Nester's Microbiology A Human Perspective PDF
Document Details
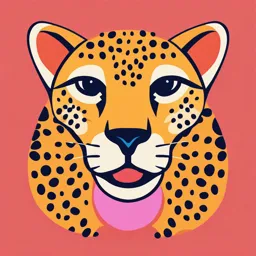
Uploaded by InexpensiveSilver
Denise Anderson, Sarah Salm, Mira Beins
Tags
Summary
This document is a lecture outline covering biotechnology, focusing on DNA-based technologies, genomics and proteomics. Topics explored include applications, techniques (such as PCR, cloning, sequencing), and relevant case studies. It is aimed at undergraduate-level biology students.
Full Transcript
Because learning changes everything. ® Chapter 9 Biotechnology Lecture Outline Nester's Microbiology A Human Perspective, Tenth Edition Denise Anderson, Sarah Salm, Mira Beins © 2022 McGraw Hill, LLC. All rights reserved. Authorized only for instructor use in the classroom. No reproduction or furt...
Because learning changes everything. ® Chapter 9 Biotechnology Lecture Outline Nester's Microbiology A Human Perspective, Tenth Edition Denise Anderson, Sarah Salm, Mira Beins © 2022 McGraw Hill, LLC. All rights reserved. Authorized only for instructor use in the classroom. No reproduction or further distribution permitted without the prior written consent of McGraw Hill, LLC. A Glimpse of History “Dirty War” in Argentina (1976 to 1983) • Military junta killed thousands of citizens • Approximately 200 children survived killings; had been kidnapped and placed with pro-junta families • Mary-Claire King (then at UC Berkeley) analyzed DNA sequences to reunite children with relatives • Parents usually dead or missing making chromosomal DNA difficult to use • King used mitochondrial DNA (mtDNA), which is inherited only from the mother, to match children with siblings, the mother’s siblings, and the maternal grandmothers © McGraw Hill, LLC 2 Applications of DNA-Based Technologies 1 Use of microbiological and biochemical techniques to solve practical problems • Traditionally included searching for naturally occurring mutants that have desirable characteristics • Today biotechnology is generally associated with procedures that involve manipulating DNA • In the 1970s methods were developed alter a bacterium’s genetic information using in vitro techniques • A process called genetic engineering • Scientists can now genetically alter yeast and mammalian cells growing in culture providing important laboratory models for studying cell functions. © McGraw Hill, LLC 3 Applications of DNA-Based Technologies 2 Microorganisms and animal cells can be genetically engineered to produce commercially valuable proteins. • Creates protein sources that are reliably available and less expensive than traditional sources, such as animal tissues Plants and animals can be genetically engineered making genetically modified organisms (GMOs) Technically a GMO is any organism that has been genetically engineered, including bacteria and yeasts © McGraw Hill, LLC 4 New Fields Resulting from Biotechnology Genomics is the study and analysis of genomes • Spurred by advances in DNA sequencing technologies Transcriptomics is the study of RNA molecules and their functions • Study of mRNA and non-coding RNAs • Studies of gene expression to determine how cancer cells escape the mechanisms that normally limit cell multiplication • Studies of host-pathogen interactions that result in disease Proteomics is the study of proteins, their functions, and their expression profile (conditions that promote the expression of various proteins by a cell or group of cells) • Important role in cancer studies and infectious disease research © McGraw Hill, LLC 5 Affects of Biotechnology on Society 1 • Cancer treatment - DNA manipulation has revolutionized cancer treatment • Diagnostic tests used in disease diagnosis often rely on detection of nucleotide sequences unique to the pathogen • DNA typing (“DNA fingerprinting”) used in forensics relies on the polymerase chain reaction (PCR) • Food production – the protein chymosin (rennin), an enzyme used in cheese production; is one of the most widely used proteins produced by genetically engineered microorganisms • Gene therapy – corrects life-threatening genetic defects, including myotubular myopathy (MTM), which causes extreme muscle weakness © McGraw Hill, LLC 6 Affects of Biotechnology on Society 2 • Precision medicine uses a patient’s genetic makeup as a basis for treatment • Research - numerous scientific discoveries have relied on genetic engineering or other biotechnologies • Therapeutic proteins - pharmaceutical proteins, such as insulin, can be produced through genetic engineering • Transgenic crops - crops have been genetically engineered to resist insect pests by making Bt toxin • Vaccines - genetically engineered microorganisms can produce proteins for use in vaccines © McGraw Hill, LLC 7 Fundamental Tools Used in Biotechnology - Figure 9.1 Restriction enzymes cut DNA strands in at a recognition sequence • Generates restriction fragments • Complementary “sticky” ends can anneal with one another • DNA ligase joins molecules covalently • Allows creation of recombinant DNA molecules Access the text alternative for slide images. © McGraw