Muscle Diseases Multiple PDF
Document Details
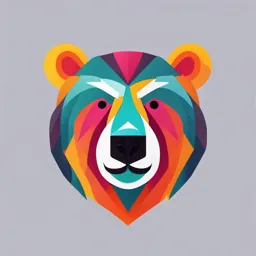
Uploaded by WellBacklitAppleTree
Tags
Summary
This document discusses the pathology of organ systems, particularly focusing on muscle diseases and related conditions in domestic animals. It describes various diseases affecting bones, joints, tendons, and ligaments, along with their causes and impacts. The text includes detailed figures and diagrams supporting the explanations.
Full Transcript
1056 SECTION II Pathology of Organ Systems and resorption, resulting in varying degrees of reactive bone formation and bone lysis. The exudate associated with some acute infections in the medullary cavity can increase the pressure in this region and cause compression of the nutrient artery, resultin...
1056 SECTION II Pathology of Organ Systems and resorption, resulting in varying degrees of reactive bone formation and bone lysis. The exudate associated with some acute infections in the medullary cavity can increase the pressure in this region and cause compression of the nutrient artery, resulting in ischemic necrosis. If resorbed cortical bone is replaced by fibrous repair tissue, the bone can become unstable. For more detailed information on inflammation, infectious agents, and immune defense mechanisms, see Chapter 3, Inflammation and Healing; Chapter 4, Mechanisms of Microbial Infections; and Chapter 5, Diseases of Immunity, respectively. Joints * The cellular and humoral defenses against infectious agents are no different in the joint from those in other tissues. However, because articular cartilage has such limited ability to regenerate, progression to DJD could occur after inflammation that either destroys the ability of synovium to provide nutrients and synovial fluid to the cartilage or destroys areas of the cartilage. For more detailed information on inflammation, infectious agents, and immune defense mechanisms, see Chapter 3, Inflammation and Healing; Chapter 4, Mechanisms of Microbial Infections; and Chapter 5, Diseases of Immunity, respectively. p A Tendons/Ligaments n The cellular and humoral defenses against infectious agents are no different in tendons and ligaments from those in other tissues. For more detailed information on inflammation, infectious agents, and immune defense mechanisms, see Chapter 3, Inflammation and Healing; Chapter 4, Mechanisms of Microbial Infections; and Chapter 5, Diseases of Immunity, respectively. Diseases Affecting Multiple Species of Domestic Animalsf Bone B Figure 16.34 Embolic (Suppurative) Osteomyelitis, Bone, Horse. A, Distal tibial physis. Localized area of infection and bone lysis (asterisk) within physis and proximal metaphysis. p, Physis. B, Talus. Suppurative osteomyelitis has extended from its site of origin in the cancellous bone into the articular-epiphyseal cartilage complex (AECC) via cartilage canal vessels (arrows). The affected bone contains multiple cavities, is separated by a cleft (arrowhead) from the overlying cartilage, and is pale and opaque (necrosis [n]). (Courtesy Dr. A. Wuenschmann, College of Veterinary Medicine, University of Minnesota.) Tendons/Ligaments Infection of tendons and ligaments usually requires a sharp force injury or puncture wound because of the dense connective tissue covering of the tendon (epitenon) or ligament (epiligament). Less commonly, there may be extension of infection from infected skin or from an adjacent joint. The sequelae of bacterial infection of tendons and ligaments most often include adhesions and accompanying loss of function. Defense Mechanisms/Barrier Systems Bone The defense against infectious agents is no different in bone than in other tissues. However, the consequences of an inflammatory response can greatly affect the structure and function of bone. Many soluble inflammatory mediators can increase both bone formation Abnormalities of Growth and Development There are many potential, suspected, or known genetic disorders (see E-Table 1.2) of bone. Improved technology and extensive research in the field has led to advancements in our knowledge of the genes underlying musculoskeletal diseases. Given the rapidity of progress in the field of genetics, detailing every known genetic variant associated with diseases of the musculoskeletal system in veterinary species is beyond the scope of this text. Many diseases may also vary in causative genetic mutation or mode of inheritance between species or even between breeds within a species. For example, cranial cruciate ligament (CCL) disease, a common skeletal disease in the dog, seems to be a polygenic (complex) trait, with occurrence related to several genetic and environmental factors. In one study, three single nucleotide polymorphisms (SNPs) were significantly associated with the risk of CCL disease in Newfoundland dogs, while 13 significant SNPs were identified to best predict risk for CCL disease in Labrador retrievers. A different study found over 100 SNPs within 99 risk loci associated with CCL disease in Labrador retrievers. If necropsy findings are consistent with a possible genetic disorder, samples of a cell-rich tissue (liver, spleen) may be collected unfixed with sterile technique and frozen to preserve genomic DNA until identification of an appropriate laboratory for further assessment. The process of genome assessment can be lengthy (months to fExamples of known or suspected genetic disorders are listed in E-Table 1.2. 1057 CHAPTER 16 Bones, Joints, Tendons, and Ligaments Articular cartilage Cartilage canal blood vessels Pus in joint Pus in cartilage canal blood vessels Epiphyseal cartilage Hematopoietic marrow Growth plate cartilage Pus Microabscess Cortex (lamellar bone) Hematopoietic marrow Vascular loop Osteoblast Osteoclast Fibrosis A Periosteum Reactive woven bone Microabscess (pus) B Draining sinus through skin Periosteum C D Figure 16.35 Patterns of Spread of Embolic Osteomyelitis from the Physis. Capillary loops in the metaphyseal side of the physis serve as a predilection site for septic emboli to lodge (A). Lysis of metaphyseal bone and growth plate cartilage secondary to inflammation may cause mechanical instability, to which the periosteum responds by producing reactive bone (B). Lysis of the cortex at its thinnest point (the metaphyseal cut back zone) may lead to extension of inflammation/pus into the periosteum (periostitis), perichondrium and cartilage canal vessels, or into the joint (arthritis) (C and D). 4 5 3 2 Figure 16.36 Embolic Bacterial Physitis, Osteomyelitis, Periostitis, Proximal First Phalanx and Arthritis, Metacarpal-Phalangeal Joint, Horse. Bacterial inflammation has destroyed the physis (arrow) and has extended into the periosteum and joint cavity (left [arrowhead]). (Courtesy Dr. S.E. Weisbrode, College of Veterinary Medicine, The Ohio State University.) years), is not guaranteed to be successful (because of the exploratory nature of the investigation), and may not be feasible in the vast majority of routine diagnostic cases. Disorders of Bone Resorption Osteopetrosis. Osteopetrosis is a heterogeneous group of conditions that is characterized by an increase in bone density resulting from a failure in bone resorption by osteoclasts. Although bone mineral density is increased, the bones usually are more fragile than normal. Pathologic fractures (abnormal bone that fractures with minimal trauma; see further discussion in the section on Fracture Repair) are reported in animals that survive birth; however, most affected animals are stillborn. Although trabecular bone accumulates in epiphyses, metaphyses, and diaphyses, there is a reduction in cortical bone quantity and quality 1 Figure 16.37 Routes of Infection for a Joint in an Adult. 1, The hematogenous route. 2, Extension from osteomyelitis. 3, Spread from an adjacent soft tissue infection. 4, Iatrogenic (diagnostic or therapeutic procedures). 5, Penetrating damage from puncture wound or incision (e.g., during surgery). because of a failure of bone modeling at this site, which likely is the explanation for the fractures that are seen in these animals. The disease occurs in human beings and in multiple animal species, including dogs, cats, sheep, horses (Peruvian Paso breed), cattle (Angus, Herefords, and Belgian Blue), rats, and several strains of mice. Osteopetrosis can be caused by mutations that impair the generation or the function of osteoclasts. Although most forms are inherited, some are associated with infectious agents (in utero bovine viral diarrhea virus [BVDV] or feline leukemia virus [FeLV] infections; avian leukosis virus), and these are termed “infectious forms of osteopetrosis.” In severe forms of osteopetrosis, the insufficient bone marrow cavity is unable to support hematopoiesis, resulting in thrombocytopenia, anemia, and susceptibility to infections. Neurologic complications (including blindness) may occur secondary to bony compression of the brainstem and/or cranial nerves. Other features of the disease may include brachygnathia inferior, impacted molar teeth, and deformed cranial vaults. 