NFNF3612 - Anat Physio Lecture Note PDF
Document Details
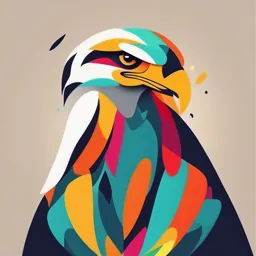
Uploaded by CleanerNewton
Tags
Summary
This document is a lecture note about the central nervous system, covering basic functions, the cerebrum, internal structure, and various areas of the cerebral cortex. It also discusses sensory areas, multimodal association areas, and neurotransmitters. Ideal for biological or medical students undertaking a course in human anatomy and physiology.
Full Transcript
The Central Nervous System Anatomy & Physiology Basic function of nervous system Sensory input : To gather information Integration: Process and interprets sensory input Motor output: Response by muscles or glands Ce...
The Central Nervous System Anatomy & Physiology Basic function of nervous system Sensory input : To gather information Integration: Process and interprets sensory input Motor output: Response by muscles or glands Cerebrum The cerebrum is composed of the right and left hemispheres, which are joined by the corpus callosum. The brain's surface, called the cerebral cortex, is folded into a series of gyri and sulci. These features increase the number of neurons (brain cells) that can fit within the skull. Sulcus - groove in cerebral cortex Gyrus - folded appearance Label the brain structures & sections Cerebrum The main sulci are: Central sulcus – groove separating the frontal and parietal lobes. Lateral sulcus – groove separating the frontal and parietal lobes from the temporal lobe. Lunate sulcus – groove located in the occipital cortex. The main gyri are: Precentral gyrus – ridge directly anterior to central sulcus, location of primary motor cortex. Postcentral gyrus – ridge directly posterior to central sulcus, location of primary somatosensory cortex. Superior temporal gyrus – ridge located inferior to lateral sulcus, responsible for the reception and processing of sound. Cerebrum: Internal structure The cerebrum is comprised of two different types of tissue – grey matter and white matter: Grey matter forms the surface of each cerebral hemisphere (known as the cerebral cortex) and is associated with processing and cognition. White matter forms the bulk of the deeper parts of the brain. It consists of glial cells and myelinated axons that connect the various grey matter areas. The cerebral cortex is classified into four lobes (frontal, parietal, temporal, occipital lobes. Each lobe contains various cortical association areas where information from different modalities are collated for processing. Together, these areas function to give us a meaningful perceptual interpretation and experience of our surrounding environment. Frontal lobe Parietal lobe Occipital lobe Temporal lobe Responsible for listening, as well as converting sounds into pictures. Provide general speech perception and enable communication. Allows you to fill in the words you hear, to find the necessary words to express your thoughts, to recognize tone, determine facial expression. Recognition of odor. If loss, early sign of Alzheimer’s. More info HERE Cerebral cortex has 3 functional areas: 1. Motor area 2. Sensory areas 3. Multimodal association area *All neurons in cerebral cortex are interneurons Motor areas Primary motor cortex Premotor cortex Broca’s area Reference link Control individual movements or The premotor cortex sends axons to the sequences of movements that require the primary motor cortex as well as to the activity of multiple muscle groups. spinal cord directly. It performs more complex, task-related processing than Encodes the force, direction, extent and primary motor cortex. speed of movement. More info on the functions of motor area HERE. Sensory areas Primary somatosensory cortex Somatosensory association cortex And others Detect sensory information from Integrate sensory inputs Visual the body regarding temperature, received from primary Auditory proprioception, touch, texture, somatosensory cortex to and pain. produce an understanding of the Vestibular object being felt. Olfactory Gustatory Multimodal association areas Sensory Multimodal Sensory Primary association association receptors sensory cortex cortex cortex Receive input from multiple senses and send output to multiple areas. Allows us to give meaning to the information received, store it in memory, tie it to previous experience and knowledge, and decide what action to take. Anterior association area Posterior association area Limbic association area In prefrontal cortex. Large region, include parts of Provides emotional impact that temporal, parietal and occipital makes scenes important to us. Most complex. lobe. Emotional behaviour, motivationally Involved in learning abilities, Plays a role in recognising patterns driven. intellect, memory, personalities. and faces, space. Develop slowly in children. Includes Wernicke’s area Anatomy of Language Language Speech Semantics or meaning The verbal means of communicating. Consists How to make new words of: Articulation: How speech sounds are made Grammar Social context