▪ Both 14C and 11C are artificially produced, unstable and radioactive. 8 Radionuclides 3 ▪ Unstable nuclei are radioactive and decay until they become stable nuclei with the emission of any combination of alpha, beta and gamma radiation. ▪ Naturally occurring radionuclides, such as uranium, radon and radium, contribute to our background radiation exposure, whether external to the body or internal. 9 Production of radionuclides ▪ There are more than 2700 known radionuclides. ▪ Radionuclides used in medical imaging are produced artificially, in the following ways: - 10 Production of radionuclides A) If an additional neutron is forced into a stable nucleus → a neutron excess ▪ This process occurs in a nuclear reactor. For example, with Molybdenum: 98Mo +n→ 99Mo ▪ The atomic number of the nucleus remains unchanged, but its mass has been increased by one. 11 Production of radionuclides B) If an additional proton is forced into a stable nucleus, knocking out a neutron → a neutron deficit ▪ This process occurs in a cyclotron, which accelerates positively charged ions (protons, deuterons or alpha particles) on the target material. For example, with oxygen: A 18O +p→ 18F Z +n ▪ The mass number has not been changed, but its atomic number has increased by one to become fluorine. 12 Production of radionuclides ▪ Radionuclides produced in a cyclotron are short-lived (i.e. with half-lives ranging from less than a minute to a couple of hours). → So, it is only possible to use them reasonably close to the & cyclotron. ▪ Medical minicyclotrons have been designed specially for the production of short-lived radionuclides such as 18F) at or near the hospital site. fluorine-18 ( S & - - - - - - 110 S & - - - M mi 13 Production of radionuclides I 2 Cyclotron 3 - - - 8 Of course, Hassan! Here's a summary of how a cyclotron works: A cyclotron accelerates charged particles, like protons or electrons, using magnetic and electric fields. The particles are injected into a circular chamber and accelerated by alternating electric fields as they spiral outward. Magnetic fields guide the particles in a circular path, gradually increasing their speed with each revolution until they reach high velocities. The accelerated particles can then be used for various scientific and medical purposes. Y - & - Feel free to use this summary to help you remember the main points about cyclotrons. Let me know if you need any further clarification or assistance! G S 7 - 14 How cyclotron work 1 A cyclotron accelerate charged Particles , like Protons or electrons , using magnetic or electric field 15 Nuclear Medicine Physics Module Dr Mohammed Saeed Alqahtani Lecture 2 Production of radionuclides D ▪ There are more than 2700 known radionuclides. ▪ Radionuclides used in medical imaging are produced artificially, in the following ways: 2 Production of radionuclides 2 A) If an additional neutron is forced into a stable nucleus → a neutronAt excess ▪ This process occurs in a nuclear reactor. For example, with Molybdenum: & A exce 98Mo Z 210 +n→ an 99Mo ▪ The atomic number of the nucleus remains unchanged, but its mass has been increased by one. 3 3 Production of radionuclides B) If an additional proton is forced into a stable nucleus, knocking out a neutron → a neutron deficit ▪ This process occurs in a cyclotron, which accelerates positively charged ions (protons, deuterons or alpha particles) on the target material. For example, with oxygen: - A 18O ② g +p→ 18F & +n 2 ▪ The mass number has not been changed, but its atomic number has increased by one to become fluorine. S 4 Production of radionuclides Y ▪ Radionuclides produced in a cyclotron are short-lived (i.e. with half-lives ranging from less than a minute to a couple of hours). → So, it is only possible to use them reasonably close to the cyclotron. ▪ Medical minicyclotrons have been designed specially for the production of short-lived radionuclides such as fluorine-18& (18F) at or near the hospital site. Doin 5 Production of radionuclides. & Es Cyclotron & & 6 Production of radionuclides · 6 A T C) Radioactive fission products may be extracted from the spent fuel rods of nuclear reactors 238U → 99Mo + other fission by-products - ▪ As the molybdenum is different chemically from the other products, it can be separated and prepared in a very pure form. 7 Production of radionuclides Z D) Some radionuclides are daughter products obtained from generator that contain a longer-lived radioactive parent. ▪ Useful daughter products: Molybdenum-99 (99Mo) generator → Technetium-99m (99mTc) Germanium-68 (68Ge) generator → Gallium-68 (68Ga) - 8 Radioactive transformation (Decay) nu 9 & Radionuclides with a neutron excess (β- decay) ▪ Radionuclides with a neutron excess may lose energy and become stable by a neutron changing into a proton plus an electron. ▪ The electron is ejected from the nucleus with high energy and is referred to a negative beta particle n → p + βB- 2+ B+ z+ 10 o Radionuclides with a neutron excess (β- decay) →For example: Iodine-131 (131I) with atomic number 53, decays in this way to xenon-131 (131Xe), with atomic number 54. ▪ There has been no change of mass number, but the atomic number has increased by one. 11 18 Radionuclides with a neutron excess (β- decay) ▪ Isomeric transition → in the case of some radionuclides, the gamma ray is not emitted until an appreciable time after the emission of the beta particle. i →For example: 99Mo decays by the emission of negative beta particle, the daughter nucleus technetium remains in the excited state for a variable length of time, which averages a matter of hours. - It is said to be metastable and is written as 99mTc - 12 Il Radionuclides with a neutron excess (β- decay) Parent nuclide → emission (half-life)→ daughter nuclide ▪ * 99Tc), and the decay happens with the decay to the ground state, technetium-99 (& emission of a gamma ray of energy 140 keV. 99mTc ▪ Both 99mTc and 99Tc are said to be isomer, nuclei having different energy states and halflives, but otherwise indistinguishable as regards mass number, atomic number and other properties. half-life = 6 hours → decay to 99Tc → unstable 99Tc half-life = 211000 years → decay to ruthenium-99 (99Ru) → stable 99mTc 13 Radionuclides with a neutron excess (β- decay) D On 12 - Dude 14 Radionuclides with a neutron deficit: β+ decay or K-electron capture 13 β+ decay → Radionuclides with a neutron deficit may lose energy and become stable by a proton within the nucleus changing into a neutron and a positive electron. ▪ The positive electron is ejected from the nucleus with high energy and is referred to a positive beta particle (i.e. positron) p → n + β+ 15 Radionuclides with a neutron deficit: β+ decay or K-electron capture In → For example: Fluorine-18 (18F) with atomic number 9, transforms into oxygen-18 (18O), with atomic number 8. ▪ There has been no change of mass number, but the atomic number has decreased by one. 16 Radionuclides with a neutron deficit: β+ decay or K-electron capture 15 K-electron capture → the nucleus may increase its number of neutrons relative to the number of protons by capturing an extranuclear electron from the nearest K-shell. p + e- → n ▪ The daughter nuclide will emit K-characteristic X-rays when an electron from an outer shell fills the created in the K-shell. 17 Radionuclides with a neutron deficit: β+ decay or K-electron capture 16 → For example: Iodine-123 (123I) decays wholly by electron capture and emits 160 keV gamma and 28 keV X-rays but no positive beta particles. 18 IE Gamma rays ▪ The gamma rays emitted during radioactive decay of a given radionuclide have at most a few specific energies (forming a line spectrum) that are characteristic of the nuclide that emits them. City ▪ Gamma rays have identical properties to X-ray. - 19 10 Gamma rays →For example: Iodine-131 (131I) emits mostly D 364 keV gamma rays. - & a 20 19 Positron emitters Et "5,5 95 ▪ Positive electrons (i.e. positrons) have a very brief existence. B ▪ When a positive beta particle comes to the end of its range, it combines with a nearly negative electron. ▪ The opposite charges neutralise each other. Str ▪ The combined masses of the two electrons are wholly converted into energy. · the mass of tow &. - + " - --- I - - / 51 j- + = = D - 5. & & - o = electron into converted energy 21 Positron emitters -re ▪ According to Einstein's formula (E = m c2 ) for the equivalence between energy E and mass m, the mass of each electron is equivalent to 511 keV. ▪ When the positive and negative electrons annihilate each other, the energy is emitted as two photons of annihilation radiation (each of 511 keV) travelling in opposite directions. 