Neuropsychology 2 Unit PDF
Document Details
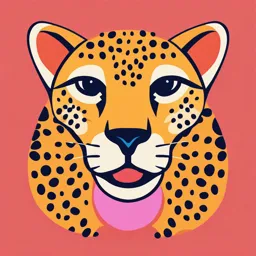
Uploaded by AmbitiousMookaite
neha bhansali
Tags
Summary
This document contains notes on Module 2: Cortical Organization and Functions. It covers the anatomy and functions of various sensory systems (vision, hearing, etc.) and the neocortex. It provides an overview of the processes and pathways.
Full Transcript
Module 2 Cortical Organization and Functions -neha bhansali 2.1 Organization of the Sensory Systems -Anatomy and functions of sensory systems (e.g., vision, audition, somatosensation) Neural processing and perception of sensory information Disorders and dysfunctions related to sens...
Module 2 Cortical Organization and Functions -neha bhansali 2.1 Organization of the Sensory Systems -Anatomy and functions of sensory systems (e.g., vision, audition, somatosensation) Neural processing and perception of sensory information Disorders and dysfunctions related to sensory systems 2.2 Overview of the neocortex and its role in higher cognitive functions -Principles of Neocortical Function, Neural mechanisms underlying perception and attention 2.3 Hemispheric specialization and lateralization of brain functions Differences between the left and right hemispheres Role of cerebral asymmetry in language, emotion, and cognition Variations in Cerebral Asymmetry 2.4 Individual differences in cerebral asymmetry Effects of handedness, sex, and genetics on cerebral lateralization Clinical implications and disorders associated with atypical cerebral asymmetry 2.1 Sensations - physical changes in our sensory systems in response to stimulation; perception is our interpretation of those sensations. Perception has us believe that we see, hear, touch, smell, and taste real things in a real world. In fact, the only input our brains receive from the “real” world is a series of action potentials passed along the neurons of our various sensory Pathways. do not fully understand how nerve impulses are converted into meaningful perceptions. Sensory Systems Vision Hearing Body senses- Somatosensory, Vestibular and Proprioception Chemical Senses- Taste and Smell Vision Photoreceptors 1. the cells are transparent, and the photoreceptors are extremely sensitive: they can be excited by the absorption of a single photon 2. Second -many of the fibers forming the optic nerve bend away from the retina’s central part, or fovea, so as not to interfere with the passage of light through the retina. Because of this bending, the fovea is a depression on the retinal surface (fovea means “pit” in Latin), Rods Capable of scotopic vision (low light levels), they have a low spatial acuity and do not mediate color Populated at the periphery of the retina. They are rod-shaped, facilitating vision during dim light/night Contain rhodopsin pigment rich in vitamin A responsible for the night vision hence making rods sensitive to light Cones Capable of photopic vision(higher light levels), they are responsible for high spatial acuity and responsible for color vision They are populated in the central fovea region and mostly found in retina Help distinguish color and other fine details and are cone-shaped They are of three types – long-wavelength sensitive cones(L-cones), middle-wavelength sensitive cones (M-cones) and short-wavelength sensitive cones (S-cones) Fovea - Cones most dense in FOVEA ( central part of retina) - this allows details in vision - Rods not present in fovea The photoreceptive cells diagrammed in Figure 8.9A synapse on bipolar cells in which they induce graded potentials. Bipolar cells in turn induce action potentials in retinal ganglion cells (RGCs). RGC axons collect in a bundle at the optic disc, your blind spot, and leave the eye to form the optic nerve in the brain proper. (Remember that the retina is a part of the brain.) Other retinal cells — including horizontal and amacrine cells — contribute to the retina’s processing of visual information. Visual Pathways the two optic nerves (one from each eye) meet and form the optic chiasm (from the Greek letter chi, which looks like an X). Contralateral control Pathways -1. Geniculostriate Pathway-from the retina to the lateral geniculate nucleus (LGN) of the thalamus to the primary visual cortex (V1, Brodmann’s area 17), is the geniculostriate pathway. -takes part in pattern, color, and motion recognition and includes conscious visual functions (Livingston & Hubel, 1988). 2. Tectopulvinar Pathway- The second main visual pathway takes part in detecting and orienting to visual stimulation. - ( optic tract to thalamus to midbrain structures)- older pathways. For detecting space and movement tectopulvinar pathway relays from the eye to the superior colliculus in the midbrain tectum and reaches the visual areas in the temporal and parietal Lobes through relays in the lateral posterior-pulvinar complex of the Thalamus and ends in V2 region (also called as prestriate cortex).. The pathway to the colliculus constitutes the entire visual system in fish, amphibians, and reptiles and thus is capable of sophisticated vision in locating events in space. In mammals, the additional projection from the colliculus to the cortex via the thalamic pulvinar nucleus provides information to the cortex about the absolute spatial location of objects (independent of a viewer’s visual gaze). Symptoms of damage to the geniculostriate and tectopulvinar system include impairments in pattern, color, and motion perception, as well as visual-form agnosia (Greek for “not knowing”), the inability to see the shapes of objects or to recognize them, and visual ataxia (Greek for “not orienting”; also called optic ataxia), the inability to recognize where objects are located and to reach for them Hearing Hearing, or audition, the ability to construct perceptual representations from pressure waves in the air, includes sound localization — identifying the source of air-pressure waves — and echo localization — identifying and locating objects by bouncing sound waves off them — as well as the ability to detect the complexity of pressure waves Changes in air pressure cause sound waves. The frequency, amplitude, and complexity of these changes determine what we hear. We hear the frequency,or speed, of pressure changes as changes in pitch; we hear the amplitude, or intensity, of pressure changes as loudness; and we