Voice: The use of the vocal folds and breathing to produce sound (e.g., hoarseness, Language disorder: has trouble understanding breathiness, projection) other people or explaining thoughts, ideas and feelings. Fluency and prosody: The rhythm, intonation, stress. Speech disorder: cannot produce speech sounds correctly or fluently or has voice problems. Anatomy of Language Broca’s area Wernicke’s area located in the left hemisphere Located in the posterior superior associated with speech temporal lobe production and articulation. connects to Broca’s area via a ability to articulate ideas, use neural pathway. words accurately in spoken and primarily involved in the written language. comprehension, language Broca’s aphasia processing. Wernicke’s aphasia Diencephalon Thalamus Epithalamus Hypothalamus Gateway to cerebral cortex. Where pineal gland (synthesize Control pituitary gland, melatonin) is. releasing hormones. Afferent impulses converge and synapse in thalamic nuclei. To connect the limbic system Maintaining daily physiological Information is sorted and to other parts of the brain. cycles. edited before sent to areas in Controlling appetite. cerebral cortex. Managing sexual behavior. Mediate sensation, motor Regulating emotional activities, learning and responses. memory. Regulating body temperature. Brain region - Cerebellum A dense layer of grey matter surrounding an inner region of white matter. Function: Coordinates our sensations with responses from our muscles, enabling most of our voluntary movements. Also processes nerve impulses from the inner ear and coordinates them with muscle movement, thus helping us maintain balance and posture. Brain region - Brainstem Midbrain The midbrain is associated with a multitude of functions, including reward, vision, hearing, and movement. Contains serotonergic neurons. Home to substantia nigra and ventral tegmentel area (VTA), collection of dopaminergic neurons. Pons Latin word for “Bridge” Connects the rest of the brainstem to the cerebral cortex. Serves as a coordination centre for signals and communications that flow between the two brain hemispheres and the spinal cord. Contains the largest collection of noradrenergic neurons, raphe nuclei (cluster of cells that contain 5HT). Medulla oblongata The medulla helps regulate breathing, heart and blood vessel function Responsible for reflexive actions like coughing, sneezing, swallowing and vomiting. Crucial area for the control of both cardiovascular and respiratory functions Basal nuclei Basal nuclei (also known as basal ganglia): a group of nuclei within the white matter of each hemisphere that form a part of a basic feedback circuit. Receive information from several sources including the cerebral cortex then feeds this information back to the cortex, via the thalamus. Plays a role in muscle coordination and movement; modulate and refine cortical activity that influence muscle movement such as arm swinging during walking. Basal ganglia are functionally associated with subthalamic nuclei and substantia nigra. Disorders of basal nuclei includes Huntington's disease and Parkinson's disease. Ventricles The ventricular system is a set of communicating cavities within the brain that are filled with cerebrospinal fluid. Consists of two lateral ventricles, the third ventricle, and the fourth ventricle. Provide a pathway for the circulation of cerebrospinal fluid throughout the central nervous system. Other functional brain systems that span multiple brain structures 1. The limbic system 2. Reticular formations The Limbic System The Limbic System A collection of structures involved in processing emotion and memory, including the hippocampus, the amygdala, and the hypothalamus. These structures are known to be involved in emotional or affective processing, helps us respond to threats or express our emotions. The limbic system is highly connected to the endocrine and autonomic nervous system, becoming an important element in our body's response to stress. Explains why sometimes our emotions override cognitive function. Relates certain senses with memory i.e why certain smell is good/bad. Hippocampus Also known as a site where neurogenesis occurs. Where episodic memories are formed then filed away into long-term storage throughout other parts of the cerebral cortex. Always plays a role in spatial navigation. Associated with learning and emotions. Damage to this area can lead to severe memory impairments, and detrimental to spatial memory, for instance, remembering directions to locations that should be familiar to the individual. Amygdala Has a role in emotional responses, including feelings of happiness, fear, anger, and anxiety. This area is also key for the formation of new memories. The amygdala interacts with the hippocampus by attaching emotional content to memories. Fearful memories can be formed after only a few repetitions, which can result in avoidance of certain fearful stimuli. Therefore, the amygdala is also linked with the fight-or-flight response, as stimulating