50 ▪ Positron emitters are used in positron emission tomography & (PET) imaging. & emission : Position =. ) tomography PET 22 21 Positron emitters & C = & D & " 23 Radioactive decay - - Si) 22 ▪ Radioactive disintegration is a stochastic (random)process. - joj Si 5 ▪ It is impossible to predict which of the unstable nuclei in a sample will disintegrate in the next second. j - & ⑮ Sijs - j5xd 61 - od) , ▪& BUT it is possible to be quite precise about the fraction that will disintegrate on account of the large numbers of nuclei the sample contains. 24 23 Activity 194 ▪ A radioactive nucleus does not make its presence known until it decays and emits a beta or gamma rays, or both. - ▪ The quantity of radioactivity is measured not by the A ‘population’, the mass or number of radioactive atoms, D BUT by the transformation rate, i.e. the number that represents disintegrate per second (also known as the decay rate). 25 Activity 2u ▪ The activity of radioactive sample is the rate of disintegration, the amount of disintegrating per second. ▪ The SI unit is the Becquerel (Bq) = 1 disintegration per second. ▪ In medical gamma imaging, most radionuclide administrations are measured in megabecquerels (1 MBq = 106 Bq). ▪ The activity of radionuclide generators in gigabecquerels (1 GBq = 109 Bq). ▪ A unit found in old textbooks is the curie (Ci) → 1 mCi = 37MBq 37x10 26 2 E Activity ▪ When the gamma rays enter a detector, they may be registered individually as counts. ▪ The count rate [number of counts per second (cps)] measured by a given instrument is less than the activity, because the greater proportion of the rays usually miss the detector and some pass through it undetected. & ▪However, there is a proportionality: Count rate α activity α number of radioactive atoms in the -sample 2 - & & - - - - S - 27 24 Activity *▪ The fundamental law of radioactive decay states that the -- - - activity of a radioactive sample decreases by equal fractions (percentage) in equal interval time. & - - ▪This is referred to as the exponential law. - - & ▪ However long the time, the activity of a radioactive sample never falls to zero. cant be zero activity 28 27 Physical half-life #▪ The half-life (t ) of a radionuclide is the time taken for its 1/2 activity to decay to half of its original value. & → For example: * Two successive half-lives reduce the activity of a radionuclide by a factor ofDa 2 X 2 = 4. * Ten half-lives reduce the activity by a factor of& 210 ≈ 1000 29 what is the def Physical half-life Radioactive decay reduces the number of radioactive nuclei over time. In one half-life (t1/2), the number decreases to half of its original value. Half of what remains decay in the next halflife, and half of those in the next, and so on. This is an exponential decay, as seen in the graph of the number of nuclei present as a function of time. P & yu with biological and Pusical 29 ↑ X Xn ADDDAD 30 30 Physical half-life ▪ This half-life is more properly called the physical half-life. ▪ It is a fixed characteristic of the radionuclide, cannot be predicted for a given radionuclide in any way. * ▪ The physical half-life is unaffected by any other factors, such as heat, pressure, electricity or chemical reactions. ▪ It can range from fractions of a second (useless in imaging) to millennia in the case of 99Tc (also useless in imaging). - - ↑ - - - 31 3) Physical half-life ▪ Physical half-lives of some radionuclide used in medical imaging: Half-life 13 s D 1 min D Radionuclide & Rubidium-82 Krypton-81m im 10 min Nitrogen-13 20 min Carbon-11 & 68 min 110 min 6h 13 h - Tom ve Gallium-68 Dam Pip Fluorine-18 13V Iodine-123& & Radionuclide 67 h Molybdenum-99 67 h Indium-111 73 h & Thallium-201 a O P Th m Technetium-99m Half-life > 78 h Gallium-67 5 days Xenon-133 8 days & 211 000 years important on deTh Iodine-131 & Technetium-99 & NM 32 Effective half-life 3 & ▪ When a radionuclide is used in medical gamma imaging, it usually forms part of a salt or organic compound, the metabolic properties of which ensure that it concentrates in the tissues or organ of interest. & ) 6 ,9 ▪ A pharmaceutical that has been labelled with a radionuclide is referred to as a radiopharmaceutical. - - - T 33 Effective half-life 34 Z ▪ If the pharmaceutical alone is administered, it is gradually eliminated from the tissue, organ and whole body by the usual metabolic processes of turnover and excretion. - ▪ Such a process can be regarded as having a biological half-life (tbiol). - ▪ If the radionuclide bottle, its activity decays with its &is stored in a& physical half-life (tphys). - - bu 34 34 Effective half-life =>i -! psy) 3 , ▪ If the radiopharmaceutical is administered to a& patient, the radioactivity in specific tissue, D an organ or the& whole body sin decreases because of the simultaneous & effects of radioactive decay and metabolic turnover and excretion. & - - - I # - - - -- ▪ The radiopharmaceutical can be regarded as having an effective half-life (teff). > - - - 35 Effective half-life 36 Y ▪ The effective half-life is shorter than either the biological or physical half-lives. teff < tbiol , tphys 1/teff = 1/tbiol + 1/tphys ▪ The effective half-life depends on the radiopharmaceutical sig used and the organ involved, and it can vary from person to person, depending on their disease state. - 36 Radiopharmaceuticals -15295) 37 Radiopharmaceuticals B: 09 37 - ▪ Desirable properties of a radionuclide for imaging are as oh half-lif follow: the best Techa H 1. A physical half-life of a few hours, similar to the time from preparation to injection. If the half-life is too short, much more activity must be prepared than the actually injected. - cuse have - 2. Decay to a stable daughter or at least one with a very long half-life (e.g. 99Tc has half-life of about 211000 years). S = contaminating 38 Radiopharmaceuticals 38 ▪ Desirable properties of a radionuclide for imaging are as follow: 3. Emission of gamma rays (which produce the image), but not alpha or beta particles nor very low energy photons, which have a short range in tissue and deposit unnecessary dose in the patient. 4. Emission of gamma rays of energy 50-300 keV and ideally about 150 keV – high enough to exit the patient but low enough to be easy collimated and easily detected. = looker 39 Radiopharmaceuticals 39 ▪ Desirable properties of a radionuclide for imaging are as follow: energy sing) Has 5. Ideally, emission of monoenergetic gamma rays, so that scattered radiation can be eliminated by energy discrimination with a pulse height analyser (PHA). sing 6. Easily and firmly attached to the pharmaceutical at room temperature, but has no affect on its metabolism. 40 Radiopharmaceuticals No ▪ Desirable properties of a radionuclide for imaging are as follow: 7. Readily available at the hospital site. 8. A high specific activity, i.e. high activity per unit volume. 41 Radiopharmaceuticals 41 ▪ Desirable properties of a radiopharmaceutical are as follow: 1. localise largely and quickly in the target (i.e. the tissue of interest) 2. be eliminated from the body with a effective half-life similar to the duration of the examination, to reduce the dose to the patient 3. have a low toxicity 42 Radiopharmaceuticals un ▪ Desirable properties of a radiopharmaceutical are as follow: 4. form a stable product both in vitro and in vivo 5. be readily available and inexpensive per patient dose → The decay during transport and storage of a short-lived radionuclide is reduced if it can be supplied with its longerlived parent in a generator. 