hear the complexity of pressure changes as timbre, the perceived uniqueness or tonal quality of a sound The outer ear consists of the pinna, the external structure that catches waves of air pressure and directs them into the external ear canal, which amplifies them somewhat and directs them to the eardrum at its inner end. Sound waves striking the eardrum vibrate it at frequencies that vary with the waves’ frequencies. Sound waves striking the eardrum vibrate it at frequencies that vary with the waves’ frequencies. On the inner side of the eardrum is the middle ear, an air-filled chamber that contains the three smallest bones in the human body, connected in a series. These three ossicles — the hammer, anvil, and stirrup, so-called because of their distinctive shapes — attach the eardrum to the oval window of the inner ear. The inner ear consists of the cochlea, which contains the organ of Corti and the auditory sensory receptors called hair cells. -snail shell The sounds are caught in the outer ear and amplified by the middle ear. In the inner ear, they are converted to action potentials on the auditory pathway going to the brain, and we interpret the action potentials as our perception of sound. In short, pressure waves in the air are amplified and transformed a number of times in the ear: by deflection in the pinna, by oscillation as they travel through the external ear canal, and by the movement of the bones of the middle ear to the cochlea. The place representation, in which different points on the basilar membrane represent different sound frequencies, is also present in the auditory cortex: there, too, different locations represent different sound frequencies. Thus, the primary auditory cortex is a map of sound frequency, a tonotopic representation (literally, “tone map”). The inferior colliculus, the main auditory region in fish, amphibians, and reptiles, plays a role in orienting to sound location. The inferior and superior colliculi are interconnected (see Figure 3.19), and the superior colliculus functions to orient the head toward visual stimuli. The connections between these two areas thus allow conjoint recognition not only of the direction a sound comes from, but also the visual source of the sound. Primary auditory pathway 2 distinct pathways emerge from the colliculus and course to the ventral and the dorsal medial geniculate nuclei in the thalamus. 1.The ventral region projects to the primary auditory cortex (A1 or Brodmann’s area 41)- identifies the sound “what” 2. The dorsal region projects to the secondary auditory regions, thus adhering to the sensory systems’ general pattern of having multiple independent ascending pathways to the cortex- indicates its spatial Source.”where” Both ipsilateral and contralateral projections Body Senses Somatosensory Receptors Nociception -Slow Hapsis and Proprioception -Rapid Nociception (noxious perception) is the perception of pain, temperature, an itch. When damaged or irritated, these endings secrete chemicals, usually peptides, that stimulate the nerve, producing action potentials that then convey messages about pain, temperature, or itch to the CNS. Many internal organs, including the heart and kidneys and the blood vessels, have pain receptors, but the ganglion neurons carrying information from these receptors lack pathways to the brain. The neurons in the spinal cord that relay pain, temperature,and itch messages to the brain receive two sets of signals: one from the body’s surface and the other from the internal organs. These spinal-cord neurons cannot distinguish between the two sets of signals — nor can we. As a result, pain in body organs is often felt as referred pain coming from the body surface. Referred pain- coming from surface Hapsis From the Greek for “touch,” hapsis is our tactile perception of objects. Haptic receptors enable fine touch and pressure, allowing us to identify] objects we touch and grasp. Haptic receptors occupy both superficial and deep skin layers and are attached to body hairs as well. John Rothwell and his colleagues (1982) described a patient, G.O., who was deafferentated (lost afferent sensory fibers) by a disease that destroyed touch sensation in his hands. He could not feel when his hand was holding something. G.O. began movements quite normally, but as he proceeded, the movement patterns gradually fell apart. When he tried to carry a suitcase, for example, he would quickly drop it unless he continually looked down toconfirm that he was carrying it. Proprioception is the perception of body location and movement. Proprioceptors, are encapsulated nerve endings sensitive to the stretch of muscles and tendons and to joint movement. We are ordinarily unaware of how proprioception contributes to our movements, but the loss of this sense highlights its importance, Somatosensory Pathways Two major somatosensory pathways extend from the spinal cord to the brain: a. posterior spinothalamic tract for hapsis (pressure) and proprioception (body awareness) - Rapid adaptation + myelinated -heir cell bodies are located in the posterior-root ganglia, their dendrites project to sensory receptors in the body, and their axons project into the spinal cord (detailed in Figure 3.13B). b. anterior spinothalamic tract for nociception (irritation)- slow adaptation + unmyelinated They follow the same course to enter the spinal cord but, once there, project to relay neurons in the more central region of the spinal cord, the substantia gelatinosa. The second-relay cells then send their axons across to the other side of the cord, where they form the anterior spinothalamic tract A unilateral spinal cord injury that cuts the somatosensory pathways in that half of the spinal cord results in the bilateral symptoms known as Brown– Séquard syndrome, after the two investigators who first described it. loss of hapsis and proprioception occurs unilaterally on the side of the body where damage occurred, loss of nociception occurs contralaterally, on the side of the body opposite the injury. Unilateral damage to one of the points where the pathways come together — that is, the posterior roots, brainstem, and thalamus — affects hapsis, proprioception, and nociception equally because these parts of the pathways are in proximity Somatosensory Cortex The density of somatosensory receptors varies greatly from one place