Myelophthisic Anemias PDF
Document Details
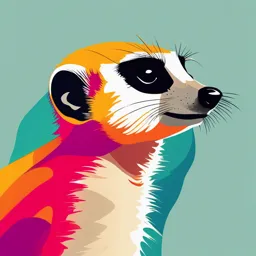
Uploaded by UsefulWichita2702
Tags
Summary
This document provides an overview of myelophthisic anemias, a group of blood disorders characterized by bone marrow fibrosis. It discusses the various causes, including metastatic cancers, infections, and hematological syndromes. The document also explains the pathophysiology and clinical symptoms.
Full Transcript
Myelophthisic anemias Here’s the translation of the provided text: --- **Sometimes, for various reasons, fibrosis occurs in the bone marrow. In these cases, a smear of blood shows a picture referred to as leukoerythroblastosis. The fibrosis that occurs in the bone marrow can sometimes present as...
Myelophthisic anemias Here’s the translation of the provided text: --- **Sometimes, for various reasons, fibrosis occurs in the bone marrow. In these cases, a smear of blood shows a picture referred to as leukoerythroblastosis. The fibrosis that occurs in the bone marrow can sometimes present as a primary bone marrow disease known as primary myelofibrosis or myeloid metaplasia. At other times, bone marrow fibrosis may be a secondary or reactive process referred to as myelophthisis or secondary myelofibrosis.** Several categories of diseases can cause secondary myelofibrosis: 1. The first group includes metastatic cancers, such as epithelial breast cancer, lung cancer, prostate- origin cancers, and neuroblastomas. *Note: Any type of malignancy can affect the bone marrow, with some having a higher prevalence than others.* 2. The second group of diseases causing bone marrow fibrosis includes various infectious diseases, such as infections with mycobacteria (both Mycobacterium tuberculosis and atypical mycobacteria such as avium), fungi, HIV, and sarcoidosis. 3. The third group of diseases causing bone marrow fibrosis comprises hematologic syndromes, especially diseases like multiple myeloma, lymphomas, hairy cell leukemia, and chronic myeloid leukemia (CML). **Pathophysiology of Bone Marrow Fibrosis:** The pathophysiology of this fibrosis has three distinct features: 1. **Proliferation of fibroblasts** within the bone marrow (leading to myelofibrosis). 2. **Extension and spread of hematopoietic cells** from within the bone marrow to extramedullary sites, such as long bones, spleen, liver, and lymph nodes; this phenomenon is called myeloid metaplasia. 3. It leads to **ineffective erythropoiesis**, meaning that every cell produced, despite potentially having a high proliferation rate, is unhealthy, has a short lifespan, and quickly dies off. Despite having a significant number of circulating hematopoietic progenitor cells, myelofibrosis in these patients causes various cytopenias, including pancytopenia. Anemia is the predominant feature of this secondary fibrosis, and their anemia is often normocytic and normochromic. The diagnosis of this secondary fibrosis is made using the leukoerythroblastic smear pattern observed in the peripheral blood smear. In myelofibrosis, the morphology of erythrocytes is highly abnormal, and nucleated RBCs (nRBCs), teardrop-shaped red blood cells, and various other RBC shapes can be observed in peripheral blood analysis. Sometimes, the WBC count in these patients increases significantly, leading to a leukemoid reaction, where WBC counts can reach 30,000 to 50,000. When white blood cells increase, we can also observe immature myeloid lineage cells in the peripheral blood, such as myeloblasts, promyelocytes, and myelocytes. Sometimes, the platelet count in these patients is very high, and their sizes are large. **A significant feature of patients with bone marrow fibrosis** is that when we attempt to perform a biopsy or bone marrow aspiration, they are "tap dry," meaning we are unable to aspirate the bone marrow. The clinical course of secondary myelofibrosis is determined by the etiological factor that caused it. For instance, metastatic cancers and advanced hematologic malignancies have their unique clinical courses. When facing secondary myelofibrosis, our first task is to investigate treatable diseases, such as infections, and initiate their treatment. In addition to treating the underlying disease causing secondary myelofibrosis, we can also provide blood transfusions during treatment to alleviate the symptoms in these patients. In this smear of peripheral blood, a leukoerythroblastic pattern is seen, with RBCs and teardrop-shaped red blood cells. The presence of abnormal red blood cells in the white blood cell lineage also shows an immature cell called a myelocyte. **Iron Deficiency Anemia** The primary function of RBCs is to carry hemoglobin, and the main role of