1058 SECTION II Pathology of Organ Systems B C A D Figure 16.38 Osteopetrosis, Bone, Foal (Peruvian Paso). A, Longitudinal section of humerus. The primary trabeculae are retained and fill the entire medullary cavity, forming a triangular shape. Although the overall amount of bone is increased, the cortices are thin (arrows). B, Radiographs of multiple long bones exhibiting the triangular-shaped radiodensities within the metaphyses and diaphysis as demonstrated grossly in A. C, Histologic section showing the transition (arrowheads) from trabeculated cortical bone (upper half of image) to retained small bony trabeculae containing numerous cartilaginous cores (lower half of image). Hematoxylin and eosin (H&E) stain. D, Osteoclasts (arrows) increased in number but are ineffective in resorbing retained bone. The pale basophilic areas of matrix represent retained cartilaginous cores. H&E stain. (Courtesy Dr. C.S. Carlson, Dr. E.J. Olson, and Dr. N.A. Robinson, College of Veterinary Medicine, University of Minnesota.) Because osteoclasts arise from hematopoietic precursor cells, bone marrow transplantation (in which normal donor marrow components are injected) is curative for some types of osteopetrosis in human beings as a result of repopulation by normal osteoclast precursors. Growth plate: There are no primary lesions in the growth plate in osteopetrosis. Trabecular bone: Although osteoclasts may be numerous, these cells are unable to resorb and shape (model) the primary trabeculae (Fig. 16.38; E-Fig. 16.16). As a result, spicules of bone with central cores of calcified cartilage fill the medullary cavity. Affected bones are dense and have little or no medullary cavity. Cortical bone: Cortical bone lesions in osteopetrosis are not reported consistently but can range from the cortex being too thin to it being inadequately compacted in animals that have plexiform (laminar) cortical bone growth (e.g., rapidly growing large animals such as sheep and cattle). CHAPTER 16 Bones, Joints, Tendons, and Ligaments 1058.e1 * P * A B C E-Figure 16.16 Osteopetrosis, Bone, Femur, Calf (Aberdeen Angus). A, The primary trabeculae are retained and fill the entire medullary cavity. B, Low magnification of boxed area in A. P, Physis. C, Higher magnification of B. Retained unremodeled trabeculae containing cartilaginous cores (asterisks) fill the medullary cavity. Hematoxylin and eosin (H&E) stain. (A courtesy Dr. H. Leipold, College of Veterinary Medicine, Kansas State University. B and C courtesy Dr. S.E. Weisbrode, College of Veterinary Medicine, The Ohio State University.) CHAPTER 16 Bones, Joints, Tendons, and Ligaments Disorders of Bone Formation Osteogenesis Imperfecta. Osteogenesis imperfecta (OI) is a heterogeneous group of heritable connective tissue disorders that are characterized clinically in their most severe form by bone fractures (resulting from osteopenia, bone density that is lower than normal peak density), joint laxity (defective composition of tendons), dental abnormalities including fractured teeth (defective dentin), and blue sclerae (because of reduced thickness of this tissue). It is the most common heritable connective tissue disorder in human beings and has been described in the veterinary literature in multiple species, including calves, lambs, kittens, puppies, and mice. Many cases of OI (estimated 90% of cases in human and veterinary medicine) are associated with mutations in COL1A1 or COL1A2, which are genes that encode the pro-α1 or pro-α2 chains of type 1 procollagen. For example, the 1A1 mutation has been described in golden retrievers and the 1A2 mutation has been described in beagles. Genes coding for enzymes responsible for posttranslational modification of collagen and genes for certain trimeric intracellular cation (TRIC) channels important in osteoblast function are mutated less commonly. Mild forms of the disease, caused by inactivation of an allele of one of the genes, result in reduced amounts of normal collagen. Conversely, mutations causing substitutions in critical amino acids necessary for collagen helix formation and cross-linking can result in production of normal amounts of structurally inferior collagen. Interestingly, the clinical manifestations of these mutations are usually limited to the bone, teeth, and eyes, even though type I collagen is also the major structural collagen in skin. Exceptions include some forms of Ehlers-Danlos syndrome that are associated with COL1A1 mutations and have skin changes. A mutation in the SERPINH1 gene has been reported in OI in dachshund dogs. SERPINH1, also known as heat shock protein 47 (Hsp47), is an endoplasmic reticulum resident pro-collagen–specific molecular chaperone that is essential for the proper assembly of the triple helical procollagen molecules, which eventually are transported across the Golgi apparatus to the extracellular space. Growth plate: Growth plates are not affected in osteogenesis imperfecta (OI) (primary collagen of hyaline cartilage is type II collagen). Trabecular bone: In severe cases, there is greatly reduced trabecular bone without evidence of marked osteoclasis (breaking down or absorption of osseous tissue) or proliferation of fibrous tissue, as would be expected in severe cases of fibrous osteodystrophy (FOD) (see later discussion). Osteoblasts may appear normal or small. In some cases, the amount of bone and its microscopic appearance are normal, but there is evidence of fractures. These fractures may occur in utero and are recognized at birth by callus formation. Bone fragility in cases in which bone morphology and mass are normal is likely to be caused by errors in helix formation or cross-linking of tropocollagen molecules. Affected individuals may become osteopenic with age, possibly as a result of decreased mechanical use because of bone pain from fractures. Cortical bone: There is a delay in the compaction of cortical bone. During normal development, species-dependent spaces of varying size and contour remain between trabeculae of woven bone in the developing cortex. The filling of these spaces by bone to solidify the cortex is called compaction. The cortices in osteogenesis imperfecta may be composed of woven bone containing large vascular spaces that remain empty. If the animal survives, these vascular spaces may eventually fill in with bone to form compact cortical bone. Teeth: Teeth may be structurally normal other than the presence of fractures or may be pink because of visibility of the dental pulp through the thin crown. Dental fractures also may be present. Histologically, the dentin tubules are short, tortuous, and sometimes 1059 absent. The disorganization of the dentin is a qualitative change that allows confirmation of the diagnosis without requiring an agematched control animal. Disorders of Endochondral Ossification Chondrodysplasias. Chondrodysplasias are hereditary dis- orders of bone growth that occur as a result of primary lesions in growth cartilage. Growth cartilage is present in the physis (responsible for longitudinal bone growth) and the epiphyseal cartilage of the AECC (growth cartilage at the ends of growing long bones; responsible for development of epiphysis), both of which are replaced by bone in the adult individual. Chondrodysplasias can result in disproportionate dwarfism. Affected animals usually are short-legged with normal-sized heads because the bones of the calvaria (but not the maxilla and mandible) arise from intramembranous, rather than by endochondral, ossification. Primordial dwarfism, in which the limbs are proportional to body length, is not classified as a chondrodysplasia and may occur secondary to underlying endocrine disease (pituitary dwarfism) or malnutrition but also may be genetically determined (e.g., by selective pressure to reduce the size of popular dog breeds). Both types of dwarfism are represented by distinct breeds. For example, dachshund, Pekingese, and basset hounds are examples of chondrodysplastic dwarfs, and miniature poodles, miniature schnauzers, and miniature pinschers are examples of primordial dwarfs. The mechanisms of chondrodysplasias in animals are becoming better understood through genetic studies and often involve inherited errors in genes that control chondrogenesis. In human beings, the most common dwarfism is called achondroplasia (a misnomer because growth cartilage is present). The condition is inherited as an autosomal dominant trait caused by a single point mutation in the fibroblast growth factor receptor 3 (FGFR3) gene, which is a negative regulator of bone growth. This mutation results in constant activation of this receptor, causing downregulation of chondrocyte proliferation. Conversely, spider lamb chondrodysplasia, which occurs in Suffolk and Hampshire sheep, is the result of a single base change in the tyrosine kinase II domain of FGFR3, which removes the FGFR3-induced inhibition of chondrocyte proliferation and results in elongation of the limbs and the presence of multiple secondary centers of ossification in the epiphyses (Fig. 16.39; also see E-Fig. 18.2, A). This unusual type of chondrodysplasia also has been reported in mice. Recently, the genetic mutation for the chondrodysplasia typifying the commonly recognized chondrodysplastic canine breeds, including dachshunds, Pekingese, and basset hounds, has been identified as a conserved fibroblast growth factor 4 (FGF4) retrogene on canine chromosome 18 that likely arose before the division of early dogs into modern breeds. Atypical expression of the FGF4 transcript in chondrocytes may cause inappropriate activation of one or more of the FGF receptors, such as FGFR3. Skeletal dysplasias have also been reported in animals with inherited lysosomal storage diseases such as mucopolysaccharidosis and gangliosidosis. In these individuals, chondrocytes may be vacuolated because of cytoplasmic retention of glycosaminoglycans and lipid. It is likely that chondrocyte function is altered, adversely affecting the process of endochondral ossification, as epiphyseal cartilage and cartilage canals in the AECC are reported to be retained in at least one feline model of gangliosidosis, and the shape of the epiphyses in these animals is markedly altered. Growth plate: Growth plate widths can be normal or reduced depending on the specific disease. Likewise, chondrocytes can be arranged in columns or markedly disorganized. The cartilage matrix also may have a normal appearance or may be rarefied (less compact or dense, characterized by reduced staining intensity). 