to another on the body surface (and varies from species to species), and somatotopic maps manifest this variability. Thus, in the human cortex , the areas representing the hands and tongue, which have a high receptor density, are extremely large, whereas the areas representing the trunk and legs, which have a low receptor density, are small. Vestibular System - Motion and Balance interoceptive receptors in the inner-ear vestibular system informed your visual system that your head was moving. The visual system responded by compensating for the head movements, and you observed the hand as stationary. Like other somatosensory submodalities, the vestibular system helps you distinguish between your own behavior and the actions of others. The inner ear contains the organs that allow you to perceive your own motion and to stand upright without losing your balance Named for an entranceway (vestibule), the vestibular organs contain hair cells that bend when the body moves forward or when the head changes position relative to the body. The semicircular canals are filled with a fluid called endolymph. Immersed in the endolymph is a set of hair cells. When the head moves, the endolymph also moves, pushing against the hair cells and bending the cilia at their tips. The receptor potential activates action potentials in neurons in the vestibular nerve, which carries information about head position to the brain. Fibers from the balance receptors project over the eighth cranial nerve to a number of nuclei in the brainstem. These nuclei interact in the hindbrain to help keep us balanced while we move; they also aid in controlling eye movements at the midbrain level Ultimately, through connections in the cerebellum, information from the vestibular system allows us not only to balance but also to record and replay, actively and in the mind’s eye, the movements we have made. Vertigo (from the Latin for “spinning”), a sensation of dizziness when one is not moving, is a dysfunction of the inner ear and can be accompanied by nausea and difficulty maintaining balance while walking. Ménière disease, named after a French physician, is a disorder of the middle ear that results in vertigo and loss of balance. It may affect as many as 200 people in 100,000 — women more frequently than men — and is most likely to occur in middle age GUSTATION AND OLFACTION Unlike carnivores and rodents, primates have relatively small gustatory and olfactory systems in comparison with their well-developed visual systems. For taste, the receptors are the taste buds, which most people mistakenly believe to be the bumps on the tongue. In fact, the bumps, called papillae, are probably there to help the tongue grasp food; the taste buds lie buried around them Chemicals in food dissolve in the saliva that coats the tongue and disperse through the saliva to reach the taste receptors. If the tongue is dry, the taste buds receive few chemical signals, and food is difficult to taste. Taste receptors are also found in the gut and elsewhere in the body, where they may play a role in food absorption, metabolism, and Appetite. Tastes are related to ingestive behavior as well as to food choice. Animals sensitive to sweet tastes respond with tongue licks and mouth movements designed to take in food when a sweet substance is placed in their mouth. Humans have clear individual differences in taste thresholds. Older people generally have higher thresholds, largely because the number of taste buds is dramatically reduced as we age. Children tolerate spicy foods poorly because their sense of taste is stronger. Gustatory pathways Three cranial nerves carry information from the tongue: the glossopharyngeal nerve (9), the vagus nerve (10), and the chorda tympani branch of the facial nerve (7). All three of these nerves enter the solitary tract, which is the main gustatory pathway one to S1 and the other to a region just rostral to S2 in the insular cortex. The latter region is probably dedicated entirely to taste because it is not responsive to tactile stimulation. Insular Cortex- Lateral Sulcus the S1 projection is sensitive to tactile stimuli and is probably responsible for localizing tastes on the tongue. (Those who enjoy wine are familiar with this distinction because wines are described not only by their gustatory qualities but also by the way they taste on different parts of the tongue.) These areas project in turn to the OFC, in a region near the input of the olfactory cortex, which may be the secondary taste area. The other route from the solitary tract (shown in blue in Figure 8.28) leads to the pontine taste area, which in turn projects to the lateral hypothalamus and amygdala. Both areas have roles in feeding, although the gustatory input’s precise contribution to this behavior is uncertain. Smell The receptor surface for olfaction is the olfactory epithelium, located in the nasal cavity and composed of three cell types: receptor hair cells, supporting cells, and an underlying layer of basal cells (Figure 8.27). The mitral-cell projection reaches the pyriform cortex and from there reaches the hypothalamus, the amygdala, the entorhinal cortex of the temporal lobe, and the orbitofrontal cortex (OFC), the area of prefrontal cortex located behind the eye sockets (the orbits). Odors must pass through the mucus to reach the receptors, which means that changes in the mucus layer’s properties (such as occur when a person has a cold) may influence how easily someone can detect an odor. The area of the olfactory epithelium varies across species. In humans, it is estimated to range from 2 square centimeters to 4 square centimeters; in dogs, the area is about 18 square centimeters; and in cats, it is about 21 square centimeters. Such differences support the observation that some species are more sensitive to odors than others. Olfactory The major output of the bulb is the lateral olfactory tract, which passes ipsilaterally to the pyriform cortex, the amygdala, and the entorhinal cortex (Figure 8.29). The pyriform cortex’s primary projection goes to the central part of the dorsomedial nucleus in the thalamus. The dorsomedial nucleus in turn projects to the orbitofrontal cortex, which can be considered the primary olfactory neocortex some responsive to specific odors and others broadly tuned (Nara et al., 2011). This research also suggests that