hemoglobin is to transport oxygen. The hemoglobin content in RBCs requires a coordinated production of globin protein, porphyrin ring synthesis, and accessibility to iron. Deficiencies in any of these three essential elements can lead to hypochromic or microcytic anemias. Iron deficiency is the most common cause of anemia worldwide, and primary care physicians encounter many patients with iron deficiency anemia. Here’s the translation of the provided text: --- **It is estimated that about 2% to 5% of young women and adolescents in the United States suffer from iron deficiency. Certainly, the prevalence of iron deficiency is higher in other countries and communities, with approximately 10% of the world's population, over 500 million people, experiencing iron deficiency. In developing countries, where food scarcity and gastrointestinal parasites are more prevalent, the incidence of iron deficiency is significantly higher.** In an adult body, there is approximately 4 grams of iron, of which 2100 mg is associated with hemoglobin, 300 mg is related to myoglobin found in muscles, 1000 mg is stored in the liver, and 600 mg is in the macrophage system of the bone marrow and spleen. From this amount of iron, about 3 to 7 mg is circulating in the blood, but this 3 to 7 mg is kinetically active and changes every 3 to 4 hours, participating in cellular turnover. On average, about 1 to 2 mg of iron is lost normally from our bodies, and we need to replenish this amount. This 1 to 2 mg is lost through various means, such as the shedding of mucosal cells, the shedding of epidermal cells from the skin, and in women of childbearing age, through menstruation, etc. **What has led to the selection of iron in mammals for the function of oxygen transport?** Iron’s ability to gain and lose electrons (changing from the ferrous (Fe²⁺) form to the ferric (Fe³⁺) form and vice versa) has made it a vital element in the porphyrin ring, as well as in the construction of many enzymes in our bodies. Free iron is very toxic to our bodies; therefore, iron does not exist freely within our system. The primary iron transport protein in the blood plasma is transferrin. The main stored form of iron within cells is ferritin. **Iron Cycle in the Human Body:** Iron, after being absorbed from food and released from the reticuloendothelial system, binds to transferrin. Transferrin is a bifunctional glycoprotein with the ability to bind two iron atoms. The form associated with one iron atom is called monoferric, while the form of transferrin that binds to two iron atoms is called diferric. The iron-transferrin complex circulates in blood plasma until it interacts with transferrin-specific receptors. When transferrin is bound to two iron atoms, it has the highest affinity for its receptors, while apotransferrins (transferrin molecules that do not carry iron) have the lowest affinity for these receptors. Transferrin receptors are found on many cell types throughout the body, but the cells with the highest concentration of transferrin receptors are developing erythroblasts. When iron-bound transferrin attaches to its receptor, it is internalized by the cell and enters an acidic endosome within the cell, where, at low pH, iron is released from transferrin. After iron is separated from transferrin, it exerts its primary function in the cell; for example, if the cell is responsible for synthesizing hemoglobin, iron is used to produce hemoglobin or for enzyme synthesis. After iron is separated from transferrin and fulfills its role, the receptor along with transferrin returns to the cell surface, and transferrin is released back into the blood. When transferrin is about to be released into the blood, some transferrin receptors are liberated from the cell surface and enter circulation, which we can later measure in the blood. These measurements can be very helpful in the differential diagnosis of anemias. Excess iron utilized in the cell binds to a protein called apoferritin, which then transforms into ferritin and is stored inside the cell. As a result of these exchanges and the iron cycle, red blood cells (RBCs) are ultimately produced, which last approximately 120 days. The bone marrow must turn over and produce about 0.8% to 1% of RBCs daily and release them into the blood. At the end of their lifespan, RBCs are phagocytosed by phagocytic cells of the reticuloendothelial system and are destroyed. When RBCs are removed, the iron contained in them is recycled, which plays a crucial role in providing the iron we need. Each milliliter of RBC is equivalent to 1 mg of iron; therefore, if an adult has about 2 liters of RBCs, approximately 16 to 20 mg of iron is recycled daily. The