Hill, LLC 8 Table 9.2 Examples of Common Restriction Enzymes Restriction enzymes recognize a 4 to 6 base-pair nucleotide sequence • Palindromes: the same on both strands when read in 5′ to 3′ direction • Name represents bacterium from which it was isolated Recognition Sequence (Arrows Indicate Cleavage Sites) Enzyme Microbial Source Alul Arthrobacter luteus ↓ 5′ A G C T 3′ 3′ T C G A 5′ ↑ BamHI Bacillus amyloliquefaciens H ↓ 5′ G G A T C C 3′ 3′ C C T A G G 5′ ↑ EcoRI Escherichia coli RY13 ↓ 5′ G A A T T C 3′ 3′ C T T A A G 5′ ↑ © McGraw Hill, LLC 9 Reverse Transcriptase - Figure 9.2 Makes DNA from an RNA template to create an intron free copy of a gene • Isolate mRNA • Reverse transcribe to single DNA strand (no introns) • DNA polymerase synthesizes complementary strand to form double-stranded cDNA Access the text alternative for slide images. © McGraw Hill, LLC 10 DNA Gel Electrophoresis • A technique to separate DNA fragments by size • DNA samples are added to wells near one end of a slab of agarose or polyacrylamide gel that has been placed in an apparatus containing a buffer (an electrically conductive solution) • A mixture of DNA fragments of known size is also added to one or more wells allowing the sizes of the fragments to be determined • An electric current is then run through the gel • DNA is negatively charged, so fragments move toward the positive electrode located at the end of the slab opposite the wells • Short fragments of DNA move easily and faster through the gel than larger fragments • Fragments separate based on size • Stain makes bands of DNA fragments visible © McGraw Hill, LLC 11 DNA Gel Electrophoresis - Figure 9.3 ©McGraw-Hill Education/Lisa Burgess, photographer Access the text alternative for slide images. © McGraw Hill, LLC 12 Molecular Cloning Introduces foreign or altered DNA to recipient cell • Replicated and passed on to cell’s progeny • DNA Segment is inserted into existing plasmid or other replicon, also called a vector • Replication enzymes makes this possible • Restriction enzymes create a recombinant DNA molecule • Vectors are commercially available © McGraw Hill, LLC 13 The Cloning Process - Figure 9.4 DNA cloning • Isolate DNA of interest • Cut with restriction enzyme • Join insert (DNA fragment) with vector (plasmid) to generate recombinant molecule • Introduce into host where it will replicate • High-copy-number vector makes large amounts of protein Access the text alternative for slide images. © McGraw Hill, LLC 14 Applications of Molecular Cloning Useful for: • Protein production • DNA production • Research tools © McGraw Hill, LLC 15 Creating a DNA Library - Figure 9.5 DNA library: collection of clones that together contain the entire genome of an organism • Cut DNA of organism with restriction enzymes • Clone all fragments into population of E. coli cells • Each cell contains one fragment of genome • Other techniques can find gene of interest within the library Access the text alternative for slide images. © McGraw Hill, LLC 16 Obtaining DNA The first step of cloning is to obtain DNA from the bacterium of interest • Add a detergent to cells in a broth culture to lyse them • DNA released from the lysed bacteria is separated from debris by precipitation using salt and alcohol When cloning eukaryotic genes into bacteria for protein production, a researcher must first obtain a copy of DNA that lacks introns • Done using reverse transcriptase to create cDNA © McGraw Hill, LLC 17 Generating a Recombinant DNA Molecule Cut the DNA of interest and the vector molecules with a restriction enzyme Combine the two types of DNA along with DNA ligase to form recombinant molecules Vectors typically have the following: • Multiple-cloning site - a short nucleotide sequence that includes the recognition sequences of several restriction enzymes • Selectable marker - usually a gene that encodes resistance to an antibiotic to eliminate any cells that have not taken up vector • Second genetic marker - gene that encodes an observable characteristic to identify colonies that contain recombinant molecules © McGraw Hill, LLC 18 Generating a Recombinant DNA Molecule - Figure 9.6 Access the text alternative for slide images. © McGraw Hill, LLC 19 Obtaining Cells That Contain Recombinant DNA Molecules- Figure 9.7 Vector pUC18 selects for cells with vector and differentiates those with recombinant plasmids • Selectable marker is gene for ampicillin resistance • Cells with intact or recombinant vector grow • Second genetic marker: lacZ′ gene • Product cleaves X-gal forming a blue compound • Insert interrupts lacZ′ • Intact vector → blue colonies • Recombinant molecule → white colonies ©2014 Edvotek, Inc. All Rights Reserved. www.edvotek.com Access the text alternative for slide images. © McGraw Hill, LLC 20 CRISPR-Cas Technologies - Figure 9.8a Access the text alternative for slide images. © McGraw Hill, LLC 21 CRISPR-Cas Technologies Used for gene editing - introduce targeted changes into the nucleotide sequence of a living cell’s genome • CRISPR systems were discovered in bacteria