Mutations and Cancer PDF
Document Details
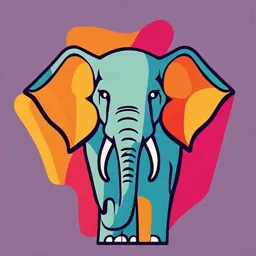
Uploaded by BestSellingAnemone
Tags
Summary
This document explains how DNA mutations can impact gene products. It covers large-scale and small-scale mutations, including substitutions, insertions, deletions, silent mutations, missense mutations, nonsense mutations, and frameshift mutations. It also discusses the consequences of these mutations in cellular processes.
Full Transcript
Mutations and Cancer Describe how DNA mutations can alter the gene product 1. Large-Scale vs. Small-Scale Mutations: ○ Large-scale mutations involve big rearrangements of DNA segments (think of them as genetic remodelling projects). ○ But today, we’re focusing on small-scale mutations, which involve...
Mutations and Cancer Describe how DNA mutations can alter the gene product 1. Large-Scale vs. Small-Scale Mutations: ○ Large-scale mutations involve big rearrangements of DNA segments (think of them as genetic remodelling projects). ○ But today, we’re focusing on small-scale mutations, which involve changes in just a few nucleotide pairs. 2. Two Types of Small-Scale Mutations: ○ Substitutions: Imagine swapping a single letter in a word. That’s what a substitution does in our genetic code. i. 3. 4. 5. 6. 7. For example, changing 3′@CCG@5′ to 3′@CCA@5′. The mRNA codon used to be GGC, but now it’s GGU. Still, both code for the same amino acid (glycine). ii. Some substitutions have no effect due to the redundancy of the genetic code. ○ Insertions and Deletions: These involve adding or removing nucleotide pairs. It’s like editing a sentence by adding or deleting words. Silent Mutations: type of substitution ○ These are like secret agents—they don’t change the protein’s appearance. ○ A change in a nucleotide pair may transform one codon into another that still codes for the same amino acid. ○ Silent mutations can occur outside genes too. Missense Mutations: type of substitution ○ Picture a protein with a new amino acid—it’s like a surprise guest at a party. ○ Sometimes, this change has little effect because the new amino acid behaves similarly to the old one. ○ In other cases, it might be in a region where the exact sequence isn’t crucial for the protein’s function. Nonsense Mutations: ○ Picture a stop sign in the middle of a sentence—it abruptly ends the translation process. ○ A nonsense mutation changes a codon for an amino acid into a stop codon. ○ Translation terminates prematurely, resulting in a shorter, often nonfunctional protein. Insertions: ○ An insertion mutation occurs when extra base pairs are added into a gene’s DNA sequence. ○ Imagine it as inserting an extra word into a sentence. This alteration can happen at any position within the gene. ○ The impact of insertions can be significant. They can lead to changes in the resulting protein, affecting its function. ○ One crucial consequence of insertions is the potential for a frameshift mutation. Deletions: A deletion mutation involves the loss of a section of DNA from the gene. Think of it as removing a word from a sentence. Like insertions, deletions can occur at various points in the gene. ○ Deletions can have serious effects, especially if they disrupt the reading frame of the genetic message. ○ Frameshift mutations often result from deletions. 8. Frameshift Mutations: ○ Protein-coding DNA is divided into codons, each consisting of three bases. ○ When an insertion or deletion alters the number of nucleotides in a gene, it can shift the reading frame. ○ This means that the codons are no longer correctly parsed during translation. ○ The result? Truncated proteins—as useless as a jumbled sentence like “hef atc ats at.” ○ ○ Consequences: ○ ○ ○ ○ All nucleotides downstream of the insertion or deletion are grouped into incorrect codons. This leads to extensive missense mutations, where amino acids are substituted in the protein. In severe cases, a premature termination occurs, known as a nonsense mutation. Unless the frameshift occurs near the gene’s end, the resulting protein is likely to be nonfunctional. Beyond Coding Regions: ○ ○ Insertions and deletions can also impact gene expression and phenotype outside of coding regions. While not always classified as frameshift mutations, these changes still play a significant role in shaping how genes function. An example - sickle cell anaemia 1. Sickle-Cell Disease: ○ Sickle-cell disease is a genetic disorder caused by a specific point mutation in the DNA. ○ This mutation affects the hemoglobin protein, which is responsible for carrying oxygen in red blood cells. ○ Individuals with sickle-cell disease have abnormal hemoglobin, leading to the characteristic sickling of red blood cells. 2. The Point Mutation: ○ The mutation occurs in a single DNA nucleotide pair within the beta-globin gene. ○ Specifically, the adenine base is replaced by thymine at a specific position. ○ This tiny change alters the amino acid sequence in the hemoglobin protein. 