43 n3 Technetium generator ▪I Technetium-99m (99mTc) is used in 90% of radionuclide imaging, as it fulfils most of the mentioned criteria. ▪NWith its gamma energy of 141 keV, it is easily collimated and easily absorbed in a fairly thin crystal, thus giving good spatial resolution. ▪3 With its short half-life (6 h) and pure gamma emission, a reasonably large activity can be administered, reducing noise in the image. - - 44 Technetium generator u5 ▪ Technetium-99m (99mTc) is supplied from a generator shielded with lead. ▪ The generator contains an exchange column of alumina beads on which have been absorbed a compound of the 99Mo (which can be produced in a nuclear reactor and parent & has a 67 h half-life). - & S 45 Technetium generator ub & Schematic of Technetium-99m generator e % 46 ni Technetium generator ~ Y ▪ When the generator is delivered, the activity of the daughter -equal to that of the parent 99mTc has built up to its maximum, 99Mo. 4▪ The daughter is decaying as quickly as it is being formed by the decay of its parent. & ▪ The daughter and the parent decay together with the halflife of the parent (67 h). - & - - - 47 v 48 Technetium generator Di i & Decay of activity of molybdenum-99 and growth and regrowth of activity of technetium-99m in a generator that is eluted daily. o & 2 + 24 + 24 - + 24 + 22 48 Technetium generator u C 49 ▪ The technetium-99m is washed off the column (eluted) as sodium pertechnetate with sterile saline solution. V ▪ This flows under pressure from a reservoir through the column into a rubber-capped sterile container. I ▪ The precise design of the generator and pressure system depends on the manufacturer. -15) 8 ▪ Elution takes a few minutes and leaves behind the molybdenum, which is firmly attached to the column. -55 % , 49 40 Technetium generator & ▪ As you have seen in the previous figure, the 99mTc in the column increases with the same half-life of 6 h. ↑ Gh 1 ▪ After 24 h, the activity has grown again to a new maximum (equilibrium) value. 50 i ▪ Elution can be made daily, although it will be seen that the strength of successive eluents diminishes in line with the decay of 99Mo. i ▪ After a week, the generator is usually replaced and the old one is returned for& recycling.. 50 Uses of technetium-99m Se & X ▪ Sodium pertechnetate- 99mTc is used for imaging the tissues: - 5 =d , → the thyroid, the gastric mucosa (localisation of Meckel’s diverticulum) and the salivary glands. %=13575.. 99 79 → Blocked from the thyroid by administration of potassium perchlorate, it can be used for cerebral blood flow and testicular imaging and mixed with bran porridge for gastricemptying studies. 51 next Sele natur ▪ 99mTc can easily be labelled to a wide variety of usual Uses of technetium-99m compounds, for example : →methylene diphosphonate & (MDP): for bone imaging → hexamethyl propylene amine oxime (HMPAO): for cerebral imaging → dimercaptosuccinic acid (DMSA) and mercaptoacetyltriglycine (MAG3): for renal studies → iminodiacetic acid (HIDA): for biliary studies 52 Uses of technetium-99m Sit → human serum albumin (HSA): imaging of liver, spleen and red bone marrow → diethylene triamine pentacetic acid (DTPA) aerosol (5µm particles): for lung ventilation studies → autologous red cells: for cardiac function AR HDARC → heat-damaged autologous red cells: for imaging the spleen → sestamibi or tetrofosmin: for cardiac perfusion imaging sot 53 other radionuclides and their uses 68 D ▪ Iodine is trapped and metabolised by the thyroid, which was 131I was the first radionuclide the first organ ever imaged, and D used for imaging. ⑬ & D → 131I was cheap, highly reactive and an excellent label. → D131I is produced in a nuclear reactor and has a long half-life (8 days), but emits beta particles as well as energetic gamma rays (mainly 364 keV). & 364 I 54 other radionuclides and their uses S → 131I has largely been replaced for imaging by 123I, which is more expensive, cyclotron produced, has a half-life of 13 h, and decays by electron capture emitting 159 keV gamma rays. → 123I is also more expensive than, but otherwise superior to, 125I with its long half-life (60 days) and low photon energy (35 keV) → Note that both 131I and 125I are used for therapy: - 131I is used for thyroid ablation - 125I is used as brachytherapy seeds jem 2 55 other radionuclides and their uses 60 ▪ Xenon-133 (133Xe): is produced in a nuclear reactor, has a half-life of 5.2 days and emits beta particles and low energy gamma rays (81 keV) → It is an inert gas, although somewhat soluble in blood and fat, and is used, with rebreathing, in lung ventilation imaging. 3 56 other radionuclides and their uses 61 ▪ Krypton-81m (81mKr): another inert gas, is generatorproduced, has a half-life of 13 s and emits 190 keV gamma rays. →The generator is eluted with compressed air, and the patient inhaling the air (i.e. 81mKr mixture) in pulmonary ventilation studies. → The parent, Rubidium-81 (81Rb), has a short half-life (4.7 h), which presents transport difficulties and means that it must be used the day it is delivered. V 57 other radionuclides and their uses 62 ▪ Gallium-67 (67Ga): is cyclotron-produced, has a half-life of 67 h, and decays by electron capture, emitting gamma rays of three main energies (93, 184, 296 and 388 keV). → Gallium citrate is used to detect tumours and abscesses. E 58 other radionuclides and their uses 63 ▪ Indium-111 (111In): is cyclotron-produced, has a half-life of 67 h, and decays by electron capture, emitting 171.3 keV and 245.4 keV gamma rays. → It is used to label white blood cells and platelets for locating abscesses and thrombosis. ↳ 59 other radionuclides and their uses 64 ▪Indium-113 (113mIn): is sometimes used instead of 111In, is generator-produced, has a half-life of 100 min, and emits only gamma rays, but they have a high energy (390 keV). 7 60 Positron emitters ba ▪ Positron emitters are needed for PET (positron emission tomography) scans. ▪ The most common PET radionuclide is 18F, with a half-life of 110 min. ▪ 18F often used in the form of 18F 2-fluroro-2deoxyglucose (2FDG) for brain and heart metabolism as well as eplipsy and tumour detection. I 61 Positron emitters 66o ▪ Other radionuclides used for PET are carbon-11 (11C), nitrogen-13 (13N), oxygen-15 (15O) and rubidium-82 (82Rb), but these positron emitters have very short half-lives: 20 min, 10 min, 2 min and 75 s, respectively. ▪ 82Rb, which is produced from a strontium-82 generator that lasts about 1 month, can be used for myocardial prefusion imaging. 2 62 Positron emitters -67 ▪ Technetium-94m (94mTc), cyclotron-produced with a half-life of 50 min. & → It can be used to label many pharmaceuticals already available for 99mTc imaging. → 94mTc sestamibi can be used in tumour imaging studies utilising PET. j 3 63 64 Nuclear Medicine Physics Module Dr Mohammed Saeed Alqahtani Lecture 3 · I Preparation of radiopharmaceuticals 2 & Preparation of radiopharmaceuticals FG= = = ⑨ & * The preparation usually involves the simple mixing or shaking at room temperature of the radionuclide (e.g. 99mTc as sodium pertechnetate) with the compound to be labelled (e.g. MDP) and other necessary chemicals. jg gi join& , je Shielded syringes are used to transfer the components between sterile vails. 9) - S The manipulations are carried out under sterile conditions in a workstation; for example, a glove box or a sterile laminar down-flow cabinet. Tol P * ) ist tailing 3 Preparation of radiopharmaceuticals & Laminar down-flow cabinet A glove box 4 2 Preparation of radiopharmaceuticals & 2 All surfaces are impervious: continuous floors, gloss-painted walls, and formicatopped or stainless steel benches. I 2 Normal sterile procedures must be followed, and entry is via an air lock and changing room. With preparation time being necessarily short for PET radiopharmaceuticals, & automated synthesis devices using microprocessor control are commonly used. Using this technology reduce the radiation exposure of the staff ↓ T 5 Preparation of radiopharmaceuticals Automated synthesis device for PET tracers’ production 6 3 Radiopharmaceuticals quality control Quality control includes testing for: A) radionuclide purity: Y 55 For example, testing for contamination with 99Mo, which would give an unnecessary dose to the patient, by measuring any gamma radiation from 99Mo after blocking off the gamma rays from 99mTc with 6mm lead. 7 Y Radiopharmaceuticals quality control Quality control includes testing for: B) radiochemical purity: For example, testing for free pertechnetate in a labelled 99mTc compound using chromatography. & C) chemical purity: For example, the spot colour test for alumina, which may have come from the 99Mo column and would interfere with labelling. 