which use them to recognize and destroy invading DNA • Their discover gave scientists an entirely new range of powerful biotechnology tools • Scientists originally worked Cas9 • Now various types of Cas nucleases have been engineered for different tasks • Cas nucleases use a piece of single-stranded RNA (gRNA) as a guide to recognize a particular DNA or RNA sequence within a cell © McGraw Hill, LLC 22 Applications of CRISPR-Cas Technologies gRNA directs the CAS nuclease to make a double-stranded cut at a precise location in the DNA • Location of the cut is controlled by synthesizing gRNA with the desired sequence • The nucleotide sequence at cut site can be changed by manipulating the cell’s normal DNA repair mechanisms • System now widely used to precisely modify an organism’s DNA as a way to study gene function • Clinical trials are assessing its use in gene therapy to correct genetic disorders in humans © McGraw Hill, LLC 23 Applications of CRISPR-Cas Technologies - Figure 9.9 “Dead” or dCas9 are used to study gene function • Does not cut DNA, but still uses RNA guide to bind to specific DNA sequence • May block RNA polymerase from transcribing the gene and turn the gene off • May deliver molecules to specific chromosome locations • Carry an activator to turn gene on • Fluorescent marker to locate a gene Access the text alternative for slide images. © McGraw Hill, LLC 24 Disease Diagnosis Using CRISPR-Cas Technologies Cas systems are used for rapid diagnosis of infectious diseases or cancers • Diagnostic kits being developed all rely on at least two components: • A Cas nuclease coupled with gRNA to recognize a nucleotide sequence for a specific disease • A mechanism to visibly detect that recognition • In 2020, the U.S. Food and Drug Administration (FDA) gave emergency-use authorization for a rapid COVID-19 diagnostic test that relies on CRISPR-Cas technologies • Results can be obtained in about an hour © McGraw Hill, LLC 25 DNA Sequencing 1 Determining the order of nucleotides in a DNA molecule • New highly automated and efficient methods have revolutionized genomics and permitted large scale projects • Human Genome Project in 1990 led the revolution • Sequenced the entire human genome • Led to the Human Microbiome Project (2007) to characterize microbial communities that inhabit the human body • The Earth BioGenome Project (2018) • Effort to sequence all the known animal, plant, protozoan, and fungal species © McGraw Hill, LLC 26 DNA Sequencing 2 These projects encouraged advances in sequencing technologies • In 1995 it took one year to sequence the first bacterial genome • It can now be done in a few hours! The rapid explosion of data that followed gave rise to a new field called bioinformatics • Uses computer-based methods to organize and analyze the sequence information © McGraw Hill, LLC 27 Applications of DNA Sequencing Comparisons can be made to gain insights into roles and relevance of encoded proteins Provides insight into the evolution of microorganisms • Whole genome sequencing (WGS) used to compare genomes of species • Led to the concept of a pan-genome WGS provides the ability to track pathogen outbreaks Applications are extensive • One example is The Cancer Genome Atlas • Characterized over 20,000 cases of cancer • Lead to better diagnosis and personalized therapies © McGraw Hill, LLC 28 High-Throughput Sequencing Methods - Figure 9.10 Next generation (“next-gen”) methods Highly automated; fast, lower costs Analyze millions of overlapping small DNA fragments Computers align and merge data to create entire sequence • Process called sequence assembly • Easier using reference genome Errors are common so genomes are analyzed multiple times Data Alignment reading th reading th keep read keep read ing the textbook ing the textbook © McGraw Hill, LLC Result keep reading the textbook 29 Nanopore Sequencing • Long fragments of DNA in electrically conductive solution • Passed through microscopic pore where different nucleotides block electric current to varying degrees • Recorded disruptions reflect nucleotide sequence • Used to sequence microbial DNA on International Space Station © McGraw Hill, LLC 30 RNA-Seq (RNA Sequencing) Relies on high-throughput DNA sequencing Determines nucleotide sequence of an organism's RNA transcripts • Total RNA is isolated from a cell • Desired types of RNA (mRNA, tRNA) are isolated • Reverse transcriptase used to create cDNA • Used to study gene expression and may replace PCR © McGraw Hill, LLC 31 Polymerase Chain Reaction (PCR) - Figure 9.11 Allows creation of over a billion copies of a given region of DNA in hours • PCR product can be visualized via gel electrophoresis • Allows detection of specific sequences • Can detect pathogens without culturing Access the text alternative for slide images. © McGraw Hill, LLC 32 Applications of PCR RT-PCR (reverse-transcription PCR) • Reverse transcriptase used to synthesize cDNA from mRNA template in a sample • cDNA is template for amplification qPCR (quantitative PCR) • Also called real-time PCR • Fluorescent