activity in the amygdala can influence the body’s automatic fear response. Damage to the amygdala can result in more aggression, irritability, loss of control of emotions, and deficits in recognising emotions, especially recognising fear. There are also links of amygdala differences in those with Autism, depression, posttraumatic stress disorder, and bipolar disorder. Basal ganglia Has multiple components (nucleus accumbens, ventral tegmental area, and ventral pallidum). These areas have shown to be involved in cognitive and emotional behaviors, and with having a role in rewards and reinforcements. Because of this, it can be linked with addictive behaviors and the formation of habits. Basal ganglia involvement in neuronal pathways connected with the prefrontal cortex may contribute to the pathogenesis of several disorders affecting mood regulation, such as depression, anxiety, OCD, and attention. A network of neurons extending from the spinal cord to the thalamus, with connections to the brainstem and diencephalon. Helps regulate level of arousal and consciousness. Can continuously send impulse to cerebral cortex, keeping the cortex alert, stimulated, and excited. Helps explain why some students are stimulated by busy environments, such as cafes, to study. Also filter repetitive or weak sensory signals eg. you are unaware of the watch you are wearing all day unless the strap is broken. During sleep, the center normally suppresses the individual's level of consciousness. The drug LSD can inhibit this and cause overloading of sensory stimulus. Protective structures of the CNS The brain is protected by Bone Meninges Cerebrospinal fluid Blood brain barrier. Meninges The meningeal layers (meninges) are three protective membranes the cover the brain and spinal cord. 1. The pia mater is the thin meningeal layer that directly covers the brain. 2. The arachnoid mater is the middle meningeal layer. The arachnoid mater contains projections that bridge the subarachnoid space between the arachnoid and pia mater. Blood vessels pass through the subarachnoid space. 3. The dura mater is the outermost meningeal layer. It is thicker and more fibrous than the other meningeal layers. Cerebrospinal Fluid (CSF) Clear, colorless plasma-like fluid that circulates through a system of cavities found within the brain and spinal cord. Produced by the choroid plexus and flows within space and canals of the CNS and eventually will be absorbed into the venous system by arachnoid granulations. Cerebrospinal fluid (CSF) Choroid plexus is located in the lining of the ventricles. It consists of capillaries and loose connective tissue, surrounded by cuboidal epithelial cells. Plasma is filtered from the blood by the epithelial cells to produce CSF. In this way, the exact chemical composition of the fluid can be controlled. Drainage of the CSF occurs in the subarachnoid space. Small projections of arachnoid mater (arachnoid villi/granulations) protrude into the dura mater. They allow the fluid to drain into the dural venous sinuses. Blood brain barrier Helps maintain stable environment in the brain from being exposed to chemical variations that might influence normal neuronal firing of neurotransmitters. An exceptionally impermeable tight junctions between capillary endothelial cells. Substances in the blood must pass through THREE layers before they reach the neurons. However, the barrier's permeability is selective, not absolute. Some substances can diffuse easily through the barrier and affect the brain. Blood brain barrier permeability Meninges cover the spinal cord Nerves leave at the level of each vertebrae Dorsal root Spinal cord Associated with the dorsal root ganglia – collections of cell bodies outside the central nervous system Ventral root Gray matter consists of sensory and motor nuclei. Dorsal horn - sensory Organisation Ventral horn - motor White matter consists of axons carrying information to and from the brain. of spinal cord Ascending - up to the higher centers (sensory inputs) Descending - down to the cord from the brain or within the cord to lower levels (motor outputs) Transverse - across from one side of the cord to the other. Neurons Components of synapse Axonal terminals contain vesicles with neurotransmitters Axonal terminals are separated from the next neuron by a gap Synaptic cleft – gap between adjacent neurons Synapse – junction between nerves Transmission at chemical synapses A single axon can have multiple branches, allowing it to make synapses on various postsynaptic cells. Similarly, a single neuron can receive thousands of synaptic inputs from many different presynaptic—sending—neurons. Inside the axon terminal of a sending cell are many synaptic vesicles. These are membrane-bound spheres filled with neurotransmitter molecules. When an action potential, or nerve impulse, arrives at the axon terminal, it activates voltage-gated calcium channels in the cell membrane. Ca2+ which is present at a much higher concentration outside the neuron than inside, rushes into the