3. Consequences: ○ The altered hemoglobin forms long, rigid fibers when oxygen levels are low. ○ These fibers cause red blood cells to become sickle-shaped, rather than their usual flexible, biconcave shape. ○ Sickle cells are less efficient at carrying oxygen and can block blood vessels, leading to pain, tissue damage, and other complications. Recall the role of cell cycle checkpoints in mitosis Looking at G2 Maturation-Promoting Factor (MPF): ○ MPF is a cyclin-Cdk complex that plays a crucial role in controlling the cell cycle. ○ It was first discovered in frog eggs, where it stimulates both mitosis (cell division) and meiosis (cell division for sexual reproduction). ○ MPF acts as a signal to transition from the G2 phase to the M phase (mitosis). ○ How does it work? By phosphorylating various proteins needed during mitosis. Structure of MPF: ○ MPF consists of two subunits: Cyclin-Dependent Kinase 1 (CDK1): This is the kinase subunit. It uses ATP to add phosphate groups to specific serine and threonine residues on target proteins. Cyclin: The regulatory subunit. Cyclins are essential for CDK1 to function properly with the right substrates. The mitotic cyclins fall into groups A and B. Interestingly, cyclins have a specific sequence called the “destruction box”. When recognized by the ubiquitin ligase enzyme, it leads to their destruction when no longer needed. Role in the Cell Cycle: ○ During the G1 and S phases, the CDK1 subunit of MPF remains inactive due to an inhibitory enzyme called Wee1. ○ Wee1 phosphorylates a specific tyrosine residue (Tyr-15) on CDK1, rendering MPF inactive. ○ As the cell transitions from G2 to M phase, CDC25 de-phosphorylates CDK1. ○ Now, the CDK1 subunit can bind to cyclin B, activating MPF. ○ The result? The cell enters mitosis. Significance: ○ MPF is often called the “M phase kinase” because it precisely phosphorylates target proteins at a specific point in the cell cycle. ○ By doing so, it controls their ability to function during mitosis. Describe how mutations in tumour supressor genes or proto oncogenes can impact cell cycle control Cell Cycle Checkpoints: ○ Cell cycle checkpoints act as quality control stations during the cell cycle. ○ They ensure that critical events (such as DNA replication and chromosome segregation) occur accurately before the cell proceeds to the next phase. ○ These checkpoints rely on stop and go signals provided by various gene products. STOP Molecules: ○ Some gene products act as brakes, preventing excessive cell proliferation: Tumor Suppressor Genes: These genes normally keep cell growth in check. When mutated, they lose their inhibitory function, potentially leading to uncontrolled cell division. Examples include p53, which monitors DNA damage, and BRCA1/2, associated with breast and ovarian cancers. GO Molecules: ○ Other gene products act as accelerators, stimulating cell proliferation: Proto-Oncogenes: These genes promote cell growth and division. When mutated or overexpressed, they become oncogenes, driving cancer development. Examples include Ras, which regulates cell signaling, and MYC, involved in cell cycle progression. Maturation-Promoting Factor (MPF): ○ MPF is a critical player during the cell cycle. ○ It consists of two components: Cyclin B: The regulatory subunit. Cdk1 (Cdc2): The kinase subunit. ○ When cyclin B binds to Cdk1, it forms MPF. ○ MPF’s activation triggers the transition from G2 phase to M phase (mitosis). Cdc2/Cdk1/P34: ○ These terms all refer to the same protein: Cdc2: Cell division cycle 2. Cdk1: Cyclin-dependent kinase 1. P34: Another name for Cdk1. ○ Cdc2/Cdk1 is a key regulator of the cell cycle, controlling entry into mitosis. Appreciate that loss of cell cycle control can result in cancer 1. Proto-Oncogenes: ○ Proto-oncogenes are like the gas pedals of cell growth and division. ○ Normally, they help cells: Grow and divide to create new cells. Survive by preventing cell death (apoptosis). ○ However, when a proto-oncogene mutates or becomes overactive, it transforms into an oncogene. ○ Oncogenes are like gas pedals stuck down—causing cells to divide out of control. ○ How do proto-oncogenes become oncogenes? Gene Variants/Mutations: Some people inherit gene differences that keep the gas pedal on all the time. Epigenetic Changes: Chemical modifications can turn on proto-oncogenes without altering the gene sequence. Chromosome Rearrangements: DNA sequence changes during cell division can activate proto-oncogenes. Tumour Suppressor Genes: Tumor suppressor genes act as the brakes in cell growth. Their normal function is to: ○ Inhibit cell division. ○ Detect and repair DNA damage. ○ Promote apoptosis if necessary. When tumor suppressor genes are mutated or inactivated, the brakes fail, allowing uncontrolled cell growth. Examples include p53, which monitors DNA integrity, and BRCA1/2, linked to breast and ovarian cancers Analogy: The Car: ○ Imagine a cell as a car: Proto-oncogenes are the accelerator (gas pedal), pushing cell growth. Tumor suppressor genes are the brakes, ensuring controlled division. Cancer occurs when the balance between these two is disrupted.