marker detects PCR product • Tracks amplification in real time • Determines relative amount of target DNA in sample Real-time RT-PCR • Combines RT-PCR and qPCR to determine the relative amount of a given RNA sequence in a sample © McGraw Hill, LLC 33 PCR Method Process involves DNA synthesis reactions and the following: • Double-stranded DNA with target sequence to serve as template • Taq polymerase: heat-stable DNA polymerase from Thermus aquaticus • Primers to determine which portion of template DNA will be amplified • Deoxynucleotides (dATP, dGTP, dCTP, dTTP) © McGraw Hill, LLC 34 Three-step Amplification Cycle - Figure 9.12 1. DNA denatured by heating (approximately 95 degrees Celsius) 2. Temperature lowered (approximately 50 degrees Celsius) to allow primers to anneal 3. Temperature raised (approximately 70 degrees Celsius) to allow DNA synthesis • Target DNA doubled in each cycle, so exponential increase • Heat-stable Taq polymerase from Thermus aquaticus critical Access the text alternative for slide images. © McGraw Hill, LLC 35 Generating the PCR Product - Figure 9.13 Primers produce PCR product containing only target sequence Access the text alternative for slide images. © McGraw Hill, LLC 36 Selecting Primers - Figure 9.14 • The two primers used in PCR dictate which part of the DNA is amplified. • Each primer is complementary to one end of the target DNA • DNA synthesis will extend across that stretch of DNA • To amplify a DNA sequence encoding a specific protein the nucleotide sequences at the ends of the gene must be known Access the text alternative for slide images. © McGraw Hill, LLC 37 Focus on a Case 9.1 Amplification of short tandem repeats (STRs) • STRs repeat consecutively a variable number of times • Usually within intron or other untranslated region • CODIS database contains amplification patterns of 20 different STR loci and allows unique identification Access the text alternative for slide images. © McGraw Hill, LLC 38 Probe Technologies - Figure 9.15 DNA probes locate specific nucleotide sequence • Probe is single-stranded piece of DNA, complementary to sequence of interest, labelled with a marker • Anneals to complement through hybridization • Makes sequence detectable Access the text alternative for slide images. © McGraw Hill, LLC 39 Colony Blotting - Figure 9.16 Colony blotting uses probes to detect specific DNA sequence in colonies grown on agar plates • Commonly used to identify which clones in a collection contain sequence of interest Access the text alternative for slide images. © McGraw Hill, LLC 40 Florescence In Situ Hybridization - Figure 9.17 Fluorescence in situ hybridization (FISH) • Fluorescently labeled probe hybridizes to nucleotide sequences in intact cells fixed to microscope slide • Cells visualized via fluorescence microscopy • Probe often binds to rRNA sequences present in high copy number • Rapid identification in specimen without culturing • Detects related organisms or specific species ©Seana Davidson • Two different markers can detect two different groups in a sample © McGraw Hill, LLC 41 DNA Microarrays A DNA microarray (gene chip) tens or hundreds of thousands of DNA spots are placed on a glass slide or other solid support • Each spot contains many copies of a specific oligonucleotide (short DNA sequence) which functions like a probe • Each spot has a different oligonucleotide and acts as a different probes - each spot is a unique probe • Used to study gene expression in organisms whose genomes have been sequenced Versions of microarrays referred to as biochips are being developed to identify bacterial pathogens in clinical specimens The goal is to screen for dozens of pathogenic microorganisms simultaneously and predict antibiotic susceptibility © McGraw Hill, LLC 42 DNA Microarrays - Figure 9.18 To study gene expression, mRNA is isolated from an organism and used to synthesize labeled, single stranded cDNA • cDNA added to microarray and allowed to hybridize • Fragments complementary to the oligonucleotides will anneal • Oligonucleotides which hybridize labeled cDNAs represent nucleotide sequences of genes that the organism was expressing © McGraw Hill, LLC Deco/Alamy Stock Photo 43 Concerns Regarding Genetic Engineering New technologies need to be tested for safety • Recombinant DNA Advisory Committee (RAC) developed guidelines for using recombinant DNA and cloning • Numerous benefits, but potential for malicious use • Creation of new infectious agents for bioterrorism • Genetically modified (GM) organisms hold promise • Concerns over possible allergens • Unintended effects on environment • Pollen from Bt plants may or may not harm butterflies • Herbicide-resistant genes may transfer to weeds • Genetic changes from CRISPR/Cas9 technologies may be difficult to detect © McGraw Hill, LLC 44 Because learning changes everything. www.mheducation.com © 2022 McGraw Hill, LLC. All rights reserved. Authorized only for instructor use in the classroom. No reproduction or further distribution permitted without the prior written consent of McGraw Hill, LLC. ®