1060 SECTION II Pathology of Organ Systems * Figure 16.39 Spider Lamb Chondrodysplasia, Thoracic Spine, Longitudinal Section, Suffolk Lamb. The vertebral column has multiple, disorganized ossification centers resulting in variation of the size, shape, and orientation of the vertebrae. (Courtesy Dr. C.S. Carlson, College of Veterinary Medicine, University of Minnesota.) Trabecular bone: Trabeculae in the primary and secondary spongiosa may be thickened and irregular and may exhibit lateral bridging between trabeculae. Cartilage cores may be larger than normal and may be retained in the secondary spongiosa. These changes may vary greatly, depending on the type of chondrodysplasia. Cortical bone: Lesions in cortical bone are variable and are reflected primarily by grossly apparent abnormalities in shape or size. Histologically, this tissue usually has a normal appearance. Osteochondrosis Latens and Manifesta. The osteochondroses consist of a heterogeneous group of lesions involving the growth cartilage of young animals and are characterized by focal or multifocal failure (or delay) of endochondral ossification. The sites where these lesions occur are the metaphyseal growth plate and the epiphyseal cartilage of the AECC. The lesions are common, often occur with bilateral symmetry, and represent an important orthopedic entity that has a number of different clinical manifestations in pigs, dogs, horses, cattle, and poultry. The hallmark of the uncomplicated gross lesions of osteochondrosis is focal retention of growth cartilage because of its failure to become calcified and replaced by bone (a failure of endochondral ossification). In the growth plate, this is the result of an accumulation of viable hypertrophic chondrocytes, whereas in the AECC it results from an area of necrosis of epiphyseal cartilage. The etiology of osteochondrosis appears to be multifactorial, with genetics, rapid growth rate, vascular factors, and trauma all being implicated. Trauma is the most widely proposed etiology in both human beings and animals; however, its most likely role is as a final insult to compromised epiphyseal cartilage rather than as an initiating factor in the development of early lesions. Rapid growth and nutritional factors also are cited. Although osteochondrosis occurs during the period of rapid growth and is most common in species that grow rapidly, most experimental work has failed to document rapid growth as a cause of the disease. In addition, little evidence is available to indicate that the lesions of osteochondrosis result from a specific nutritional deficiency. Copper deficiency, perhaps induced by excess dietary zinc, produces lysis of the AECC and formation of thin flaps of cartilage in thoroughbred suckling foals; however, the distribution of these lesions and their extent and severity distinguish them from the multifocal failure of endochondral ossification that is the hallmark of osteochondrosis. Experimental work in pigs and horses has demonstrated that a defect (unknown cause) in vascular supply to growth cartilage, resulting in localized areas of ischemic necrosis, is important in the Figure 16.40 MRI Image Using Susceptibility Weighted Imaging, Distal Femur, Coronal Section, Immature Pig. Avascular articular cartilage of femoral trochlea (arrow); cartilage canal vessels indicated by black lines; secondary center of ossification (asterisk); physis (arrowheads). (Courtesy Dr. C.S. Carlson and Dr. F. Tóth, College of Veterinary Medicine, University of Minnesota.) initiation of subclinical lesions. Although articular cartilage is avascular throughout life, subarticular epiphyseal cartilage of the AECC and growth plate cartilage both are highly vascularized tissues and depend on a vascular supply for viability (Fig. 16.40; E-Fig. 16.17). The blood vessels supplying growth cartilage run in channels that are termed cartilage canals. Both growth plate and epiphyseal (growth) cartilage are temporary, by definition. As endochondral ossification proceeds during growth, the epiphyseal cartilage gradually becomes reduced in volume and requires the nutritional support of fewer vascular channels, until it becomes entirely avascular and eventually is completely replaced by bone. It is during the time frame in which the epiphyseal cartilage is supplied by blood vessels that subclinical lesions of osteochondrosis occur. This time frame varies, based on site, age, and species, which likely explains, along with biomechanical factors, why different species have different predilection sites for the development of osteochondrosis. Detailed studies in pigs and horses have revealed that subclinical lesions occur within the first few weeks of life in some predilection sites. The finding of extensive areas of necrosis of epiphyseal cartilage in 2-week-old foals suggests that the underlying lesion may, in some cases, even occur in utero or soon after birth. Although the nature of the insult to the vessel(s) is unclear, the site of the injury appears to be localized to the chondroosseous junction, and the timing of its occurrence corresponds with the formation of vascular anastomoses between vessels in epiphyseal cartilage and vessels in the subchondral bone as the ossification front reaches the vessels that are present in the epiphyseal cartilage. The result is a well-demarcated area of necrosis of epiphyseal cartilage that is centered on necrotic blood vessels, visible only microscopically, and termed osteochondrosis latens (Fig. 16.41). When the ossification front of the secondary center of ossification reaches the area of necrosis, failure of endochondral ossification occurs, resulting in an area of retained necrotic epiphyseal cartilage that is visible grossly and radiographically. The lesion at this stage is termed osteochondrosis manifesta (Fig. 16.42; E-Fig. 16.18). This lesion is highly vulnerable to traumatic clefting through the area of necrosis and extending into the overlying articular cartilage, at which point it is termed osteochondrosis dissecans (Fig. 16.43; E-Fig. 16.19). It may also result from normal physiologic pressure. Once clefting through the articular cartilage occurs and subchondral bone is exposed, the lesion is highly painful and results in clinical signs of lameness. In CHAPTER 16 Bones, Joints, Tendons, and Ligaments 1060.e1 E-Figure 16.17 Vascular Supply to Cranial Aspect of Distal Intermediate Ridge, Tibia, 14-Day-Old Standardbred Foal. The arteries have been perfused with micronized barium and the tissues cleared by the modified Spälteholz technique. The cartilage has become translucent, and the subchondral bone is reddish-brown. The image shows a perfused blood vessel (arrow) coursing distally on the cranial aspect of the tibia before turning, branching, and continuing within the cartilage on the articular aspect of the distal tibia. (Courtesy Dr. K. Olstad, Norwegian School of Veterinary Medicine, Oslo, Norway.) c A B E-Figure 16.18 Osteochondrosis Manifesta, Bone, Distal Femur, Lateral Trochlear Ridge, Horse. A, The cartilage (c) extending into the epiphysis is composed of necrotic epiphyseal cartilage that has failed to undergo endochondral ossification and has been retained, whereas the remainder of the epiphyseal cartilage has been converted to bone. The retained cartilage has a yellowish appearance compared with the overlying articular cartilage resulting from degeneration/necrosis of the retained cartilage. B, Histologically, there is evidence of a marked cartilage repair process that is characterized by chondrocyte proliferation. The chondro-osseous junction (arrowheads) contains a thin margin of acellular, eosinophilic matrix that is associated with several small clefts and is interpreted as necrotic cartilage; eosinophilic streaks (arrows) within the retained cartilage may represent collapsed, necrotic vascular channels. Hematoxylin and eosin (H&E) stain. (Courtesy Dr. S.E. Weisbrode, College of Veterinary Medicine, The Ohio State University.) 1060.e2 SECTION II Pathology of Organ Systems c E-Figure 16.19 Osteochondrosis Dissecans, Bone, Articular Cartilage, Humerus, Proximal End, Dog. A flap of thickened cartilage (c) has detached from the underlying bone. The synovium is hyperemic and hyperplastic. (Courtesy Dr. S.E. Weisbrode, College of Veterinary Medicine, The Ohio State University.) CHAPTER 16 Bones, Joints, Tendons, and Ligaments B A 1061 C Figure 16.41 Osteochondrosis Latens, Lateral Femoral Condyle, 12-Week-Old Pig. A, Locally extensive area of chondronecrosis (within ellipses) within the epiphyseal cartilage of the articular-epiphyseal cartilage complex (AECC), centered on necrotic cartilage canal blood vessels (arrows). Neither the overlying articular cartilage nor the subjacent subchondral bone is involved at this stage of the disease. Hematoxylin and eosin (H&E) stain. B, Higher magnification of A showing necrotic cartilage canal blood vessels (arrows) and necrotic chondrocytes (arrowheads). C, Low-magnification image of osteochondrosis latens lesion (arrowheads) confined to epiphyseal cartilage and composed of necrotic cartilage. H&E stain. (Courtesy Dr. C.S. Carlson, College of Veterinary Medicine, University of Minnesota.) * B A C D Figure 16.42 Osteochondrosis Manifesta, Bone, Distal Femur, Immature Pig. A, Femoral condyles (with medial femoral condyle on the left [asterisk]) showing no articular cartilage surface abnormalities. B, Coronal slab section through medial femoral condyle showing intact articular surface and areas of retained cartilage (arrows) typical of locally extensive delay of endochondral ossification (osteochondrosis manifesta). C, Faxitron radiograph of 3-mm–thick coronal slab section showing focal area of radiolucency typical of osteochondrosis manifesta lesion involving the medial femoral condyle (same animal/anatomic site as in A and B). D, Locally extensive area of chondronecrosis in epiphyseal cartilage resulting in delayed endochondral ossification (osteochondrosis manifesta); upper limit of lesion demarcated by arrowheads. Hematoxylin and eosin (H&E) stain. (Courtesy Dr. C.S. Carlson and Dr. E.J. Olson, College of Veterinary Medicine, University of Minnesota.) 