cell. The Ca2+ allows synaptic vesicles to fuse with the axon terminal membrane, releasing neurotransmitter into the synaptic cleft. The molecules of neurotransmitter diffuse across the synaptic cleft and bind to receptor proteins on the postsynaptic cell. Activation of postsynaptic receptors leads to the opening or closing of ion channels in the cell membrane. How a synapse work Excitatory and inhibitory postsynaptic potentials When a neurotransmitter binds to its receptor on a receiving cell, it causes ion channels to open or close. This can produce a localized change in the membrane potential—voltage across the membrane— of the receiving cell. In some cases, the change makes the target cell more likely to fire its own action potential. In this case, the shift in membrane potential is called an excitatory postsynaptic potential, or EPSP. In other cases, the change makes the target cell less likely to fire an action potential and is called an inhibitory post-synaptic potential, or IPSP. Signal termination What are the different ways to clear neurotransmitters from the synaptic cleft? Enzymatic degradation e.g. Ach Transported back into presynaptic to be recycled e.g. 5HT Supporting cells (glial cells) of the nervous tissue Other than neurons, there are several different cells that forms part of the central nervous system. These cell are: Astrocytes Microglia Ependymal cells Oligodendrocytes Neuron cell body Label and state the function(s) of each cell Myelin sheath type. Reference Neurotransmitters Neurotransmitters = "language" of the nervous system. Plays a role in emotion, hunger, satiation, memory, movement, and even smile. More than 50 have been identified. Some neurons produce and release one type of neurotransmitters. Most produce two or more, and may release one or all at a given time. Can be classified by chemical structure or by function. Norepinephrine/Noradrenaline Generally excitatory behavioral effects Biosynthesis: DA NE Dopamine Beta-hydroxylase Many receptor types (metabotropic) 1, 1-2 (postsynaptic, excitatory) 2 (autoreceptor, inhibitory) Major pathway Locus coerulus → broad projections Major NE Pathway Locus Coeruleus → throughout brain [vigilance and attentiveness] Dopamine Monoamine and catecholamine neurotransmitter - derived from an amino acid & contains catechol nucleus. Dopamine synthesis: Tyrosine converts to L-Dopa and is decarboxylated into Dopamine. Dopamine rich areas of the brain: Substantia Nigra, Ventral regimental area (Both located in the mid brain), Hypothalamus, Olfactory bulb, Retina Dopamine pathways: 1. Nigrostriatal Pathway - from the substantia nigra to the striatum 2. Mesolimbic Pathway - from ventral tegmental area to the nucleus accumbens 3. Mesocorticol Pathway - from ventral tegmental area throughout the cerebral cortex Dopamine Receptors: 5 subtypes, GPCR Dopamine metabolism: Dopamine transporter (reuptake), COMT & MAO-B (enzyme) Serotonin (5HT) Monoamine neurotransmitter - derived from an amino acid Dopamine synthesis: Tryptophan converts to 5htp and is decarboxylated into 5HT. Serotonin rich areas of the brain: Raphe nuclei in the brainstem Serotonin pathways: Raphe nuclei to the rest of the brain Serotonin Receptors: 7 families, 13 subtypes, GPCR + ion channel Serotonin metabolism: Serotonin transporter (reuptake), COMT & MAO-B (enzyme) GABA (Gamma Aminobutyric Acid) Principal Inhibitory NT Biosynthesis: Glu Glutamic Acid GABA Decarboxylase (GAD) and B6 Removed by reuptake 2 receptor types GABAA GABAC (ionotropic; Cl- channel) GABAB (metabotropic; K+ channel) GABAA Binding Sites GABA Benzodiazepine (indirect agonist) – Probably also site for alcohol – Endogenous inverse agonist binds here Barbiturate (indirect agonist) Steroid (indirect agonist) Picrotoxin (inverse agonist) Phosphate groups attach to the receptor inside the cell and regulate receptor sensitivity (via phosphorylation) to agents such as alcohol Acetylcholine Quaternary amine Mostly excitatory effects Synthesis Removal Acetyl CoA CoA Acetate + + Ach + Choline Acetyltransferase Acetylcholine Choline (ChAT) ACh Esterase (AChE) Choline 2 receptor types Nicotinic (ionotropic) Muscarinic (metabotropic) Glutamate Principal excitatory NT Biosynthesized as byproduct of cell metabolism Removed by reuptake Elevated levels → neurotoxic 4 receptor types – NMDA – AMPA Ionotropic – Kainate – mGluR - Metabotropic NMDA Binding Sites “The specific subunit composition of each receptor determines its 4 outside cell overall pharmacological properties” – Glutamate – Glycine Obligatory co-agonist Inhibitory NT at its “own” receptor – Zinc (inverse agonist) – Polyamine (indirect agonist) 2 inside cell – Magnesium (inverse agonist) – PCP (inverse agonist) Neuropeptides Low concentration in brain (picomolar) Large vesicles Co-localized with other transmitters Widespread but particular in pain modulation, rewards, response to stress and autonomic control Mostly inhibitory Virtually all opioid receptors are metabotropic Slow acting, long duration (10-1000 ms) Examples: Enkephalins, Endorphins, Oxytocin, Vasopressin, Opioids Endogenous Opioids -endorphins = the heroine within – made from proopiomelanocortin (POMC) – produced in pituitary gland, hypothalamus, brain stem Enkephalins – made from preproenkephalin (PENK) – produced throughout brain and spinal cord Dynorphins – made from preprodynorphin (PDYN) – produced throughout brain and spinal cord Opioids Receptors Receptor High affinity ligands mu -endorphin, enkephalins delta enkephalins kappa dynorphins Opioids act at all opioid receptors (inhibitory GPCRs), but with different affinities Distributed throughout brain and spinal cord, especially in limbic areas Some overlap but quite distinct localizations Opioid Receptors (cont.) Metabotropic, with either – moderately fast indirect action on ion channels – long-term action via changes in gene expression Most analgesic effects from mu receptor action Some analgesic effects from delta Many negative side effects from kappa Opioids Morphine and heroin are agonists that bind to receptor sites, thereby increasing endorphin activity Functional classification Some neurotransmitters are generally viewed as “excitatory," making a target neuron more likely to fire an action potential. Others are generally seen as “inhibitory," making a target neuron less likely to fire an action potential. For instance: Glutamate is the main excitatory transmitter in the central nervous system. GABA is the main inhibitory neurotransmitter in the adult vertebrate brain. Glycine is the main inhibitory neurotransmitter in the spinal cord. HOWEVER, a neurotransmitter can sometimes have either an excitatory or an inhibitory effect, depending on the context. How can that be the case? As it turns out, there isn’t just one type of receptor for each neurotransmitter. Instead, a given neurotransmitter can usually bind to and activate multiple different receptor proteins. Whether the effect of a certain neurotransmitter is excitatory or inhibitory at a given synapse depends on which of its receptor(s) are present on the postsynaptic (target) cell. Example: Acetylcholine Acetylcholine is excitatory at the neuromuscular junction in skeletal muscle, causing the muscle to contract. In contrast, it is inhibitory in the heart, where it slows heart rate. These opposite effects are possible because two different types of acetylcholine receptor proteins are found in the two locations. The acetylcholine receptors in skeletal muscle cells are called nicotinic acetylcholine receptors. They are ion channels that open in response to acetylcholine binding, causing depolarization of the target cell. The acetylcholine receptors in heart muscle cells are called muscarinic acetylcholine receptors. They are not ion channels, but trigger signaling pathways in the target cell that inhibit firing of an action potential. Types of neurotransmitter receptors Can divide the receptor proteins that are activated by neurotransmitters into two broad classes: Ionotropic receptors (Ligand-activated ion channels): These receptors are membrane-spanning ion channel proteins that open directly in response to ligand binding. Metabotropic receptors: These receptors are not themselves ion channels. Neurotransmitter binding triggers a signaling pathway, which may indirectly open or close channels (or have some other effect entirely). Ligand-activated ion channels Undergo a change in shape when neurotransmitter binds, causing the channel to open. This may have either an excitatory or an inhibitory effect, depending on the ions that can pass through the channel and their concentrations inside and outside the cell. Ligand-activated ion channels are large protein complexes. They have certain regions that are binding sites for the neurotransmitter, as well as membrane-spanning segments that make up the channel. Produce very quick physiological responses. Metabotropic receptors Activation of the second class of neurotransmitter receptors only affects ion channel opening and closing indirectly. Signaling through these metabotropic receptors depends on the activation of several molecules inside the cell and often involves a second messenger pathway. Because it involves more steps, signaling through metabotropic receptors is much slower than signaling through ligand-activated ion channels. Some metabotropic receptors have excitatory effects when they're activated while others have inhibitory effects. Often, these effects occur because the metabotropic receptor triggers a signaling pathway that opens or closes an ion channel. Neurotransmitters Ionotropic receptors Metabotropic receptors a1, a2, b1, b2, b3 adrenergic Norepinephrine (NE) - Dopamine (DA) Serotonin (5HT) GABA Glutamate Glycine Endorphins Define the following & give example(s): Heteroreceptors Autoreceptors The Reward System Refers to a group of structures that are activated whenever we experience something rewarding like using an addictive drug. Major components & their function: Ventral tegmental area (VTA) Nucleus accumbens Amygdala Hippocampus Prefrontal cortex