8 E Radiopharmaceuticals quality control Quality control includes testing for D) response of the radionuclide calibrator: For example, testing the detector against a standard radioactive source with a long half-life. - 9 Dose calibrator b 10 Planar imaging 11 Planer imaging process - T The patient is given an appropriate radiopharmaceutical, usually by intravenous injection. (b)gj4)) The function of the radiopharmaceutical is, ideally, to concentrate in the organ or tissues of interest. The role of radionuclide is to signal the location of the radiopharmaceutical by the emission of gamma rays. 12 Planer imaging process * The radionuclide most commonly used, 99mTc, emits 141keV gamma rays. The emitted gamma photons are detected by a gamma camera, and an image of the radioactive distribution is produced on a monitor screen. As gamma rays cannot be focused, instead of a lens a multi-hole collimator is used to delineate the image from the patient. 13 er Large field of view (LFOV) gamma cameras Diversified hardware and connections are assembled together to form a medical gamma ray detection instrument, also known as the gamma camera. Each component plays an integral role in the identification of gamma photons and producing proper medical gamma images. These slides elaborate on the conventional gamma imaging system’s chief elements only and does not provide an explanation of every single component and their detailed functions. D 1 Shildin X Collomitar - i - scrclition Radig light gude - - PMT > - - - electrones Computer display 14 D LFOV gamma imaging system D The major components observed in each gamma camera I 3 include a light guide, photomultiplier tube array, radiation * ⑤ $ shielding, scintillation crystal, collimator, electronics for energy positioning and differentiation. Additionally, there a ⑦ would be a display device and a computer for processing and demonstrating images and data ~ 2 - ~ - - - ~ - & 15 " LFOV gamma imaging system 16 LFOV Gamma Camera Scintillators 12 DThe process of converting gamma rays into optical light is performed using a scintillation crystal. As a part of a gamma camera design, several characteristics are considered before choosing the appropriate crystal for gamma ray detection. To attain enhanced imaging performance suitable for clinical use, the scintillator chosen must be inexpensive, have short decay duration, with high atomic number, and high density and light output. ~ 17 LFOV Gamma Camera Scintillators the following table lists properties of common scintillators used in conventional gamma cameras: 13 Properties Na(Tl) CsI(Na) CsI(Tl) Density (g cm-3) 3.67 4.51 4.51 Decay time (µs) 0.23 0.63 1 Refraction Index Photon yield (keV) 1.85 38 1.84 39 1.8 45 – 52 Hygroscopic Yes Yes Slightly Effective atomic number (Zeff) 50 54 54 420 540 Peak emission wavelength (nm) 415 & 18 LFOV Gamma Camera Scintillators 2 jet Sodium iodide & (NaI) scintillators are the most widely utilised for radiation detection in conventional gamma ray imaging systems. - However, it has been found that NaI crystals have unwanted characteristics of gudi moisture absorption and fragility; therefore, they are required to be sealed in airtight , containers.-(j) - & -g) To enhance the NaI crystals reaction to the incident gamma photons, they can be ,. doped with thallium (Tl) atoms, which improves the scintillator’s response to the gamma photons. & in 19 D LFOV Gamma Camera Scintillators 1s As a result of photoelectric or Compton scattering interactions of the incident photons with the scintillator’s material, an energetic electron is released and moves within the scintillator and interacts with other atoms to produce more excitations and ionisations. The resultant electrons that are in an excited state subsequently return to their stable state through realising their energy in a form of optical photons. 20 LFOV Gamma Camera Scintillators If Crystal design is an integral factor influencing the scintillation process. It has been shown that consistently high sensitivity levels are recorded while utilising thick crystals, as they can absorb most incident gamma photons; however, a reduction of spatial resolution is recognised. thin Conversely, gamma photons can escape thinner crystals easily, which will degrade the sensitivity of the detector. 21 LFOV Gamma Camera Scintillators 175 For medical imaging purposes, the usual thicknesses for the manufactured scintillators of gamma cameras is in the range 6 to 12.5 mm, and these thicknesses match the detection of gamma photons in the 141 keV range (i.e. 99mTc isotope energy). MTC 22 & LFOV Gamma Camera Scintillators " If 7 23 Photomultiplier tube (PMT) Z 19 There are two steps in the procedure of gamma detection using - gamma cameras. The process commences with an interaction between the scintillation crystal and the incident gamma photons. Crystal tillation 5555 Following this, the energy of these photons is deposited in the crystal and converted to visible optical photons. The transmitted light But propagates and is guided towards an array of PMTs. > -Photons > - 24 20 Photomultiplier tube (PMT) The PMT is a high-voltage, sensitive, optical detector, which can detect and convert optical photons into a measurable electrical signal. * desir3 javijade The PMT works on the directly proportional relationship between the electric current and the number of detected optical photons. - , - D ⑰ the PMT is a vacuum tube with an entrance window, an anode, photocathode, dynodes (electron multiplier), and focusing electrodes. ③ D ⑬ 25. Photomultiplier tube (PMT) I 44 The NaI scintillator transmits optical photons at a peak wavelength of 415 nm and these optical photons are received by the photocathode, which liberates photoelectrons (i.e. low energy phenomena: photoelectric effect). The photoelectrons, produced by the photocathode, are directed by a focusing electrode towards the first dynode. photons PhotocatioFocusinto & N - de demon The collision between the primary electrons and the first dynode surface liberates more electrons. Consequently, the same step repeats between a series of dynodes with further stages of electron amplification. 26 Photomultiplier tube (PMT) 22 This process does not end until the electrons reach the final dynode. This cascade procedure works as all the electrons are collected at the PMT’s anode. 27 Photomultiplier tube (PMT) 23 · -. 28 ionizationa I High energy Photonsume /T Photocatod Prim Kum low energy Photon * goynade - Fusingsee secondre E & & ↓ f To + Y -I = Ti Y PMT ionization Track sintillator N [ m Phimry elefror ↑ High energy · ↑ me - 33 d Secondre Cathode ↓ Photo · electron low energy Photons > - esing electron o trode camer Pens ey Gamma ray collimation principles The gamma image produced by a conventional gamma camera is formed on a pixelated grid. The number of detected gamma photons is recorded for each pixel within the camera’s field of view. -G Therefore, the formation of a gamma image is actually a true mapping for the radioactive agents in the targeted region of interest (i.e. the spatial locations of the detected gamma photons). 