1062 SECTION II Pathology of Organ Systems t Figure 16.43 Osteochondrosis Dissecans, Bone, Articular Cartilage, Femur, Distal End, Pig. A chondro-osseous fragment (arrowhead) has detached from the underlying bone (arrow) of the medial femoral condyle and is displaced to the intercondylar area within the joint; long digital extensor tendon (t). (Courtesy Dr. C.S. Carlson and Dr. E.J. Olson, College of Veterinary Medicine, University of Minnesota.) the majority of cases, however, cleft formation does not occur, and the subclinical lesions heal. In these cases, the necrotic epiphyseal cartilage becomes surrounded by the advancing ossification front, as adjacent viable epiphyseal cartilage undergoes endochondral ossification. Over time, the area of necrotic cartilage gradually diminishes in size and eventually becomes completely replaced by bone. In horses, particularly in the medial femoral condyle (weight-bearing location), evidence strongly supports the theory that some of these early lesions of osteochondrosis develop into subchondral bone cysts. Physeal lesions of osteochondrosis also are likely to have a vascular etiology but are characterized by multifocal areas of retained, viable, hypertrophic chondrocytes. If these involve an extensive area of the growth plate, the growth plate may fracture, either partially or completely, resulting in a variety of clinical sequelae, including angular limb deformities. Growth plate and AECC: Lesions of osteochondrosis are not recognized grossly until they result in a focal failure of endochondral ossification, at which time they are composed of a well-demarcated wedge of retained cartilage involving the epiphyseal cartilage at the AECC (osteochondrosis manifesta) or physis (physeal osteochondrosis). Often there is considerable reaction of the underlying marrow spaces, including increased numbers of osteoblasts, osteoclasts, and fibroblasts. Predilection sites for both clinical and subclinical lesions include the AECC of the distal femur (especially the medial femoral condyle) and humerus (condyles and head) of pigs; the distal femur (medial condyle and both trochlear ridges), distal tibia (cranial intermediate ridge and medial malleolus), talus (trochlear ridges), and articular processes of the cervical vertebrae of horses; the humerus (humeral head and medial humeral condyle), distal femur (both condyles), and talus (medial and lateral trochlear ridges) of dogs; the talus (medial and lateral trochlear ridges) and distal femur (both trochlear ridges) of cattle; and the proximal tibia of rapidly growing birds. Osteochondrosis latens lesions are only recognized histologically in the AECC and occur as well-demarcated areas of necrosis of epiphyseal cartilage, usually adjacent to or surrounding one or more necrotic cartilage canal blood vessels (see Fig. 16.41). The overlying articular cartilage and subjacent subchondral bone are not affected at this stage of the disease. Osteochondrosis manifesta lesions also are composed of necrotic epiphyseal cartilage, but at this point of the disease, the ossification front has reached and partially surrounded the area of necrosis so that it is grossly visible (see Fig. 16.42). Lesions of physeal osteochondrosis, in contrast to those occurring in the AECC, are composed of columns of retained, viable, hypertrophic chondrocytes without evidence of mineralization or vascular invasion. Trabecular bone: In lesions of osteochondrosis latens, trabecular bone is normal. Osteochondrosis manifesta lesions, however, include direct contact of the subchondral bone with an area of necrotic cartilage. Marked myelofibrosis and bony remodeling, with formation of locally extensive areas of woven bone, are nearly always present; however, inflammatory cells are rarely a feature of the lesion. A closely similar bony reaction is seen in lesions of physeal osteochondrosis. In both sites, cleft formation may occur at the junction of the retained cartilage and the underlying bone or within the necrotic cartilage (see the following section on Osteochondrosis Dissecans). Cortical bone: Lesions in cortical bone are not expected at any stage of osteochondrosis of the AECC complex or physis. Osteochondrosis Dissecans. Osteochondrosis dissecans (OCD) is the name given to osteochondrosis at the AECC that forms clefts in the necrotic cartilage with subsequent fracture of the overlying articular cartilage (Fig. 16.44; also see Fig. 16.43; E-Fig. 16.20; also see E-Fig. 16.19). The result is a cartilaginous or osteochondral flap, depending on whether bone is present within the lesion. This may change with the duration of the lesion because cartilaginous flaps that have a blood supply will ossify over time. OCD can be accompanied by pain, joint effusion, and nonspecific secondary lymphoplasmacytic synovitis. Free-floating chondral or osteochondral fragments occasionally interfere with mechanical movement of the joint. Common sites of OCD are the same as those of osteochondrosis manifesta (see the previous section). The disease is extremely common in young breeding pigs and is a significant cause of lameness in this species. Although less common in horses and dogs, it is also an important cause of lameness in these species. The articular cartilage defect in OCD has poor healing capabilities, and such joints commonly develop some degree of DJD Surgical removal of the flap reduces the long-term clinical consequences; however, the defect repairs by the formation of fibrocartilage, which provides suboptimal biomechanical properties to the joint surface compared with articular cartilage. For the development of OCD, clefts must develop in the necrotic epiphyseal cartilage of the AECC, usually at the stage of osteochondrosis manifesta (see Fig. 16.42). Cleft formation can cause mechanical instability and lead to separations within cartilage or between cartilage and the underlying bone. External pressure, most commonly resulting from normal physiologic activity, can cause the cleft to extend through the overlying articular cartilage, resulting in the formation of a flap (see Figs. 16.43 and 16.44). At this stage of the disease, the affected animal may exhibit lameness. Cartilage flap formation involving extensive areas of multiple joints can develop in horses without predisposing lesions of osteochondrosis latens or manifesta. In these cases, articular cartilage of normal thickness can be peeled from the subjacent bone because of fissuring in the deeper layers of the AECC. Such lesions have been seen in foals that have licked fences with zinc-based white paint. The pathogenesis of the lesion is thought to involve copper deficiency induced by the zinc excess (zinc blocks the absorption of copper from the gastrointestinal tract). Copper is a required cofactor for enzymes that facilitate cross-linkages between tropocollagen molecules; however, these foals do not appear to have a generalized collagen dysplasia. The extensive distribution of the articular lesions CHAPTER 16 Bones, Joints, Tendons, and Ligaments A C B E-Figure 16.20 Osteochondrosis of the Articular-Epiphyseal Complex (AECC), Bone. A, Humerus, pig. The retained cartilage has separated from the subchondral bone (arrows), but a fissure extending to the articular surface (osteochondrosis dissecans) has not formed. B, AECC, distal femur, horse. A linear fissure between the thickened epiphyseal cartilage and subjacent underlying (subchondral) bone is extending into adjacent normal (right) articular cartilage (arrow). Hematoxylin and eosin (H&E) stain. C, Humerus, horse. Note the linear fissure within the epiphyseal cartilage of the AE complex, which likely occurred secondary to ischemic necrosis of cartilage before the occurrence of a failure of endochondral ossification. H&E stain. (A and B courtesy Dr. S.E. Weisbrode, College of Veterinary Medicine, The Ohio State University. C courtesy Dr. C. Bridges, College of Veterinary Medicine, Texas A&M University; and Dr. J. King, College of Veterinary Medicine, Cornell University.) 