additional iron needed by the body is obtained through dietary sources. Adult men require about 1 mg of iron daily, which must be absorbed from food. Adult women need about 1.4 mg of iron daily, which must also come from dietary sources, so women require more iron. If an individual’s iron absorption is less than what is needed, their bone marrow becomes hypoproliferative, leading to a decrease in bone marrow cellularity and subsequently causing microcytic hypochromic anemia. Iron balance in the human body is a controlled issue; the essence of iron balance is recycling. There is no regulatory mechanism for the excretion of iron; the only mechanisms we have for losing iron are through menstrual cycles in women, shedding of epithelial cells on the skin, and the mucosa of the gastrointestinal and urinary-reproductive tracts. Iron can enter the body through food consumption and blood transfusions, or it can be administered orally or via injection. The dietary iron content depends on the caloric intake throughout the day; approximately 6 mg of iron is consumed for every 1000 calories ingested daily. The bioavailability of this iron depends on the quality of the food we eat, with red meat having a higher iron absorption rate. In a typical American diet, an adult man consumes about 15 mg of iron daily, of which about 6% is absorbed. In women, around 11 mg of iron enters the body daily, with about 12% of this amount being absorbable. When someone suffers from iron deficiency, they can increase their iron absorption to about 20%, provided their dietary intake is of good quality, such as red meat. However, if the individual's dietary intake is primarily plant-based, the increase in absorption will only be around 5% to 10%.Here’s the translation of the provided text: --- **The presence of phytates and phosphates in plant foods reduces iron absorption. If we compare the absorption rates of iron from food sources and ferrous salts, we can see that only \( \frac{1}{12} \) (one- twelfth) of the iron in plant foods is absorbable, \( \frac{1}{8} \) of the iron in eggs is absorbable, and \( \frac{1}{2} \) to \( \frac{2}{3} \) of the iron in meat is absorbable. Iron is absorbed in the proximal small intestine. For iron absorption to occur effectively, the food must first be acted upon by gastric acid to free iron from food and make it soluble. When iron becomes soluble and is located in the intestinal lumen, it must be converted from the ferric (Fe³⁺) form to the ferrous (Fe²⁺) form at the brush border of the absorptive cells. This conversion is performed by the enzyme ferrireductase.** After iron enters the cell, there is a lateral membrane molecule called ferroportin that carries iron and releases it into the bloodstream. For iron to be released into the bloodstream, it must revert from the ferrous form to the ferric form, which is accomplished by a ferroxidase called hephaestin. It is very important to note that the function of ferroportin is regulated by a crucial regulator called hepcidin, which determines whether ferroportin is allowed to absorb iron and release it into the bloodstream or not. When we consume iron, it must be made soluble by acidic content and absorbed at the level of an intestinal cell before moving inside the cell, being released into the bloodstream by ferroportin, and transported in the blood by transferrin. **What happens that leads to iron deficiency?** - Blood loss for various reasons. - Women of childbearing age and individuals in growth phases may experience iron deficiency. - When the amount of iron intake is insufficient. - When malabsorption occurs at the level of intestinal cells. Iron loss from the gastrointestinal tract can occur for various reasons. In developed countries, iron loss at the gastrointestinal level is typically due to neoplasms, i.e., various cancers occurring in the gastrointestinal tract. Sometimes, these lesions are benign; for example, a person with a small intestine ulcer may lose some blood through this ulcer and become iron deficient. Chronic use of certain medications can cause mucosal damage, leading to blood loss. This is seen in individuals who consume alcohol, take aspirin, or use NSAIDs. These factors gradually lead to blood loss at the gastrointestinal level. In developing countries, a more significant cause of iron loss from the gastrointestinal tract may be gastrointestinal parasites, although neoplastic diseases and gastrointestinal ulcers also play a significant role in these countries. Bleeding in the urinary-reproductive system can occur, such as in women during menstruation. A less common cause of blood loss includes certain hematological diseases, where lysis of RBCs occurs within the vessels, leading to hemoglobinuria. Here’s the translation of the provided text: --- **Each 1 cc of RBCs is equivalent to 1 mg of iron, so for every unit of blood lost, the individual also loses iron. Another less common cause of bleeding occurs in the respiratory system, so any factor that leads to blood loss from the body can be associated with iron deficiency, which later results in anemia. Women of childbearing age and individuals in growth phases may gradually develop iron deficiency if they do not consume enough iron. Poor dietary habits can also lead to iron deficiency because these diets may be associated with insufficient iron intake.