neurons responsive to specific odors are linked to specific olfactory-related behaviors, but they elicit these behaviors within a context identified by the more broadly tuned neurons. Accessory Olfactory System A unique class of odorants is pheromones, biochemicals released by one animal that act as chemosignals (chemical signals) to affect the physiology or behavior of another animal. In many animals, pheromones are unique odors and are detected by a special olfactory receptor system known as the vomeronasal organ, which is made up of a small group of sensory receptors connected by a duct to the nasal passage. The receptor cells in the vomeronasal organ send their axons to the accessory olfactory bulb, which lies adjacent to the main olfactory bulb. The vomeronasal organ connects primarily with the amygdala and hypothalamus and likely plays a role in reproductive and social behavior Most evidence suggests that humans do not have a vomeronasal organ, so odors that affect human sex-related behaviors must act through conventional olfactory neural pathways. Humans make extensive use of odor to guide their behavior in such things as food choice, mate choice, and the avoidance of various substances. Much of this behavior is influenced by learning. There has been substantial research into whether humans use pheromones in social communication, as do some other animals, for detecting and choosing mates. However, results have been inconclusive; many studies have produced positive findings, but others have produced negative ones. Our sensory impressions are affected by the contexts in which they take place, by our emotional states, and by our experiences. All of these factors contribute to perception, the subjective experience by the brain of the sensory transduction events outlined in this chapter. Evidence that perception is more than sensation lies in the fact that we transform the same sensory stimulation into totally different perceptions at different times. Perception If you recall shivering on hearing a particular piece of music or the noise of fingernails scratching across a chalkboard, you have felt sound. Such sensory mixing, or synesthesia (literally, “feeling together”), is the ability to perceive a stimulus of one sense as the sensation of a different sense. For For some people, the mixing of the senses is much richer; these people are referred to as synesthetes In addition, more than 1 in 5 people described as autistic experience similar synesthesia (Burghoorn et al., 2020). Synesthesia can become more pronounced following brain injury, suggesting that new connections between sensory systems occur as the brain compensates for injury (Yong, 2017). Synesthetes are grouped into two general classes. Projectors experience sensory mixing as reality. When looking at numbers printed in black text, for example, 7 appears yellow to a projector, whereas 2 looks blue Associators experience sensory mixing in their mind’s eye, associating one number with one color and another number with another color but not actually seeing those colors These actions increase the activity in fine-touch and pressure pathways and can block information transmission in spinal-cord relays through connections known as pain gates. A dramatic example of sensory interaction between vision and hearing is the visual modification of sound known as the McGurk effect. If a recorded speech syllable, such as “ba,” is played to a listener who at the same time observes someone whose lips are articulating the syllable “da,” the listener hears not the actual sound ba but the articulated sound da. The viewed lip movements modify the listener’s auditory perception. 2.2 Overview of the neocortex and its role in higher cognitive functions Hierarchical Organization from Spinal Cord to Cortex Brain Plasticity Levels of function - “ Subcortical structures can mediate complex behaviors” “ hierarchical organization. English neurologist John Hughlings-Jackson (1835–1911) proposed this principle of cerebral organization in which information is processed serially and organized as a functional hierarchy (1931). Each successively higher level controls more complex aspects of behavior and does so via the lower levels Spinal Cord - Reflexes Injury -paraplegia and Quadriplegia- “The spinal cord also contains the neural circuitry to produce stepping responses and walking, provided that body weight is supported.” Hindbrain - “If the hindbrain and spinal cord remain connected after an injury but both are disconnected from the rest of the brain, the subject is called a low decerebrate.” LOW decerebrate animal has difficulty maintaining consciousness because many essential inputs to the brain regions above the injury are disconnected, presumably leaving the forebrain “in the dark.” The Midbrain: Spontaneous Movement- “The next level in the brain organization hierarchy can be seen in an animal with an intact midbrain (mesencephalon) but lacking higher-center functioning an intact midbrain (mesencephalon) but lacking higher-center functioning. High decerebration tectum, the coordinating centers for vision (superior colliculus) and hearing (inferior colliculus) and, in the tegmentum, a number of motor nuclei subsets of voluntary movements — movements that take an animal from one place to another, such as turning, walking, climbing, swimming, and flying — are present at the subcortical level of the midbrain.” High-decerebrate animals can also effectively perform automatic movements, units of stereotyped behavior linked in a sequence. Grooming, chewing food, lapping water, and rejecting food are representative automatic behaviors of the rat.” The Diencephalon: Affect and Motivation “A diencephalic animal, although lacking the basal ganglia and cerebral hemispheres, has an intact olfactory system, enabling it to smell odors at a distance. ” -hypothalamus and pituitary also intact, and their control over hormonal systems and homeostasis no doubt integrates the body’s physiology with the brain’s activity. ” “Walter Cannon and S. W. Britton (1924) studied diencephalic cats and described what they called “quasi-emotional phenomena,” or sham rage, such as that usually seen in an infuriated animal. ” Clinical reports indicate that similar sham emotional attacks can occur in people who have hypothalamic lesions Diencephalic animal’s hyperactivity - sham motivation to distinguish it from a typical animal’s goal-oriented behavior. ” “The Basal Ganglia: Self-Maintenance “Decortication, removal of the neocortex, leaves the basal ganglia and brainstem intact” At first glance appear normal- basic functions , walking, eating, finding food, mating all seem fine Inhibition and facilitation of movements The Cortex: Intention Goal oriented behavior Decorticate animals can perform pattern discriminations in different sensory modalities - only very simple sensory stimuli Decorticate animals “David Oakley (1979) show that decorticate animals can perform well in tests of classical conditioning, operant conditioning, approach learning, cue learning, and pattern discrimination Cannot perform - complex pattern discriminations and how to find their way around in a space. Skilled movements, sequencing of activities - impaired Neocortex The neocortex, also called the neopallium, isocortex, or the six-layered cortex, is a set of layers of the mammalian cerebral cortex involved in higher-order brain functions such as sensory perception, cognition, generation of motor commands, spatial reasoning and language. In the human brain, the cerebral cortex consists of the larger neocortex and the smaller allocortex. The neocortex is made up of six layers, labelled from the outermost inwards, I to VI. Cortex structure “ Paul Flechsig (1920) divided cortical regions into (1) an early-myelinating primordial zone including the motor cortex and a region of visual, auditory, and somatosensory cortex; (2) a secondary field bordering the primordial zone that myelinates next; and (3) a late-myelinating (tertiary) zone that he called “association.” Jerison - evolutionary as cortex grew amount of sensory information reaching cortex also grew- as a result categorization and labeling became important to distinguish and keep in memory Labels, coordinates, and categories are products of cognition. Viewed in this way, Jerison’s (1991) analogy of multiple cortical maps provides a basis for thinking about how the information that is arriving at the cortex is integrated into perception and organized as knowledge, thought, and memory. A Hierarchical Model of Cortical Function Alexenader Luria - Functional Model 1960s 1.The posterior cortex (parietal, occipital, and temporal lobes) is the sensory unit that receives sensations, processes them, and stores them as information 2. The anterior cortex (frontal lobe) is the motor unit that formulates intentions, organizes them into programs of action, and executes the programs The first zone corresponds to Flechsig’s primary cortex; the second zone corresponds to the more slowly developing cortex bordering the primary cortex, labeled secondary cortex by Luria; and the third is the most slowly developing cortex, which Luria labeled tertiary cortex. Sensory input enters the primary sensory zones, is elaborated in he secondary zones, and is integrated in the tertiary zones of the posterior unit. Luria (1973) based his theory on three assumptions: 1. The brain processes information serially, one step at a time. Thus, information from sensory receptors goes to the thalamus, then to the primary cortex, then to the secondary cortex, and finally to the tertiary sensory cortex. Similarly, output goes from tertiary sensory to tertiary motor, then to secondary motor, and finally to primary motor. 2. Serial processing is hierarchical: each level adds complexity that is qualitatively different from the processing in the preceding levels. The tertiary cortex could be considered a terminal station insofar as it receives input from the sensorimotor and perceptual areas and performs higher cognitive processes on that input. 3. Our perceptions of the world are unified and coherent. Luria’s formulation was in accord with the commonsense view that some active process produces each percept, and, naturally, the simplest way to do so is to form it in the tertiary cortex. Consider a simplified example of Luria’s model: you are walking along and come upon a soccer game. 1.The actual perception of the movements of players and the ball is in the primary visual area. 2.The secondary visual sensory zone recognizes that those activities constitute a soccer game. 3.In the tertiary zone, the sounds and movements of the game are synthesized into the realization that one team has scored and is ahead and that the game has significance for league standings. By the time the information is integrated in the tertiary sensory zone, it is considerably richer than what we would think of as “sensory.” Rather, it has become knowledge. Information in the tertiary sensory zone activates the paralimbic cortex for memory processing and the amygdala for emotional assessment. These cortical events can then activate, in the tertiary zone of the frontal (motor) cortex, the intention to find a viewing spot and root for your team The execution of this plan is formulated in the secondary frontal zones. The actual movements required to join the crowd are initiated in the primary motor zone of the frontal cortex. Damage to the paralimbic cortex leaves no memory of the event, and damage to the amygdala renders the person unresponsive to the event’s emotional significance. A lesion in the tertiary motor area might prevent formation of the intention to become a soccer player and join a club, buy a uniform, or get to practice on time. A lesion in the secondary motor zone might make it difficult to execute the sequences of movements required in play A lesion in the primary zone might make it difficult to execute a discrete movement required in the game — for example, kicking the ball. The beauty of Luria’s theory is that it used the then-known anatomical organization of the cortex to craft a simple explanation for observations that Luria made daily in his clinic and published in 1973. The difficulty is that its basic assumptions have been questioned by newer anatomical and physiological findings The fact that cortical operations are relayed directly to subcortical areas implies that cortical processing can bypass Luria’s motor hierarchy and go directly to subcortical motor structures An area such as the primary visual cortex, which processes color, form, and movement, might be considered more complex than an area that processes only color. Contemporary model The Felleman and Van