1062.e1 CHAPTER 16 Bones, Joints, Tendons, and Ligaments * A * B d * C Figure 16.44 Osteochondrosis Dissecans of the Articular-Epiphyseal Cartilage Complex (AECC), Bone, Humerus, Distal End, Immature Pig. A, Partially detached cartilage fragment (asterisk) involving almost the entire trochlea (medial aspect). B, Partially detached cartilage flap (involving the right half of the figure) subjacent to which are cartilage fragments and bone dust (asterisk); synovial fossa (arrow) separates medial from lateral aspect of the distal humerus. Hematoxylin and eosin (H&E) stain. C, High-magnification image of cleft; necrotic chondrocytes (asterisk); chondrocyte cluster/clone (arrow); bone dust (d). H&E stain. (Courtesy Dr. C.S. Carlson and Dr. E.J. Olson, College of Veterinary Medicine, University of Minnesota.) distinguishes them from OCD, which occurs multifocally in speciesdependent predilection sites. Growth plate and AECC: The growth plate is not involved in OCD. The lesion in the AECC includes a locally extensive area of 1063 fibroplasia, neovascularization, and bony remodeling at the chondro-osseous junction. Even in the most chronic lesions, variably sized areas of epiphyseal cartilage necrosis (usually a thin margin) often can be identified at the chondro-osseous junction or along the deep surface of the articular cartilage. The articular cartilage may be morphologically normal, other than the presence of a fissure that extends from the necrotic zone of epiphyseal cartilage to the articular surface. In cases in which the cartilage fragment has been displaced, all or part of the AECC is replaced by an area of cartilage ulceration that overlies reactive and fibrotic subchondral bone. Trabecular bone: The severity of changes in trabecular bone of the epiphysis reflects the extent of the cleft formation. Marked myelofibrosis, locally extensive osteopenia, and formation of reactive woven bone are expected at the base of the flap. Cortical bone: Lesions in cortical bone are not expected with OCD. Epiphysiolysis. Epiphysiolysis is the separation of the epiphysis from the metaphysis as the result of the formation of a horizontal fissure through an abnormal physis. The condition is most common in pigs and dogs but is also reported in calves, lambs, and foals. In market-weight pigs and in young gilts, the femoral head may be involved, whereas in sows, separation of the ischial tuberosity (site of insertion of semimembranosus, semitendinosus, and biceps femoris muscles) at its growth plate (often bilaterally) is a common cause of caudal weakness and inability to stand; it often occurs at parturition. In dogs, the process of epiphysiolysis can affect the anconeal process of the ulna, resulting in a condition known as ununited anconeal process. This may occur as one aspect of a condition known as the elbow dysplasia syndrome, which includes one or more of the following: OCD of the medial humeral condyle, fragmentation of the medial coronoid process, and ununited anconeal process. Elbow incongruity (shortening of the ulna relative to the radius secondary to lesions in the distal ulnar growth plate) is recognized as the major cause for the manifestations of elbow dysplasia. Because these lesions have primarily been studied in their chronic stages, the nature of the initial lesion is unclear; however, it is strongly suspected that they occur secondary to an extensive area, or to multifocal coalescing areas, of physeal osteochondrosis. A similar disease, known as physeal dysplasia with slipped capital femoral epiphysis, is described in young cats, most commonly in overweight, castrated males. The growth plates in these cats are widened and appear to remain open longer than expected. Histologically, the growth plates have a disorganized appearance in which chondrocytes are present in clusters, rather than columns, and are separated by variable amounts of extracellular matrix. It has been hypothesized that these dysplastic lesions may be present in all growth plates in affected animals, but fractures occur only in sites (e.g., femoral head) where shear forces occur during weight bearing. Transphyseal fractures occurring secondary to this condition are distinguished from traumatic fractures histologically by the presence of irregular clusters of chondrocytes separated by abundant extracellular matrix on both the epiphyseal and metaphyseal sides of the physeal cleavage site versus retention of the linear arrangement of chondrocytes on both sides of the growth plate (traumatic fracture). Osteochondrosis is rarely reported in cats, and the histologic changes in affected and contralateral growth plates are more characteristic of a physeal dysplasia (a diffuse process that affects the entire physis) than of osteochondrosis (focal to multifocal physeal lesions) in this species. Several types of underlying conditions may result in partial closure of the physis, causing an angular limb deformity in the affected limb. This condition is most common in horses, in which it usually occurs secondary to lesions of physeal osteochondrosis. Other causes include trauma and inflammation of the growth plate and/or primary trabeculae. 1064 SECTION II Pathology of Organ Systems Growth plate: Grossly there is a horizontal fissure/fracture through the physis with complete or partial separation of the epiphysis from the metaphysis. In cats, the remaining physeal cartilage is dysplastic, as described previously (disorganized arrangement of chondrocytes with separation by variable amounts of extracellular matrix). In fissures occurring secondary to osteochondrosis, the remaining physeal cartilage on the metaphyseal side of the growth plate contains areas of delayed endochondral ossification. The chronicity of the lesions (with accompanying secondary changes) complicates their interpretation. Trabecular bone: The response of trabecular bone in epiphysiolysis is characteristic of a reaction to trauma and fracture and includes myelofibrosis, proliferation of reactive woven bone, and the presence of variable amounts of hemorrhage and fibrin. Cortical bone: There are no primary changes in cortical bone with this condition; any lesions that develop would be secondary to altered mechanical use. Cervical Vertebral Myelopathy. Cervical vertebral stenotic myelopathy, sometimes called “Wobbler syndrome,” is a neurologic disease of horses and dogs (usually giant breeds) that occurs secondary to static or dynamic compression of the spinal cord by abnormally developed cervical vertebrae. This compression can be constant as the result of an anatomic stenosis of the spinal/vertebral canal, termed cervical vertebral static stenosis (CVS), or may occur only during movement (usually flexion), which is termed cervical vertebral instability (CVI). CVS involves the less mobile caudal cervical vertebrae, mainly C5-C6 and C6-C7, and most commonly affects older horses (usually 1 to 4 years old). In contrast, the spinal cord lesions of CVI are centered on the more mobile cranial cervical vertebrae, primarily at C3-C4 and C4-C5, in younger horses (6 to 15 months of age). In both syndromes, anatomic localization of the lesion by neurologic examination, myelography, or computed tomography is very helpful to the pathologist. In thoroughbred and standardbred horses and in dogs, the static compression is usually the result of a grossly appreciable narrowing of the spinal canal from caudal to cranial within a single vertebral body that almost certainly occurs during development (Fig. 16.45; E-Fig. 16.21). In adult quarter horses, localized hyperplasia and fibrocartilaginous metaplasia of the ligamentum flavum (the ligament between the dorsal lamina), likely occurring secondary to chronic mechanical irritation and/or trauma, can protrude into the spinal canal, causing static compression of the spinal cord and closely similar clinical signs. The lesions associated with dynamic compression of the spinal cord are more variable and less definitive because identical lesions often are present in asymptomatic animals. In horses, the most common lesions are those of osteochondrosis (see previous discussion) of the cervical facets. Osteochondrosis can cause abnormal and asymmetric development of the facets or abnormally large cranial vertebral epiphyses. With either lesion, the dorsal aspect of the vertebral body may compress the ventral aspect of the spinal cord during flexion, causing transient ischemia. Such compression may initially cause functional deficits without lesions in the spinal cord but also may progress to produce notable Wallerian degeneration (see Chapter 14, Nervous System). The frequency of osteochondrosis of the cervical vertebrae in clinically normal horses is the same as in horses with clinical signs of CVI; however, it is more severe in the latter cases. In addition, the frequency and severity of osteochondrosis lesions in the appendicular skeleton are greater in horses with clinical signs of CVI, further supporting the hypothesis that osteochondrosis may be an important underlying cause for this condition. Localized Skeletal Dysplasias. A wide variety of anomalies of the appendicular and axial skeleton have been described in domestic animals. Additional malformations of the vertebral column (see A B Figure 16.45 Static Stenosis, Bone, Cervical Vertebra, Macerated Specimen, Horse. A, Caudal aspect. B, Cranial aspect. Note the narrowing of the spinal canal from caudal to cranial. (Courtesy University of Minnesota Veterinary Diagnostic Laboratory Archives.) previous discussion on cervical vertebral myelopathy) are discussed later, and the terminology describing defects of skeletal development in the head, limbs, and digits is summarized in Box 16.3. Malformations of the vertebral bodies are most common and often occur in the thoracic region of the vertebral column. Alterations in the normal curvature of the spine include (1) lordosis (abnormal ventral curvature of the vertebral column, Fig. 16.46, A); (2) kyphosis (abnormal dorsal curvature of the vertebral column, Fig. 16.46, B); and (3) scoliosis (lateral deviation of the vertebral column, Fig. 16.46, C). Also see Box 16.3 and Fig. 14.70 in Chapter 14, Nervous System. The cause of vertebral malformations is generally believed to be caused by failure of vertebral ossification centers to form and/or fuse properly during embryonic or fetal development; however, the underlying cause of most congenital spinal deformities is not known (proposed mechanisms include congenital absence of vertebral vasculature, genetic defects, teratogenic insult to active cartilaginous proliferation, metabolic disease, lysosomal storage diseases, and toxin exposure); it is thought that some cases may be acquired. In dogs, any breed can be affected; however, the congenital vertebral body malformations appear to be more common in brachycephalic, CHAPTER 16 Bones, Joints, Tendons, and Ligaments E-Figure 16.21 Static Stenosis, Bone, Cervical Vertebra, Sagittal Section, Horse. Static stenosis (narrowing) of the vertebral canal caused by malformation of the vertebra (bottom vertebra, arrows). The top vertebra is normal. (Courtesy College of Veterinary Medicine, University of Illinois.) 