** Iron is easily absorbed in the duodenum, but if malabsorption occurs in the intestines, as seen in conditions like celiac disease, atrophic gastritis (where gastric acid is not sufficiently secreted), or after gastric and intestinal surgeries, the individual may gradually develop iron deficiency. **Clinical Symptoms of Iron Deficiency:** In the early stages of iron deficiency, due to compensatory mechanisms in our body, the body can compensate for this iron deficiency that leads to iron deficiency anemia, and the individual may not exhibit any symptoms. This initial stage can be identified through routine tests, allowing us to detect early stages of iron deficiency. However, when iron deficiency persists and leads to anemia, or when hemoglobin levels drop to 10 mg per deciliter or hematocrit levels fall to 30%, the individual will gradually begin to experience a series of symptoms similar to many other types of anemia, which can be non-specific. - The individual feels weak, experiences early fatigue following activity, has dizziness, and shows pallor. Given that iron is a key element in many of our enzymes, aside from symptoms of anemia, the individual may experience a range of new symptoms. Some of the fatigue may not be related to their anemia. The deficiency affecting their body enzymes, such as cytochrome enzymes, may cause them to experience early fatigue after activity. Thus, sometimes the degree of symptoms is not directly related to the level of anemia; an individual with hemoglobin levels we do not expect may experience much more severe symptoms. One rare symptom seen in individuals with iron deficiency anemia is dysphagia. This dysphagia is due to strictures and webs formed in the esophagus, known as Plummer-Vinson syndrome; however, this syndrome is very rare and can be considered a symptom of iron deficiency. The symptom known as pica can be specific and pathognomonic for iron deficiency. Pica refers to an abnormal craving for unusual food items, such as ice, dirt, or starch. The degree of this abnormal craving for food varies. For instance, in Iran, there is often a tendency to eat dirt or pieces of prayer beads. Thus, we only see pica symptoms in iron deficiency. **Physical Symptoms in Individuals with Iron Deficiency:** Certain symptoms are more commonly observed in iron deficiency, although some may also be present in other diseases. Symptoms include glossitis (inflammation of the tongue) and angular stomatitis (cracks at the corners of the mouth). Very rare manifestations such as koilonychia (spoon-shaped nails) or sclerae (pale sclera) can be seen in iron deficiency. In glossitis, the tongue appears red and loses its papillae. Glossitis, angular stomatitis, and spoon-shaped nails are observed in advanced stages of iron deficiency. **Diagnosis of Iron Deficiency:** One of the most basic tests for diagnosing anemia is the CBC test, where we can measure RBC count, hemoglobin, RDW, MCHC, MCH, MCV, and hematocrit. For example, if an individual presents with a hemoglobin level of 9.7, an MCV of 69.7, and an MCH of 22.6, all of which are below normal limits, this individual is diagnosed with microcytic anemia and hypochromic anemia. One of the actions we can take for this type is to prepare a peripheral blood smear to observe certain changes that can help us. In this anemia, the paleness of the RBC centers is much more pronounced. In hyperchromic microcytic anemia, besides the paleness of the RBC centers, we can observe that the size of the RBCs has decreased, with RBCs smaller than the nucleus of a lymphocyte, indicating they have become microcytic. Thus, in hypochromic microcytic anemia: the size of the RBCs has decreased, central paleness has increased (indicating they have become hypochromic), and the size of the RBCs varies significantly. In the central blood smear, in addition to observing the reduced size of the RBCs (microcytes) and the paleness of the centers (hypochromia), we see a significant variation in the size of the RBCs, indicating the RBCs