Essen model and the process of reentry illustrate that cortical connectivity is not a simple junction of one cortical module with another but rather a dynamic interplay between and among the operations of different regions. By analyzing the patterns of connectivity among the visual, auditory, and somatosensory areas, Felleman and van Essen found evidence of what they called a distributed hierarchical system Attention 1. Ventral Attention Network (TPJ , and ventral frontal cortex- inferior and middle - largely laterized on rt hemisphere) 2. Dorsal Attention Network ( Frontal parietal functioning- goal directed attention- includes eye fields and acc) 3. Default mode network- ( medial and posterior regions of the frontal and parietal lobes) Ventral attention network TPJ , and ventral frontal cortex- inferior and middle More lateralized on rt hem Reflexive attn Attn to novel stimuli, location change (where ) Warning stimuli Lesions- Neglect- disengaging function Dorsal Attention Network Frontal parietal functionin network reflects the sources of attention signals in the goal-directed control of attention. Default mode network -became clear that some connected areas of the brain are highly active when a person is not engaged in focused activities but rather appears to be resting in a passive condition. This network, which includes parts of the prefrontal cortex, posterior parietal cortex, posterior cingulate cortex, and medial temporal regions, came to be known as the default mode network, or default network (see Figure 10.19A). Because the default network was originally identified as a resting state, it was thought to reflect a mind-wandering state, such as daydreaming, but this is a misnomer. The default network is also active during such directed tasks as thinking about one’s past (autobiographical memory), thinking about the future, or understanding a story. The cerebral cortex can be subdivided into a mosaic of areas that can be identified based on differences in function, cytoarchitecture, connectivity, and topography (the FACT approach) For example, the mouse cortex has about 41 distinct areas, the rhesus monkey about 140, and the human 180 (van Essen, Donahue, & Glasser, 2018). Overall, humans also have a higher density of cortical neurons than other mammals. The increased neuronal density means that the humans actually have more neurons in their brains than do animals with much larger brains, such as whales and elephants. A final special characteristic of the human brain is a difference in some classes of cortical neurons. For example, one class of cortical neuron, von Economo neurons, is found only in humans; other great apes; and possibly in macaques, cetaceans, and elephants — but these neurons are most abundant in humans (Cauda et al., 2014). These large bipolar neurons are located in the dorsolateral prefrontal cortex and in deep layers of the insula and in a lateral cortical region of the anterior cingulate cortex John Allman and his colleagues (2005) proposed that von Economo neurons are associated with the emergence of theory of mind. Even more provocatively, Allman speculated that these cells fail to develop normally in people with autism spectrum disorder (ASD), leading to the faulty social intuition characteristic of the disorder (see Section 24.3). Cerebral Asymmetry Split Brain research in 1960’s by Roger Sperry and Michael Gazzaniga Patients Had either whole or parts of Corpus Callosum removed Lateralization - refers to functions that may be reliant on a particular hemisphere Eg. Language is lateralized on the left hemisphere Hemisphere Specialization- refers to a hemisphere dedicated largely to some specific functions eg Left Hemisphere is specialized at language The single most curious feature of human brain organization is cerebral asymmetry: the left and right cerebral hemispheres have partly separate functions. Five variables complicate research on laterality: 1. Laterality is relative, not absolute. Both hemispheres participate in nearly every behavior; thus, for example, although the left hemisphere is especially important for producing language, the right hemisphere also has many language capabilities. 2. Cerebral site is at least as important in understanding brain function as cerebral side 3. Environmental and genetic factors affect laterality- Handedness and sex(females) 4. A range of animals exhibit laterality 5 Function as though there is a single mind Anatomical Asymmetries MRI scans of living brains confirm eight major anatomical differences between the two hemispheres: 1. The right hemisphere is slightly larger and heavier than the left, but the left contains more gray matter (neurons) relative to white matter(connections). 2. The marked structural asymmetry of the left and right temporal lobes may provide an anatomical basis for their observed specialization in language and in music functions, respectively. 3. The anatomical asymmetry in the temporal lobes’ cortex correlates with an asymmetry in the thalamus, This asymmetry complements an apparent functional asymmetry in the thalamus: the left thalamus is dominant for language functions. 4. The slope of the lateral fissure is gentler on the left hemisphere than on the right. The region of the temporoparietal cortex lying ventral to the lateral fissure therefore appears larger on the right. (Toga & Thompson, 2003.) 5.. The frontal operculum (Broca’s area) is organized differently on the left and right. The area visible on the brain surface is about one-third larger on the right than on the left, whereas the area of cortex buried in the region’s sulci (ridges) is greater on the left than on the right. This anatomical asymmetry probably corresponds to the lateralization of these regions, with the left side affecting grammar production and the right side possibly influencing tone of voice (prosody). 6. The distribution of various neurotransmitters is asymmetrical in both the cortical and the subcortical regions. The particular asymmetries in the distribution of ACh, GABA, NE, and DA depend on the structure under consideration. 7. The right hemisphere extends farther anteriorly than does the left, the left hemisphere extends farther posteriorly than does the right, and the occipital horns of the lateral ventricles are five times as likely to be longer on the right as on the left. The left/right asymmetry in frontal and parieto-occipital regions is known as cerebral torque, or Yakovlevian torque. 