1064.e1 CHAPTER 16 Bones, Joints, Tendons, and Ligaments 1065 Box 16.3 Skeletal Anomalies LIMBS (APPENDICULAR SKELETON) melia: absence of one or more limbs A Hemimelia: partial absence of part of the distal limb Peromelia: a severe, congenital malformation of a limb or absence of the extremity of a limb Polydactyly: an increase in the number of digits (see Fig. 16.47, B) Polymelia: an increase in the number of limbs (see Fig. 16.47, A) Syndactyly: partial or complete fusion of functional digits Valgus: angular limb deformity in which the limb distal to the lesion deviates laterally Varus: angular limb deformity in which the limb distal to the lesion deviates medially A HEAD (AXIAL SKELETON) Brachygnathia inferior (mandibular brachygnathism): shortening of the mandible Brachygnathia superior (maxillary brachygnathism): shortening of the maxilla “screw-tailed” breeds such as the English bulldog, French bulldog, Boston terrier, and pug. Congenital vertebral malformations can be classified by defects of segmentation (in which portions of adjacent vertebral elements fail to divide, including block vertebrae) and defects of vertebral formation (in which a portion of vertebral element is deficient, including hemivertebrae and butterfly vertebrae). However, classification systems have been inconsistent, and the authors recommend a review of the literature for further details. Congenital anomalies of the vertebral column may also be associated with anomalies of the spinal cord and/or other systems. The clinical significance of vertebral malformations can range from incidental finding to neurologic signs associated with progressive vertebral canal stenosis and instability of the vertebral column. Other anomalies of the skeleton include polymelia, an increase in the total number of limbs Fig. 16.47, A), and polydactyly, an increase in the number of digits (Fig. 16.47, B) (also see Box 16.3). Metabolic Bone Diseases Metabolic bone diseases are systemic skeletal diseases that are generally of nutritional, endocrine, or toxic origin (Table 16.1; E-Table 16.1) and occur in both growing and adult skeletons. Metabolic bone diseases are often called osteodystrophies, which is a general term that implies defective bone formation. The classic metabolic osteodystrophies are osteoporosis, FOD, rickets, and osteomalacia. These terms imply specific pathologic lesions, but these lesions may occur as a result of multiple different causes. In addition, different osteodystrophies can coexist in the same skeleton. In fact, most nutritional deficiencies in domestic animals are multiple, relatively mild, and do not produce the “classical” lesions that occur under experimental conditions. Osteoporosis. Osteoporosis is a disease in which bone fractures occur secondary to a reduction in bone density or mass (Fig. 16.48). The bone present, although reduced in amount, is normally mineralized. When there is reduced bone mass but no clinical disease (fractures), the term osteopenia is more appropriate; however, once fractures occur, the disease is called osteoporosis. In senile osteoporosis in human beings, in addition to decreased bone density, the turnover rate of bone is reduced, allowing microcracks (small cracks in the bone visible only microscopically) to accumulate. These B C Figure 16.46 Axial Skeleton, Malformations. A, Lordosis, horse. Lordosis is an abnormal ventral curvature of the vertebral column. Note the ventral deviation of the mid-thoracic aspect of the spine. B, Kyphosis, pig. Kyphosis is an abnormal dorsal curvature of the vertebral column. Note the dorsal deviation of the mid-thoracic aspect of the spine. C, Scoliosis, thoracic vertebrae, ventrodorsal view, sheep. Note the lateral deviation of the spinal column (arrows). (A courtesy Dr. H. Leipold, College of Veterinary Medicine, Kansas State University and Noah’s Arkive. B courtesy Dr. W. Crowell, College of Veterinary Medicine, The University of Georgia and Noah’s Arkive. C courtesy Dr. M.D. McGavin, College of Veterinary Medicine, University of Tennessee.) microcracks, superimposed on the reduced bone mass, make bones more brittle than would be predicted from the reduced mass alone. In growing animals, osteoporosis is potentially reversible; however, in adults the loss of trabecular bone is generally considered to be permanent. Although osteoporosis affects both cortical and trabecular bone, the loss of trabecular bone occurs earlier because of its higher surface area, which provides a greater opportunity for osteoclastic bone resorption. Although the thickness and density of cortical bone generally determine bone strength, trabecular bone contributes significantly to bone strength in some locations, including the femoral neck, vertebral bodies, and distal radius in human beings, CHAPTER 16 Bones, Joints, Tendons, and Ligaments E-Table 16.1 Toxic Osteodystrophies Principal Route/Source Vitamin D Ingestion of plants (e.g., Solanum malacoxylon) that contain watersoluble glycosides of the vitamin; ingestion of excess vitamin D added to feed Vitamin A Lead Fluoride 1065.e1 Lesions Mechanism Acute high dose: increased absorption of calcium from intestines and increased release of calcium by osteoclasts from bone Chronic lower dose: suppression of Chronic lower dose exposure: bone resorption by hypercalcemia, osteosclerosis with formation of which suppresses PTH and abnormal woven bone matrix with increases calcitonin secretion. increased basophilia Vitamin D also likely has direct stimulatory effect on osteoblasts. Retinoids cause degeneration and Growing animals: multifocal Ingestion of excessive retinoids (not necrosis of chondrocytes and premature closures of growth carotenoids) as found in certain osteoblasts in growing animals plates with subsequent growth plants (sweet potatoes) and meat and paradoxically are stimulatory to deformity, osteoporosis, and (liver) or drug toxicity osteoblasts in adult animals. pathologic fractures Adult animals: osteosclerosis, periosteal bone formation, and exostoses Lead is stored in bone mineral; part of Characteristic lesion is a “lead Ingestion usually from licking the sclerosis seen radiographically line,” an osteosclerotic band of lead-based paint (batteries/ is a result of the radiodensity of the bone parallel to the growth plate; environmental exposure) lead; lead is toxic to osteoclasts; osteoclasts can have acid-fast bone mineral containing lead is lead inclusions; osteoporosis somewhat resistant to osteoclasis. also can be present but is likely secondary to systemic illness of lead toxicity At lower toxic levels, fluoride is Ingestion of rock phosphates naturally Chronic low toxic levels cause stimulatory to osteoblasts, and its osteosclerosis; chronic higher high in fluoride; grazing on pastures incorporation in the hydroxyapatite toxic levels cause osteopenia with naturally high fluoride soil makes the crystal more resistant to and periosteal bone formation content or contaminated with resorption. At higher toxic levels, fluoride as industrial by-product; the mechanisms are uncertain, but drinking water with naturally high direct toxic injury to osteoblasts is fluoride content possible, resulting in osteopenia accompanied by periosteal proliferation. PTH, Parathyroid hormone. Acute high-dose exposure: widespread soft tissue mineralization 1066 SECTION II Pathology of Organ Systems new (reduced) level. Postmenopausal osteoporosis is a common and important disease in human beings and occurs primarily as a result of declining concentrations of estrogens. Osteopenia associated with reduced estrogens from ovarian atrophy or ovariectomy appears to be greatest in animals with estrous cycles that extend throughout the year (e.g., rat, pig, and primate), rather than being seasonal. Growth plate: Lesions in the growth plate are not expected in osteoporosis, unless the disease is related to pituitary dysfunction or protein calorie malnutrition, in which case the growth plate would be reduced in thickness. Trabecular bone: Trabeculae become thinner, are decreased in number, and develop full-thickness perforations as a result of osteoclastic resorption that result in loss of trabecular continuity. With time, the normal structure of trabecular bone (anastomosing plates) is replaced by smaller, more widely spaced trabeculae that do not interconnect but are separated by marrow spaces. The loss of trabecular continuity results in reduced ability of the trabeculae to withstand stress. Cortical bone: Cortical bone becomes thin because of osteoclastic resorption on the endosteal surface, with corresponding enlargement of the medullary cavity. The porosity of the cortex also increases because of increased osteoclastic resorption within the cortical vascular spaces and Haversian systems and/or decreased osteoblastic activity. With increased time or severity of disease (osteopenia), the resulting tissue may more closely resemble trabecular bone than cortical bone (Fig. 16.49). In severe cases, loss of cortical bone results in an increased susceptibility to fracture. A B Figure 16.47 Appendicular Skeleton, Malformations. A, Polymelia, sheep (lamb). Polymelia refers to an increase