29 Gamma ray collimation principles 25 To enhance the produced gamma image quality, scattered gamma photons should be minimised to reduce image noise. ① D Additionally, improving the ability of the gamma camera to maximise the detection of primary gamma photons would improve the gamma imaging outcome. * - 30 Gamma ray collimation principles 26 When the process of nuclear scanning takes place, there are several elements that influence the appropriate type of collimator for gamma ray detection. Such as FOV dimension, targeted areas’ dimensions, and the desired level of sensitivity and spatial resolution. the gamma rays that are emitted from the examined region in the patient are mapped onto the scintillator and represent the targeted tissues’ respective locations in the image. The main drawback of this approach is that only gamma photons passing through the collimator’s holes can be registered and utilised to produce a gamma image; the other gamma photons will be absorbed by the collimator’s material. Therefore, the sensitivity of the gamma camera is affected. The following slides show routinely used gamma camera collimators in nuclear medicine clinics. - 31 Gamma ray collimation principles 22 - pRiging 32 Diverging Hole Parallel Hole image- - Image - Scintillator 11119(11/12/11) DC (IIII object same object - ////xyNIV object - size pinhole image scintillator &S Demagnified be inverted image image Converging · Hole -Image- , i n gi l a t o r 177 /// - magnified -- object magnified image - · & P &Il - > Scintillator N J & object Demagnified size Pinhole mean ta Immage I same obj n Diverging - Non-P image conversing image Hole / obj magnified image e Parallel hole collimator 29 , The most common collimators for gamma image formation are the parallel hole collimators. This type of collimator consists of a high density material plate (e.g. lead is a commonly used material) with hexagonal structured holes arranged in a d close-packed hexagonal array. Using this preferred hole structure and arrangement would maximise the exposed area of the gamma camera detector. However, these holes can be manufactured in other structures, such as triangular, square, or circular holes. 33 302 Parallel hole collimator Generally, the thickness of parallel hole collimators manufactured for traditional gamma cameras ranges between 25 and 80 mm, and they contain 3×104 - 9×104 90000 holes. 20000 = The structure of the parallel bore arrays is symmetrical, with identical holes, surrounded by lead septa. 99 : i 34 Parallel hole collimator 3 The parallel hole collimator allows incident gamma photons to pass through at a perpendicular angle... Parallel hole collimators provide a superior level of sensitivity compared to pinhole collimators. However, generally collimators impose considerable limitations on the FOV of the gamma imaging systems because most of the off-axis gamma photons are absorbed by the collimator body. 35 Parallel hole collimator 344 In practice, the parallel hole collimator allows several incidence angles because the hole diameter is not infinitesimally small and the spatial resolution quality of the gamma camera degrades with larger dimensions of the collimator’s holes, as these will allow a wider range of acceptance angles. Using different gamma emitters also plays a significant role in the selection of the appropriate parallel-hole collimator; the energy of the gamma photons is the key factor to determine the septal thickness. This is because the scattered gamma photon penetration for the collimator body must be minimised using an appropriate septal thickness to avoid the projection of inaccurate data on the gamma image. 36 Non-parallel hole collimator 34 Parallel hole collimators differ from diverging and converging collimators based on the field of view despite their corresponding exit plane dimensions. The converging collimator gives a smaller FOV (used to scan small targeted z * areas such as during brain or cardiac scanning procedures); whereas, the diverging collimator provides a larger FOV in comparison to the parallel-hole collimator. Draw Diverging-hole collimators are utilised to collect gamma photons from a large region of interest (i.e. larger than a camera’s crystal) Brex 37 Non-parallel hole collimator yar To satisfy the needs of nuclear medicine clinics with small organ imaging procedures, such as parathyroid and thyroid scanning, pinhole collimators are appropriate. The pinhole collimator has a cone-shaped structure made from metals, such as tungsten, lead or platinum. 3 id 2 & The aperture has a diameter range of 2 to 6 mm. It further demonstrates its effectiveness in examining bone joints, paediatric scans, and skeletal extremities nuclear scanning. Draw 38 Non-parallel hole collimator 357 The spatial resolution requirements are different (i.e. higher spatial resolution is required) for small animal gamma imaging systems as the small targeted structures require 1-2 mm pinhole diameter or even lower. 39 Non-parallel hole collimator & / 3 The spatial resolution requirements are different (i.e. higher spatial resolution is required) for small animal gamma imaging systems as the small targeted structures require 1-2 mm pinhole diameter or even lower. 40 Non-parallel hole collimator & & 3 The spatial resolution requirements are different (i.e. higher spatial resolution is required) for small animal gamma imaging systems as the small targeted structures require 1-2 mm pinhole diameter or even lower. 41 Types of collimator ·. no Low-energy collimator has thin septa (0.3 mm) and can be used with gamma rays of up to 150 keV (e.g. with 99mTc). 2x(0 A general purpose collimator might have 20000 holes each 2.5 mm diameter, a resolution (at 10 cm from the face) of 9 mm, and sensitivity 150 cps MBq-1. 42 Types of collimator 41 A high-resolution collimator would have more and smaller holes and lower sensitivity; It can be used where high resolution is required and the amount of radioactivity and the imaging times needed are acceptable. - 43 Types of collimator n2 A high-sensitivity collimator would have fewer and larger holes and poorer resolution. It can be used in dynamic imaging where short exposure times are necessary and the poorer resolution must be accepted. 44 Types of collimator 43 Medium-energy collimators, for use up to 400 keV (e.g. with 111In, 67Ga and 131I), have thicker septa (1.4 mm) and consequently fewer holes and lower sensitivity. 45 45 Conventional planar gamma imaging produces a twoTomography with radionuclides dimensional projection of a three-dimensional distribution of a radiopharmaceutical. The images of organs are superimposed, depth information is lost and contrast is reduced. These deficiencies are addressed in emission tomography. 46 46 Tomography with radionuclides There are TWO methods of emission tomography: A) Single-photon emission computed tomography (SPECT) B) Positron emission tomography (PET) - 47 Single-photon emission computed tomography (SPECT) UT In its simple form, a gamma camera with a parallel hole collimator rotates slowly in a circular orbit around the patient lying on a narrow cantilever couch. - resso Stop-iggj -25 Every 6°, the camera halts for un 20 – 30s and acquires a view of the patient. 300 · ---s : 36 60x6 30x601800 60 views are taken from different directions, each, however, comprising fewer counts than in conventional static imaging. -Y 48 Single-photon emission computed tomography (SPECT) SPECT B Y ⑳ > - Some 3 million counts are acquired in an overall scanning time of around & 30 mins. 60 x30 = 194 1800s - 6 = 30min &i Image acquisition time is& halved or the sensitivity improved by using a dual- or triple-headed camera. un & - ﯾﺘﻢ ﺗﻘﻠﯿﻞ وﻗﺖ اﻟﺤﺼﻮل ﻋﻠﻰ اﻟﺼﻮر إﻟﻰ اﻟﻨﺼﻒ أو ﺗﺤﺴﯿﻦ اﻟﺤﺴﺎﺳﯿﺔ ﺑﺎﺳﺘﺨﺪام ﻛﺎﻣﯿﺮا ﻣﺰدوﺟﺔ أو ﺛﻼﺛﯿﺔ اﻟﺮأس. 49 Single-photon emission computed tomography (SPECT) 49 The camera must move on a sufficiently& large circular orbit to miss the patient’s shoulders. - ⑳ # &&S. An elliptical orbit is sometimes used to minimise the gap between the collimator and the patient and so improve the resolution. Rotation of 180° is useful option (e.g. in cardiac tomography). ↳ 50 Single-photon emission computed tomography (SPECT) So 3 M Some dedicated equipment uses three or four gamma cameras equally spaced around the rotating gantry, thus improving sensitivity. - S - - Inste The increase sensitivity can be used for faster patient throughput or to improve the resolution. 5587 - - - T / ﯾﻤﻜﻦ اﺳﺘﺨﺪام اﻟﺤﺴﺎﺳﯿﺔ اﻟﻤﺘﺰاﯾﺪة ﻟﺰﯾﺎدة ﺳﺮﻋﺔ إﻧﺘﺎﺟﯿﺔ اﻟﻤﺮﯾﺾ أو ﻟﺘﺤﺴﯿﻦ اﻟﺪﻗﺔ. SPEC 51 Single-photon emission computed tomography (SPECT) S 1733] SPECT studies can be presented either as a series of slices or as a three-dimensional display. * 3D The 3-D display is particularly effective when the image is rotated continuously on the computer screen, as persistence of vision helps to reduce the effect of image noise. 