8. Meta Analysis found generally thicker cortex in the left hemisphere but larger surface area in the right hemisphere. Neuronal Asymmetry Arnold Scheibel and his colleagues (1985) compared the dendritic fields of pyramidal cells in Broca’s area, the left frontal operculum (LOP), with those in the facial area of the motor cortex in the left precentral cortex (LPC) and with homologous regions in the right hemisphere. Their results show that neurons in each region have distinct patterns of dendritic branching, as diagrammed in Figure 11.3. The degree or pattern of branching is important because each branch is a potential location for enhancing or suppressing graded potentials in the dendritic tree (see Section 4.4). More branch points allow the cell more degrees of freedom with respect to its function. Note the abundant branches in neurons in Broca’s area (LOP), where there are far more branches than in the other areas. Genetic Assymetry The Human Genome Project, completed in 2003, allows investigators to address the genetic regulation of cerebral asymmetry. Tao Sun and colleagues (2006) compared gene expression levels in the perisylvian regions of the left and right hemispheres of the fetal brain. They found genes that are expressed differently in the two hemispheres, and it is reasonable to predict that epigenetic changes may differentially influence the two hemispheres In fact, the differences in gene expression can be seen in embryonic and fetal human brains, with the youngest brains studied being 5 weeks postconception (de Kovel et al., 2018). Asymmetries in Patients 1930s, Wilder Penfield- Epilepsy surgery Careful study of hundreds of patients in Montreal by Penfield and his students and more recently by George Ojemann (1983) and his colleagues at the University of Washington have provided clear evidence of cerebral asymmetry. Anesthetizing of the left hemisphere can block the ability to speak, whereas stimulation of the right hemisphere seldom does so. ( eg. WADA testing using sodium amobarbital injections) 1. The brain has symmetrical as well as asymmetrical functions. 2. The right hemisphere has perceptual functions not shared by the left Hemisphere 3.Stimulating the left frontal or temporal region may accelerate speech production. Commissurotomy- 1940s by William Van Wagnen 1960s, when research with monkeys and cats by Ron -Myers and by Roger Sperry - Joseph Bogen and Philip Vogel Split Brain studies - Roger Sperry and Gazzaniga Individual Differences in Cerebral Organization No two brains are alike Two Major Factors Sex of the Individual and Handedness Historically, left-handedness has been viewed as odd at best. But it is not rare. When looking solely at writing preference, an estimated 10% of the human population worldwide is left handed. Hand preference appears early in fetal development: by 10 weeks of age, 75% of fetuses show a strong preference for right-hand movements and starting at the fifteenth gestational week, 90% of fetuses prefer right thumb sucking Overall studies of cortical thickness and surface area using MRI have failed to find any consistent difference between left- and right-handers. One MRI study did find a difference in the size of the motor cortical hand area: it was larger in the left hemisphere for right-handers and larger in the right hemisphere for left-handers (Germann et al., 2019). Katrin Amunts and colleagues (2000) found that male right-handers have a significantly deeper fissure on the left than on the right but found no difference in left-handers. Graham Ratcliffe and his colleagues (1980) correlated the asymmetry in the course (angle) of the Sylvian (lateral) fissure -with the results of carotid sodium amobarbital speech testing (see Figure 11.12). They found that left- and right-handers with speech in the left hemisphere had a mean right–left difference of 27° in the angle formed by the vessels leaving the posterior end of the Sylvian fissure. In left and right-handers with speech in the right hemisphere or with bilateral speech, the mean difference shrank to 0°. Handedness may appear to be closely related to anatomical anomalies because left-handers display more variation in speech lateralization. Apparently, more fibers descend to the right hand both from the contralateral left hemisphere and from the ipsilateral right hemisphere than descend to the left hand Sandra Witelson (1989) studied the hand preference of terminally ill patients on a variety of unimanual tasks. During postmortem studies of the patients’ brains, paying particular attention to the size of the corpus callosum, she found that the cross-sectional area was 11% greater in left-handed and ambidextrous people than in right-handed people Eileen Luders and her colleagues (2010) summarized the results of 20 such studies and concluded that if there is a difference in callosal size, it is rather small. They also made another interesting conclusion: as a group, left-handers are less lateralized in their hand dominance than right-handers Given that left-handers are generally less lateralized, we can see how they might appear to have larger corpus callosum The middle portions of the callosum are heavily involved in transferring motor information, which might mean that a smaller callosum in people with strong hand preference is related to diminished interhemispheric communication. Handedness is not related to the anterior region of the callosum but rather to the prefrontal regions, which are involved in processing higher-order cognitive operations. This may imply that individuals with different degrees of handedness lateralization have somewhat different cognitive processing. Charles Branch and colleagues (1964) found that, in left-handers, language is represented in the left hemisphere in 70%, in the right hemisphere in 15%, and bilaterally in 15%. Doreen Kimura (1983) reported the incidence of aphasia and apraxia in a consecutive series of 520 patients selected for unilateral brain damage only. The frequency of left-handedness in her population was within the expected range, and these patients did not have a higher incidence of either aphasia or apraxia than right-handers did. In fact, the incidence of aphasia in left-handed patients was approximately 70% of