in the total number of limbs. This lamb has two forelimbs, two hindlimbs, and two limbs that appear to originate from the cranial to middle aspect of the thorax. B, Polydactyly, cat (palmar aspect). Polydactyly refers to an increase in the number of digits. This cat had seven digits on each forelimb paw (depicted in image) and five digits on each hindlimb paw. (A courtesy Dr. A. Wuenschmann, College of Veterinary Medicine, University of Minnesota. B courtesy Dr. J.A. Dykstra, College of Veterinary Medicine, University of Minnesota.) thus explaining why osteoporotic fractures occur most commonly in these locations. Causes of osteopenia include calcium deficiency, starvation, physical inactivity, hypogonadism, and chronic glucocorticoid administration. Calcium deficiency can result in hypocalcemia, which is compensated for by increased PTH output, resulting in increased bone resorption. Starvation and malnutrition can result in arrested growth and osteoporosis, largely because of reduced bone formation resulting from deficiencies of protein and mineral. Reduced physical activity (disuse or immobilization osteoporosis) causes increased bone resorption and decreased bone formation. This loss of bone from disuse may be mediated through changes in piezoelectrical activity, streaming potentials, and stretch receptors that are able to detect decreased mechanical use of the skeleton. Loss of bone mass associated with long-term paralysis or immobilization is not necessarily progressive; rather, the skeleton stabilizes at a Rickets and Osteomalacia. Failure of mineralization with subsequent bone deformities and fractures is called rickets in the growing skeleton and osteomalacia (soft bone) in the adult. Rickets is a disease of bone and epiphyseal (growth) cartilage in immature animals, whereas osteomalacia is a disease of adults in which the lesions are confined to bone. Affected animals have bone pain, pathologic fractures, and deformities such as kyphosis and scoliosis (see previous section on Localized Skeletal Dysplasias). The most common causes of rickets and osteomalacia are deficiencies of vitamin D or phosphorus. However, both disorders can occur in chronic renal disease and in chronic fluorosis. Dietary phosphorus deficiency is not common but can occur in herbivores grazing on phosphorus-deficient pastures. Phosphorus-deficient animals often have reduced feed intake, are unthrifty, and have impaired reproductive performance. Because of the common addition of this vitamin to commercial feed, vitamin D deficiency is rare in domestic animals other than in New World primates (in which vitamin D2 appears to be less active than vitamin D3) and “sun-basking” reptiles (when housed indoors). In addition, animals exposed to adequate sunlight should be able to synthesize vitamin D if their kidneys are normal. Although calcium deficiency is not commonly considered to be a cause of rickets in animals (other than birds), studies in human beings provide evidence that some cases of rickets may be attributable to extremely low dietary calcium intake in the presence of adequate vitamin D intake, particularly in infants and younger children (versus adolescents). In addition, low dietary calcium is known to exacerbate the development of vitamin D deficiency rickets in children. The mechanisms of this phenomenon are not known; however, it has been shown that knockout mice with no receptors for vitamin D develop rickets that can be corrected by feeding a high-calcium diet. Growth plate: The growth plates in rickets are diffusely but irregularly thickened because of failure of cartilage matrix mineralization and endochondral ossification (Fig. 16.50). This lesion may be evident in the costochondral junctions as prominent, nodular CHAPTER 16 Bones, Joints, Tendons, and Ligaments Table 16.1 1067 Metabolic Bone Disease Disease Characteristics Causes Osteoporosis Reduced bone mass: porous, thin, and fragile bones Osteomalacia (adult animals) Rickets (growing animals) Protein calorie malnutrition, immobilization, dietary calcium deficiency, glucocorticoid excess, estrogen or androgen deficiency, and advanced age Vitamin D deficiency, phosphorus deficiency Decreased bone mineralization (accumulation of osteoid): soft bones Decreased bone mineralization (accumulation of Vitamin D deficiency, phosphorus deficiency osteoid): soft bones; thickened hypertrophic zone of growth plate (failure of endochondral ossification) Hyperparathyroidism Decreased bone mass and increased pliability of bone resulting from resorption and 1. Primary: functional chief cell adenoma of parathyroid replacement by fibro-osseous tissue gland 2. Paraneoplastic: parathyroid hormone–related protein (and possibly also interleukin-1 [IL-1] and transforming growth factors α and β [TGF-α and TGF-β]) secreted from neoplasms such as adenocarcinoma of apocrine glands of anal sac 3. Secondary nutritional: from diets low in calcium and high in phosphorus 4. Secondary renal: from failure of the kidney to secrete phosphorus and reduced synthesis of 1,25-dihydroxyvitamin D Fibrous osteodystrophy (FOD) Figure 16.48 Osteoporosis, Bone, Two Cervical Vertebrae, Sagittal Section, Horse. Note the markedly thin cortices, particularly dorsally. The thickness of trabeculae also has been reduced, but this is difficult to appreciate grossly. The vertebra to the right has a compression fracture, causing shortening of the length of the vertebral body between the growth plates and fracture of the ventral cortex. The marrow has been flushed from the specimen to illustrate the bone changes. (Courtesy Dr. S.E. Weisbrode, College of Veterinary Medicine, The Ohio State University.) thickenings that historically have been called rachitic rosary because of the resemblance to a string of prayer beads. This thickening is apparent grossly as retained epiphyseal cartilage. Microscopically, the columns of chondrocytes in the growth plate may appear somewhat disorganized. In mammals, there is an increased number of chondrocytes in the zone of hypertrophy compared with normal, and this increase may be marked. It is uncertain if the disorganization of chondrocytes in vitamin D–deficiency rickets is caused by a primary effect of a lack of vitamin D metabolites (specifically, 24,25-dihydroxyvitamin D) or to a mechanical consequence of the failure of endochondral ossification. Because of the inadequate absorption of calcium, the rachitic growth plate does not undergo mineralization. In mammals, when mineralization of the cartilage matrix does not occur, blood vessels with accompanying chondroclasts do not A B Figure 16.49 Osteopenia, Bone, Metatarsal, Sheep. A, There is marked reduction in the number and length of metaphyseal trabeculae. B, The cranial cortex (right) is markedly porous (trabeculated), and the caudal cortex (left) is thin. The marrow has been flushed from the specimen. (Courtesy Dr. S.E. Weisbrode, College of Veterinary Medicine, The Ohio State University.) invade the physis and the process of endochondral ossification does not proceed. Trabecular bone: Grossly and radiographically, the metaphyses are “flared” in rickets because of failure of bone and cartilage removal in the cutback zones (see Fig. 16.50). Poorly mineralized matrices cannot be resorbed because osteoclasts cannot bind to an unmineralized matrix (see previous discussion). Microscopically, 1068 SECTION II Pathology of Organ Systems Figure 16.50 Vitamin D Deficiency (Experimental), Bone, Tibia, Chicken. Left specimen: rachitic tibia; middle and right specimens: normal chicken and a chicken fed a vitamin D–deficient diet supplemented with calcitriol. The latter two specimens appear normal and are indistinguishable from each other. The growth plate in the rachitic bird is thickened. The arrowheads indicate the junction between the growth plate and the epiphyseal cartilage. The metaphysis has not undergone modeling (“cutback”). In the normalappearing bones, notice the tapering of the metaphysis (“cutback” zone) and the thickness of the growth plate. In these normal chickens, the cleft (arrows) separating the growth plate from the epiphyseal cartilage is an artifact. There is no ossification center present in the epiphysis, which is normal for young broilers. Hematoxylin and eosin (H&E) stain. (Courtesy Dr. L. Nagode.) the surfaces of trabecular bone in rickets and osteomalacia contain excessive amounts of osteoid (unmineralized matrix) (Fig. 16.51). Because osteoclasts are not able to adhere to or resorb osteoid, bone modeling and remodeling are impaired. Hypocalcemia can develop concurrently with vitamin D deficiency, and lesions of secondary hyperparathyroidism (FOD [see later discussion]) also can develop. Cortical bone: Grossly, cortical bone can appear normal or the softened bone can be deformed by weight bearing. In severe cases, the bones are so soft that they can be cut with a knife. Microscopically, many endocortical and trabecular surfaces in the cortex contain wide seams of unmineralized osteoid. Osteomalacic bone is susceptible to accumulation of microcracks because of the impaired remodeling and modeling secondary to the inability of osteoclasts to bind to surfaces covered by osteoid. Fibrous Osteodystrophy. FOD is the name given to the skeletal lesions that result from primary hyperparathyroidism, secondary hyperparathyroidism, and pseudohyperparathyroidism (also known as humoral hypercalcemia of malignancy [HHM]). These disorders are characterized by increased, widespread osteoclastic resorption of bone and replacement by primitive fibro-osseous tissue, which results in a weakened bone structure. Sequelae include lameness, pathologic fractures, and deformities. As described