52 Single-photon emission computed tomography (SPECT) 52 Thallium studies of myocardial infarctions and ischaemia are major uses of SPECT, along with quantitative cerebral blood flow. - Other applications, such as the detection of tumours and bone irregularities, are increasing in clinical usefulness, particularly when SPECT is combined with CT. -5) -= 53 Single-photon emission computed tomography (SPECT) 43 Gated acquisitions are also possible with SPECT as in planar imaging (e.g. MUGA studies). Cardiac gated myocardial SPECT can be used to obtain quantitative information about myocardial perfusion, thickness of the myocardium, left ventricular ejection fraction, stroke volume and cardiac output. Indeed, SPECT is probably the clinical standard for assessment of myocardial ischaemia, although PET mow offers more accuracy and better spatial resolution. 54 55 Nuclear Medicine Physics Module Dr Mohammed Saeed Alqahtani Lecture 4 Single-photon emission computed tomography (SPECT) SPECT/CT machine, which is used for daily nuclear medicine procedures Preclinical SPECT/CT machine, which is used for small animal studies 2 Positron emission tomography (PET) 18F. The most common positron emitter utilised in PET is& - - When 18F emits a positive beta particle (positron), this travels for about 2 mm through the patient before being annihilated by a negative electron. Their combined mass (positron + electron) is converted into two energetic photons, each of exactly 511 keV, emitted simultaneously and in practically opposite directions. 3 Positron emission tomography (PET) A positron or PET camera comprises a ring, hexagon or other polygon surrounding the patient and composed of a very large number of solid scintillation detectors (10000-20000). These detectors often made of bismuth germinate (BGO). Other efficient scintillators, such as lutetium oxyorthosilicate (LSO) or gadolinium oxyorthosilicate (GSO), are also in use. 4 Positron emission tomography (PET) The ideal choice, from the previously mentioned scintillators, would be readily available, inexpensive to produce, and easy to manufacture into crystal blocks, with: A) High detection efficiency – to absorb and convert keV photons into optical light. B) Very short scintillation decay time C) Good energy resolution 5 Positron emission tomography (PET) As usual, a compromise is necessary; the following table shows a comparison with sodium iodide doped with thallium, which is normally used in gamma cameras: Property of the material BGO LSO GSO NaI(Tl) Effective atomic number Relative light output Decay time Typical energy resolution at 511 keV 75 15 300 ns 25 % 66 75 40 ns 25 % 59 25 60 ns 15 % 51 100 230 ns 10 % % 6 Positron emission tomography (PET) 7 Positron emission tomography (PET) Positron emission tomography detectors are commonly made in block format, coupled to photomultiplier tubes. Note that the detector material, configuration, number of detector blocks and number of photomultiplier tubes depend on the manufacturer, but the principles of operation are the same. 8 Positron emission tomography (PET) 2 I ? - - & Y 8.. - & /.... - , ↑ & I - - / S - 9 Dinin & ↑ cuts S sinticfilt ator ↓ with matira inter dement sits into lock I S Siminitiator crystal Relative Even X = bilker Positron emission tomography (PET) Y · If, as in the beside figure, the annihilation photons from the event (a) enter detector A and B, they produce simultaneous (coincident) pulses, which are then accepted and combined by the electronics. Any pulses that do not coincide in the time are ignored by the electronics, as are any single photons of background radiation. S 10 Positron emission tomography (PET) These two detectors therefore measure only the sum of the activity present along a line AB, called the line of response (LOR). Each detector can operate in coincidence with perhaps half of the detectors that face it in the ring, so that the patient is criss-crossed by hundreds of LORs. 11 Positron emission tomography (PET) There are three types of coincidences that can occur: a) True b) Random – from independent events that may nor occur in the same plane (ring). c) Scattered – that may also be cross-plane. 12 Positron emission tomography (PET) To reduce the random and scatter events from adjacent rings, narrow lead or tungsten septa (b), which is about 1 mm thick by up to 10 mm deep. comi-y & tenausten I man seet thecnk Som nm , f It can be used between each ring of detectors to act like a parallel antiscatter grid in radiography. 13 Positron emission tomography (PET) The main positron emitter used in PET imaging is 18F (half-life 110 min), primarily used to label deoxyglucose (FDG). Other useful radionuclides are 68Ga (68 min) and 82Rb (1 min), particularly as these two are produced by radionuclide generator. 14 Integrated SPECT/CT and PET/CT All gamma images give functional and physiological information. To be able to locate and visualise this information within the patient’s anatomy, PET (and SPECT) images are usefully fused with CT images or MRI images. Combining two sets of such digital images is often complicated by the need for adjustment of matrix size, matching rotational position, the transverse plane and coregistration to enable one to one spatial matching of the images. 15 Integrated SPECT/CT and PET/CT To overcome these complications, integrated SPECT/CT and PET/CT systems are used. Both detection systems are mounted on the same support, adjacent to each other, so that the single patient table moves along the central axis. Once the CT scan is complete, the patient table moves into position for the SPECT or PET data collection. Almost perfect matching of the images is obtained, except for involuntary patient movement. 16 Integrated SPECT/CT and PET/CT The combined images are particularly useful in oncology, both for diagnosis and for accurate tumour localisation and follow up. Carefully gating image acquisition to the cardiac cycle can also produce useful fused images in cardiology. 17 Dose to the patient 18 Dose to the patient Administrating a radioactive material to the patient necessarily gives the patient a radiation dose. the organ of interest that takes up the radionuclide (referred to as the source organ) will receive a dose, but it also acts as a source of irradiation for other socalled target organ and tissues in the body. 19 Dose to the patient An example of the source and target organs in lung ventilation studies is shown in the beside figure: 20 Dose to an organ The absorbed dose delivered to an organ by the activity within it increases in proportion to: the activity administered to the patient the fraction taken up by the organ the effective half-life of the activity in the organ the energy (keV) of beta and gamma radiation emitted in each disintegration 21 Dose to an organ It is also depends on how much of that energy escapes from the organ, and so does not contribute to the absorbed dose within the organ but will irradiate other tissues. Almost all the energy of beta ray is deposited inside the organ, and very little escapes. Some of the energy of gamma ray is deposited in the organ and some leaves it, to an extent depending on the size of the organ and how energetic the gamma ray is. 22 Effective dose to the body X Unlike imaging with X-rays, the dose delivered by a radionuclide examination is unaffected by the number of images taken, neither it is confined to the region of diagnostic interest. 1,61 ; /1 After an intravenous injection, most tissues may receive some dose, but the organs of interest and the organs of excretion generally receive the highest doses. 