the incidence in right-handers, exactly what would be predicted from the sodium amobarbital studies. Thus, although a small proportion of left-handers have bilateral or right-hemisphere speech, the majority do not. Familial left-handers perform much differently, suggesting to Hécaen that they have a different pattern of cerebral organization. Sex Differences in Cerebral Organization Melissa Hines (2015) argued that the biggest sex difference in children’s behavior can be seen in play. Beginning at about 7 weeks’ gestation, the testes begin to produce testosterone, leading to a vast difference in testosterone concentrations in males and females prenatally. Bonnie Auyeung and her colleagues (2009) found a significant correlation between fetal testosterone and sexually dimorphic play in both girls and boys. Given that the parents did not know their children’s testosterone levels, it is unlikely that socialization accounts for the sex difference. In Sex and Cognition, Kimura (1999) examined five classes of cognitive behaviors and found compelling sex differences in all: motor skills, spatial analysis, mathematical aptitude, perception, and verbal abilities Table 12.2 summarizes her major conclusions and shows that the verbal–spatial dichotomy just noted is a gross oversimplification Sex differences in anatomy 1.Asymmetry (left larger than right) in the planum temporale (Wernicke’s area) is seen more often in men than in women. In fact, an MRI study by Jennifer Kulynych and her colleagues (1994) found a large asymmetry in males (left 38% larger) but less asymmetry in females. Agnès Dorion and colleagues (2000) found a similar result but noted in addition that the absolute magnitude of cerebral asymmetry was negatively correlated with the size of the corpus callosum in males but not in females. This suggests a sex-dependent decrease in hemispheric connectivity with increasing hemispheric asymmetry. 2.Sandra Witelson and Debra Kigar (1992) quantified the slope of the Sylvian (lateral) fissure with reference to various cortical landmarks, which led to a separate measure of its horizontal and vertical components (Figure 12.8A). They found that, although the horizontal component was longer in the left hemisphere of both sexes, men had a larger horizontal component in the left hemisphere than did women. There was no difference in the right hemisphere. Thus, Witelson and Kigar found that male brains have a larger asymmetry in the Sylvian fissure than do female brains. Taken together, the results of the studies of the planum temporale and the Sylvian fissure reinforce evidence for a sex difference in the organization of language-related functions. 3.The asymmetry in the planum parietale, which favors the right hemisphere, is about twice as large in men as in women (Jancke et al., 1994). 4. Numerous studies have found that women have more interhemispheric connections, in both the corpus callosum and the anterior commissure, than do men. women have a larger anterior commissure than do men, even without correcting for brain size. This result is likely due to a difference in the number of neural fibers in the two sexes, which presumably affects how the two hemispheres interact. 5. The ridges in our fingerprints, established early in fetal life, are asymmetrical: the fingers on the right hand have more ridges than do fingers on the left hand. Kimura (1999) found that most people have the asymmetry, but women are far more likely to show an atypical pattern, much as seen for brain asymmetries. The critical part of the Kimura studies (and later studies by others) is that the pattern of ridges is correlated with performance on certain cognitive tests. Disorders linked to Cortical Asymmetries Autism Functional imaging data have indicated that people with ASD have reduced leftward language lateralization more frequently than healthy controls (Kleinhans, Muller, Cohen, & Courchesne, 2008; Knaus et al., 2010; Lindell & Hudry, 2013).. rightward shift of asymmetry that involves various functional networks ASD have a higher rate of left‐handedness brain structural imaging studies have reported altered hemispheric asymmetry in ASD, including studies of white matter tracts (Carper, Treiber, DeJesus, & Muller, 2016; Conti et al., 2016; Joseph et al., 2014), gray matter volume, surface and thickness (Dougherty, Evans, Katuwal, & Michael, 2016; Floris et al., 2016). Regions involved in perceptual and cognitive processing, including medial frontal, anterior cingulate and inferior temporal regions, overlap with the default mode network (DMN) Major Depressive Disorder neurophysiological asymmetry between individuals with MDD and healthy controls, particularly involving reductions of left frontal and right parieto‐ temporal function in depressive disorders (Bruder, Stewart, & McGrath, 2017; Coan & Allen, 2003; Davidson, 1998; Jesulola, Sharpley, Bitsika, Agnew, & Wilson, 2015; van der Vinne, Vollebregt, van Putten, & Arns, 2017). Poorer integration of left and right hemispheres OCD Altered functional laterality has been investigated in OCD (Abramovitch, Abramowitz, & Mittelman, 2013; Kuelz, Hohagen, & Voderholzer, 2004), partly stemming from observations of psychometric deficits within the visual–spatial domain (typically rightward lateralized in healthy people) (Kuskowski et al., 1993; Maril, Hermesh, Gross‐Isseroff, & Tomer, 2007; Rao et al., 2015),- right occipital parietal regions as well as altered emotional processing (again some aspects of emotion are typically rightward lateralized) (Ischebeck, Endrass, Simon, & Kathmann, 2014; Schienle, Schafer, Stark, Walter, & Vaitl, 2005; Simon, Kaufmann, Musch, Kischkel, & Kathmann, 2010; Wexler & Goodman, 1991). However, left‐ sided dysfunction has also been suggested in OCD, on the basis of neuropsychological data (Wexler & Goodman, 1991) ADHD caudate, thalamus, hippocampus, and amygdala for lateralized comparisons between the ADHD and ADHD-free groups- Increased hyperactivity and inattention scores correlated with increased asymmetry in fusiform cortex Left hemisphere differences were significant in inferior fronto-occipital( should be more right hemisphere dominant) LD Earliest studies show lack of asymmetry in Planum Temporale. In neurotypical participants - Planum Temporale is larger in the left hemisphere. This asymmetry is lacking in individuals with LD