have developed anisocytosis. One of the parameters reported by the Coulter counter is RDW, indicating a significant difference in the volume distribution of RBCs. This phenomenon can be observed in the early stages of hyperchromic microcytic anemia as an increase in CV-RDW. A definitive diagnosis of iron deficiency anemia is made by measuring a series of blood iron tests, known as the iron profile. Here’s the translation of the provided text: --- **The iron profile consists of three tests:** 1. Measurement of serum iron levels 2. Measurement of TIBC (Total Iron Binding Capacity) 3. Measurement of ferritin Serum iron refers to the iron present in the bloodstream, while TIBC is a direct measure of transferrin levels. After measuring serum iron and TIBC, if we divide the iron level by TIBC and multiply by 100, we obtain a value known as Transferrin Saturation (TS). In cases of iron deficiency, this TS falls below 20%. Ferritin is a very valuable test for individuals suspected of iron deficiency and indicates the body's iron reserves. In a stable individual with no inflammatory conditions, ferritin levels should be between 50- 100 ng/mL in adult males and 30-50 ng/mL in females. When an individual develops iron deficiency, ferritin drops below 50 ng/mL, and if it falls below 15 ng/mL, the likelihood of iron deficiency becomes very high. A significant problem with the ferritin test is that it is considered an acute phase reactant protein, meaning that when an individual is in an inflammatory state, ferritin may have little value for our diagnosis. One of the very important tests that helps evaluate an individual's body iron is measuring iron levels in a bone marrow sample. However, since bone marrow sampling is painful and invasive, this method is usually not employed. In situations where the individual is in an inflammatory disease state and we cannot estimate body iron levels based on blood tests, we may be compelled to perform this biopsy. Measuring the level of protoporphyrin in red blood cells can sometimes be very helpful. Protoporphyrin is an intermediary in the heme synthesis pathway, so any factor that disrupts heme synthesis can lead to increased levels of protoporphyrin in red blood cells. One of the very important causes of increased protoporphyrin levels in red blood cells can be iron deficiency or lead poisoning. **One valuable test is measuring transferrin receptors.** Sometimes, when our body is in an inflammatory condition, measuring ferritin is not useful, and tests like TIBC are also unhelpful. In such cases, measuring transferrin receptors can be very valuable for distinguishing between different causes of hypochromic microcytic anemia. When an individual has iron deficiency, the levels of these transferrin receptors can significantly increase in the blood, helping us differentiate between iron deficiency-related hypochromic microcytic anemia and hypochromic microcytic anemia due to inflammatory conditions. When a patient presents with anemia characterized by a decrease in red blood cell size (with MCV < 80) and a reduction in MCH, commonly referred to as hypochromic microcytic anemia, a series of differential diagnoses arise. The first differential diagnosis for this anemia is thalassemia. In individuals with thalassemia, MCV and MCH decrease, thus placing it in the differential diagnosis of hypochromic microcytic anemia. Here, assessing the iron status is very helpful in distinguishing between iron deficiency anemia and thalassemia-related anemia. The second differential diagnosis for hyperchromic microcytic anemia is anemia due to inflammatory diseases. Inflammatory diseases typically result in normocytic normochromic anemia, but over time, if an inflammatory disease becomes chronic, the individual may gradually develop hypochromic microcytic anemia. Here, it must be determined whether this anemia, which is characterized by reduced MCV and MCH, is due to iron deficiency or a chronic inflammatory disease. Unfortunately, in this situation, iron tests are not very helpful, and we may need to rely on bone marrow biopsy or measuring transferrin receptors in the blood for better assistance. Here’s the translation of the provided text: --- Another disease that is in the differential diagnosis of hypochromic microcytic anemia is myelodysplastic syndromes. This syndrome falls within the differential diagnosis of normochromic microcytic, normocytic anemias, and hypochromic microcytic anemias. In this context, measuring iron tests is also very helpful. Therefore, decreased MCV and MCH indicate several diagnoses: - Iron deficiency - Lead poisoning - Chronic inflammatory diseases - Thalassemia - Myelodysplastic syndromes.