23 Effective dose to the body The distribution of a dose is non-uniform and specific to the examination, but an average dose to the body as a whole can be calculated to give a measure of risk. This is termed the effective dose (E), which has the unit sievert (Sv). It is calculated using the differing sensitivities of the various organs and tissues to irradiation, by weighting each organ absorbed dose with a tissue-weighting factor (WT) to produce an equivalent dose. These are then summed to give the effective dose per MBq activity administered. * 24 Typical activities and doses Most nuclear medicine investigations deliver an effective dose of less than 5mSv, which is of the order of the variation from place to place and individual to individual, in the annual dose of natural radiation. Some procedure, such as bone or static brain imaging, deliver doses in the region of 5mSv. A few examinations, such as tumour or abscess imaging with 67Ga, deliver higher doses and should be undertaken when other imaging modalities are inappropriate. jiy s , 25 Typical activities and doses Some typical radionuclide administrations for adults and consequent effective doses Site Agent Activity (MBq) Effective dose (mSv) Bone Technetium-99m diphosphonates 600 5 Lung ventilation Technetium-99m DTPA aerosol Krypton-81m gas 80 6000 0.5 0.1 Lung perfusion Technetium-99m HSA macroaggregates 100 1 Kidney Technetium-99m DTPA gluconate Technetium-99m MAG3 300 100 2 0.7 Infection Gallium-67 citrate Indium-111 leucocytes 150 20 15 7 Tumour Iodine-123 MIBG 400 5 Thyroid Iodine-123 iodide 20 4 Heart Technetium-99m MIBI Thallium201 chloride 400 80 3 18 Brain Fluorine-18 FDG 400 8 26 Typical activities and doses The activity should be checked and recorded before administration. The vial is placed in the ‘well’ of a large re-entrant ionisation chamber the radionuclide or dose calibrator. The accuracy of the dose calibrator must be checked regularly using a reasonably long-lived source, such as 57Co. In order to minimise patient dose, patients should drink a good deal of water and empty the bladder frequently to reduce the dose to the gonads and pelvic bone marrow. 27 Typical activities and doses As fetal doses should also be constrained, various guides recommending that female patients should avoid conception for an appropriate period following administration of longlived (half-life more than 7 days) diagnostic radionuclides. Male patients do not need to be given any particular advice concerning diagnostic examinations. 28 Typical activities and doses If a female patient is or may be pregnant, certain examinations may result in a fetal dose greater than 10 mSv and should be avoided. Equally, if a patient is breast feeding, her infant will be exposed to a radiation dose from the activity secreted in her milk. An interruption in feeding may be recommended, and advice should be sought. 29 -5 Precautions necessary in handling radionuclides. xi When handling radionuclides, in addition to the external hazards from external radiation, there is a potential hazard from internal radiation due to accidental ingestion or inhalation of the radionuclide or its entry through wounds. I It is therefore important to avoid contamination of the environment, the workplace and persons, and to control any spread of radioactive materials. Generally, the risk from contamination id greater than that from external radiation. 30 Precautions necessary in handling radionuclides – Segregation A nuclear medicine facility must have identified and preferably separate areas for: j - -S & , & - ⑤ Perpation of the preparation and storage of radioactive materials - the injection of the patient -di patients to wait (to allow uptake of the radiopharmaceutical into the organ of interest) imaging temporary storage of radioactive waste 31 Precautions necessary in handling radionuclides – Segregation Patients containing radioactivity are a source of external radiation. TF They should be spaced apart in the waiting area. TF Departmental layout should make use of the inverse square law to reduce the effect of background radiation from other patients and sources, particularly in the imaging area. 32 Precautions necessary in handling radionuclides – Personal protection Use should be made of distance, shielding and time. Staff should enter areas where there is radioactivity only when it is strictly necessary. All procedures must be carried out expeditiously and efficiently. Radionuclides are contained in shielded generators or in bottles inside lead pots. Where feasible, bottles and syringes are handled with long-handled forceps (tongs). 33 Precautions necessary in handling radionuclides – Personal protection Manipulations, such as the labelling of pharmaceuticals and the loading of syringes, are carried out with the arms behind a lead barrier that protects the body and face, and over a tray, lined with absorbent paper, to catch any drips. Syringes are protected by heavy metal, tungsten or lead glass sleeves # (which can reduce radiation doses to the fingers by 75%), and are carried to the patient in special containers or on a disposable tray. 34 Precautions necessary in handling radionuclides – Personal protection Before injection, syringes are vente into swabs or closed containers and not into the atmosphere. To avoid accidental ingestion, waterproof (double latex) surgical gloves are worn when handling radionuclides. Cuts and abrasions must be covered first. There must be no eating, drinking or facial contact. Hands and work surfaces are routinely monitored for radioactive contamination, and the air in radiopharmacies should be also monitored. 35 Precautions necessary in handling radionuclides – Personal protection Staff may also be monitored for internal contamination. Swabs are taken from the radiopharmacy workstation to monitor for radioactive and bacterial contamination. Note that lead-rubber aprons are ineffective against the high-energy gamma rays of 99mTc. Hands should be washed regularly at special washbasins. Wherever there is a spillage, however slight, decontamination procedures must be followed. 36 Precautions necessary in handling radionuclides – Patient protection # Every radionuclide should be checked for activity before administration, using a radionuclide (well) calibrator. The patient identity must be checked against the investigation to be made and the activity to be administered, and this must be recorded. Particular care should be taken to avoid contamination during oral administrations. Special circumstances apply for pregnant patients and those with babies whom they are breast feeding. 37 38 Nuclear Medicine Physics Dr Mohammed Saeed Alqahtani Lecture 5 Nuclear Oncology 2 Background Nuclear medicine has a major role in the management of malignant tumors. With the developments toward molecular imaging and the advancement of equipment used for imaging particularly after combining the nuclear medicine instruments with morphologic modalities, it has even become a more integral part of management protocols. This role includes detection of malignant tumors, staging and restaging of the disease, early detection of recurrence, evaluation of the response to therapy, and prediction of the prognosis. Additionally sentinel node identification and localization for biopsy is another important use of nuclear medicine to help plan the most suitable management for several tumors. 3 Background The main tumor imaging radiopharmaceuticals can be summarized as shown in the following table: Nonspecific agents Specific agents 67Ga 131I /123I 201TI 111In / 99mTc - Octreotide 99mTc - Sestamibi Monoclonal antibodies 99mTc - MDP 131I /123I 18F MIBG - FDG 4 Clinical Uses Diagnosis of Tumors Pretreatment staging of malignant disease Detection of residual or recurrent disease Evaluating response to therapy Radiotherapy planning Sentinel lymph node localization ▪ تشخيص األورام ▪ مرحلة املعالجة املسبقة لألمراض الخبيثة ▪ الكشف عن األمراض املتبقية أو املتكررة ▪ تقييم االستجابة للعالج ▪ تخطيط العالج اإلشعاعي ▪ توطني العقدة الليمفاوية الحارسة 5 Diagnosis of Tumors Nuclear medicine helps in the detection and characterization of certain tumors. Examples include evaluation of nodular thyroid disease as nodules sair -gol blogling that may be malignant. gij - - - & & Scintimamography - is another example used in selected patients when mammography is not conclusive. It is a simple procedure using intravenous injection of 99mTc& MIBI followed by early and delayed imaging of the breasts and can indicate the nature of visualized lesions. - & - & & - & & Positron emission mammography (PEM) is recent being used in such cases. PEIM - 6 Diagnosis of Tumors Negative radionuclide mammography study & J 7 Diagnosis of Tumors Focal accumulation of 99mTc MIBI in the right breast (arrow) proven to be cancer MIBIS em 8 Diagnosis of Tumors # PET/CT is also increasingly used for managing many types of cancer including detection. Evaluation of the nature of solitary lung nodule and, in certain cases, of suspected breast cancer and tumor detection in cases with unknown primary are examples. 9 Diagnosis of Tumors Solitary lung nodule with high FDG activity (arrow) indicating malignancy 10 Diagnosis of Tumors 18F FDG-PET/CT study showing primary tumor in the right breast (solid arrow) and foci of lymph node involvement (open arrow) 11 Primry Staging of Malignant Disease neoplasm - 5;% +1 5. D Staging of cancer depends on the size of the primary neoplasm, its extent to regionalElymph nodes, and the presence or absence of metastasis. 3 C Accurate staging of malignant lesions at the time of initial presentation is of utmost importanceto provide appropriate management for a particular patient. & & - 12 Staging of Malignant Disease y ;& /my Overstaging can inappropriately deprive a patient from receiving curative treatment suchas surgery. 695 - S - On the other hand understaging can subject a patient to undergo futile but drastic treatments that can even increase the morbidity and mortality (e.g. pneumonectomy in lung cancer) without any increase in the chance of cure. - 99mTc MDP and Imaging plays a significant role in staging. Bone imaging using# 18F has established value in detecting metastatic bone disease. & -- Similarly, several radiopharmaceuticals are used in staging and follow-up of neuroendocrine tumors. Si , DP 13 Staging of Malignant Disease & Although the ideal staging is a microscopic process, many times it is not possible to biopsy each and every lesion to find out whether they are malignant or F not. best than CandURI FDGPET - - alone Nuclear medicine procedures, especially 18F FDG-PET, have a higher degree of accuracy compared with CT or MRI alone& in staging tumors particularly lymph z node involvement. = - -- = Da The accuracy of& CT in staging mediastinal disease in lung cancer as an example is approximately 70 %; MRI is slightly higher at around 80 %, while O 18F FDG accuracy is better than 90 %. # 70 % - &Lungeancer & - Pf - 1 - > > MRI· 80 % FDG - PET > 40 14 % C p 1) Ji,SS. Detection of Residual or Recurrent Disease The role of identifying viable tumors by imaging is increasing because of the problems encountered by MRI and CT, especially after surgical, radiation, or chemotherapy treatment in differentiating post-therapy changes from residual viable tumor tissue, local recurrence, or necrosis. As a result different radiopharmaceuticals play an important role in different malignancies and for detecting recurrence. PET, and if not thalium-201, helps in detecting tumor recurrence and in differentiating tumor recurrence f necrosis as post-therapy changes that is difficult to differentiate based on CT or MRI. 15 Detection of Residual or Recurrent Disease -D Baseline (a) and follow-up (b) F-18-FDG-PET/CT studies of a 21-year-old man with Hodgkin’s lymphoma. The follow-up shows recurrent tumor at multiple lymph nodes and spleen 16 Evaluating Response to Therapy The role of identifying viable tumors by imaging is increasing because of the problems encountered by MRI and CT, especially after surgical, radiation, or chemotherapy treatment in differentiating post-therapy changes from residual viable tumor tissue, local recurrence, or necrosis. As a result different radiopharmaceuticals play an important role in different malignancies and for detecting recurrence. PET, and if not thalium-201, helps in detecting tumor recurrence and in differentiating tumor recurrence f necrosis as post-therapy changes that is difficult to differentiate based on CT or MRI. 17 Evaluating Response to Therapy Patients can respond differently to the same treatment protocol and accordingly individualization of therapy should be more appropriate. -- %bis However, as tumors and their hosts are heterogeneous, there are many crucial secondary problems related to the individualization of therapy. The most important element in individualization of therapy is to be able to rapidly assess whether a chosen treatment strategy is effective. 18 Evaluating Response to Therapy Tumor volume changes observed during follow-up CT or MRI studies in principle can be used for evaluation of response to antitumor therapy. However, it is not early enough before the patient develops considerable toxicity and side effects from the prescribed chemotherapy. Radiopharmaceutical agents like Gallium in lymphoma and FDG in many tumors such as Lymphoma, lung, head and neck cancer, breast cancer and others have shown good results to predict response to a treatment regimen as early as after 1–3 cycles of chemotherapy. 19 Evaluating Response to Therapy Ga-67 study (a) in a patient with non-Hodgkin’s lymphoma. Follow-up study (b) shows clearly an excellent response to chemotherapy evidenced by clearing of the foci of uptake noted in the first pretherapy study 20 Radiotherapy Planning CT has a high diagnostic ability by visualizing lesion morphology and by providing the exact localization of sites but lacks the information of the functional status. Whereas, PET with 18F-FDG provides information about the metabolism and viability of the lesions but fails to provide precise topographic localization. 21 Radiotherapy Planning Fused images from FDG-PET and CT provides valuable information resulting in more accurate delineation of normal tissues from tumor-bearing areas at high risk for recurrence compared with CT alone and PET/CT can improve the therapeutic window for radiation therapy. Image fusion is also reported to increase the confidence of the radiation oncologist while drawing target volumes and thereby, reduces the interobserver variation. 22 Sentinel Lymph Node Localization This is relatively a recent trend for identifying the sentinel node(s) which represents the first drain for the primary tumor and is particularly used in breast Cancer, Cutaneous Melanoma, Squamous Cell Carcinoma of Head and Neck, and colorectal cancer. Tracer (99mTc radiolabeled particles) is injected into a specific location based on tumor type, and they are retained in the lymph nodes. Radioactive sentinel nodes can be detected using imaging with a gamma camera and/or a gamma probe at surgery. 23 Sentinel Lymph Node Localization a b (a) FDG-PET pretherapy study of a patient with non-Hodgkin’s lymphoma (b) Follow-up study showing favorable response to therapy with clearing of the uptake in multiple foci in the chest and abdomen 24 Sentinel Lymph Node Localization Initial (a) and follow-up (b) F-18 FDG of a patient with osteogenic sarcoma in the right distal femur (arrow) showing decreasing uptake in the follow-up study after chemotherapy indicating favourable response to therapy 25 Sentinel Lymph Node Localization Initial (a) and follow-up (b) F-18 FDG of a patient with osteogenic sarcoma in the right distal femur (arrow) showing decreasing uptake in the follow-up study after chemotherapy indicating favourable response to therapy 26 Sentinel Lymph Node Localization (a) Sentinel node visualization (arrow) in a patient with breast cancer. (b) an example of a gamma probe which is used during surgery for localization with no need for imaging 27 Sentinel Lymph Node Localization Although sentinel node imaging does not image the cancer, it can identify the lymph node/nodes where the primary tumor drains. Excision followed by immunohistological staining of the sentinel node can identify micrometastases. At the present time, sentinel lymph node localization technique is the most acceptable alternative to routine total node dissection, such as axillary dissection in breast cancer. 28 29 Further Reading 1. Bahl S, Alavi A, Basu S, Czerniecki BJ (2009) The role of PET and PET/CT in the surgical management of breast cancer: a review. PET Clin 4:277–287 2. Eubank WB, Lee JH, Mankoff DA (2009) Disease restaging and diagnosis of recurrent and metastatic disease following primary therapy with FDG-PET imaging. PET Clin 4:299–312 3. Francis IR, Brown RKJ, Avram AM (2005) The clinical role of CT/PET in oncology: an update. Cancer Imaging 5:S68–S75 4. Jana S, Abdel-Dayem HM (2006) Basis of tumor imaging; scintigraphic and pathophysiologic correlation. In: Elgazzar AH (ed) Pathophysiologic basis of nuclear medicine, 2nd edition. Springer, Berlin-New York 30