previously, osteoblasts—but not osteoclasts—have receptors for PTH and respond by upregulating production of RANKL and downregulating secretion of OPG. Bone marrow stromal cells also have receptors for PTH, and when the hormone is expressed constantly at high levels, these cells differentiate into fibroblasts. Paradoxically, intermittent (versus continuous) dosing of PTH has the opposite effect on bone (increased number of osteoblasts and increased bone formation) and is used clinically in human beings for the treatment of osteoporosis. In domestic animals, primary hyperparathyroidism, which includes functional parathyroid adenoma, parathyroid carcinoma, and idiopathic bilateral parathyroid hyperplasia, is rare. Secondary hyperparathyroidism is more common and can be either nutritional or renal in origin. Nutritional hyperparathyroidism is caused by dietary factors that tend to decrease the concentration Figure 16.51 Osteomalacia, Bone, Cross Section, Metaphysis, Trabeculae, Human Being. This person had osteomalacia secondary to malabsorption of fat-soluble vitamins (including vitamin D) associated with gastrectomy. This section was not demineralized and was stained to show the difference between osteoid (red) and fully mineralized bone (blue-green). Normally, no more than 10% of trabecular surfaces should be covered by osteoid seams; however, in this case almost all surfaces are covered by osteoid. Goldner’s trichrome stain. (Courtesy Dr. S.E. Weisbrode, College of Veterinary Medicine, The Ohio State University.) of serum ionized calcium, to which the parathyroid glands respond by increasing output of PTH. Nutritional hyperparathyroidism is most common in young, growing animals that are fed rations that are deficient in calcium and have a relative excess of phosphorus. Unsupplemented cereal grain rations fed to pigs, all-meat diets fed to dogs and cats, and bran fed to horses are examples of low-calcium, high-phosphorus diets that can cause secondary nutritional hyperparathyroidism and, eventually, FOD. Increased concentrations of dietary phosphorus are important in the evolution of FOD, perhaps by interfering with the intestinal absorption of calcium. Pseudohyperparathyroidism is also known as HHM and involves the release of circulating humoral factors (e.g., parathyroid hormone-related peptide [PTHrP], interleukins, transforming growth factors, and tumor necrosis factors) from malignant neoplasms (e.g., lymphoma and apocrine carcinoma of the anal sac glands in dogs); this condition is discussed in Chapter 12, Endocrine System (see also the section on Proliferative and Neoplastic Lesions). Growth plate: No lesions are expected in the growth plate or AECC. Trabecular bone: Trabeculae are replaced by irregular spicules of woven bone that are separated by variable amounts of fibrous connective tissue and are accompanied by numerous osteoclasts. Osteoblastic proliferation can be marked but is ineffectual in that little osteoid is produced. Proliferation of fibrous tissue in the marrow space is usually marked but may be subtle in early stages, consisting of only two or three layers of fibroblasts between the bone lining cells and the marrow space (Fig. 16.52; tunneling resorption). In severe cases, the marrow cavity is filled with fibrous tissue. Cortical bone: Bone resorption and replacement by fibrous tissue can be so extensive that the bone becomes pliable (E-Fig. 16.22). Clinically and at postmortem examination, severely affected bones can be bent at a right angle without fracture. This outcome, when it affects the mandible, has been called rubber jaw. Osteoclastic resorption begins on the endocortical bone surface, but any vascular spaces within the bone can undergo marked enlargement by osteoclastic resorption and replacement by fibrous tissue. In advanced disease, the entire width of the cortex may be replaced by reactive woven CHAPTER 16 Bones, Joints, Tendons, and Ligaments A C 1068.e1 B D E-Figure 16.22 Fibrous Osteodystrophy, Bone, Maxillae and Mandibles. A, Nutritional fibrous osteodystrophy, horse. Note the swelling of the facial crest. B, Maxillae, cross section, horse. Proliferating and poorly organized fibro-osseous tissue in the maxillae has distorted and extended their contours laterally and compressed the nasal cavity medially. Note the absence of normal bone. C, Renal fibrous osteodystrophy, dog. Exuberant poorly mineralized woven bone and fibrous tissue has caused the maxillary bones to expand and distort the positioning of the teeth. Similar changes affect the mandible, and the teeth at this site are also malpositioned. This outcome when it affects the mandible has been called “rubber jaw.” D, Radiograph, renal fibrous osteodystrophy, dog. Note the reduced bone density (radiodensity) of the proliferative woven bone and fibrous tissue that has replaced the preexisting maxillary bones and the absence of normal radiodense bone. Postmortem transverse slab section. (A and D courtesy Dr. S.E. Weisbrode, College of Veterinary Medicine, The Ohio State University. B courtesy Dr. W. Crowell, College of Veterinary Medicine, The University of Georgia; and Noah’s Arkive, College of Veterinary Medicine, The University of Georgia. C courtesy Department of Veterinary Biosciences, The Ohio State University; and Noah’s Arkive, College of Veterinary Medicine, The University of Georgia.) CHAPTER 16 Bones, Joints, Tendons, and Ligaments bone and fibrous tissue. The proliferation of fibrous tissue may be so exuberant that the external dimension of the bone is increased (Figs. 16.53, 16.54, and 16.55). This lesion is most common in the maxilla and mandible and may reflect the response of the weakened bone to the intense mechanical stress of mastication. Inflammation Infectious Inflammation. Inflammation of bone is termed osteitis. Periostitis is the appropriate term if the periosteum is involved, and osteomyelitis is the appropriate term if the medullary cavity (and bone marrow) of the bone is involved (Table 16.2). These conditions generally occur together and may be life threatening, requiring early diagnosis and vigorous treatment. They often involve the simultaneous necrosis and removal of preexisting bone and the compensatory production of new bone, occurring over a prolonged period of time. Infectious inflammation of bone in animals is usually caused by bacteria. Hematogenous bacterial osteomyelitis is uncommon in dogs and cats, but it is common in neonatal foals and animals used for food and fiber production (Fig. 16.56). A wide range of Grampositive and Gram-negative bacteria are responsible for hematogenous osteomyelitis in calves and foals. Trueperella (formerly known as Arcanobacterium) pyogenes and other pyogenic bacteria (e.g., Streptococcus spp. and Staphylococcus spp.) and Salmonella spp., Escherichia coli, and other coliforms are among the most common microbes that cause hematogenous osteomyelitis. Staphylococcus intermedius is the most common cause of hematogenous osteomyelitis in dogs. Diskospondylitis (“spondyl” = spine/vertebral body) refers to an infection (inflammation) of the intervertebral disk with concurrent osteomyelitis of contiguous vertebrae (see Fig. 16.82). A Figure 16.52 Tunneling Resorption (within a Trabecula), Fibrous Osteodystrophy, Bone, Longitudinal Section, Dog. Osteoclasts (arrows) have resorbed the central portion of the trabecula, and this tissue is being replaced by fibrous tissue rather than bone. Hematoxylin and eosin (H&E) stain. (Courtesy Dr. S.E. Weisbrode, College of Veterinary Medicine, The Ohio State University.) 1069 B Figure 16.53 Fibrous Osteodystrophy, Bone, Maxillae. A, Maxillae, cross section, horse. Proliferating and poorly organized fibro-osseous tissue in the maxillae has distorted and extended their contours laterally and compressed the nasal cavity medially. Note the absence of normal bone. B, Radiograph, renal fibrous osteodystrophy, dog. Note the reduced bone density (radiodensity) of the proliferative woven bone and fibrous tissue that has replaced the preexisting maxillary bones. Postmortem transverse slab section. (A courtesy Dr. W. Crowell, College of Veterinary Medicine, The University of Georgia; and Noah’s Arkive, College of Veterinary Medicine, The University of Georgia. B courtesy Dr. S.E. Weisbrode, College of Veterinary Medicine, The Ohio State University.) A B C D E Figure 16.54 Fibrous Osteodystrophy, Bone, Maxillae and Mandibles, Dromedary Camel. A, Swollen maxillae and mandible of affected camel with displaced mandibular incisor teeth, right lateral aspect. B, Dorsoventral view of mandible of unaffected camel. C, Dorsoventral view of affected rostral mandible showing fibro-osseous proliferation and displaced incisor teeth. D, Ventrodorsal view of unaffected rostral maxillae. E, Ventrodorsal view of affected maxillae showing marked locally extensive enlargement. (A, C, and E courtesy Dr. A.G. Armién, Dr. E.J. Olson, and Dr. N.A. Robinson, College of Veterinary Medicine, University of Minnesota. B and D courtesy Dr. J.A. Dykstra and Dr. A. Wuenschmann, College of Veterinary Medicine, University of Minnesota.) 1070 SECTION II Pathology of Organ Systems * * * A * * B Figure 16.55 Fibrous Osteodystrophy, Bone, Maxilla, Dromedary Camel. A, Multiple small bony trabeculae (arrows) separated by loose fibrous connective tissue (asterisks) that fills marrow spaces. Oral mucosa (arrowhead) lines the upper surface of the tissue. Hematoxylin and eosin (H&E) stain. B, Primitive new bone formation (asterisks) accompanied by numerous osteoclasts (arrows) within resorption/erosion lacunae and surrounded by loose fibrovascular connective tissue. H&E stain. (Courtesy Dr. C.S. Carlson and Dr. E.J. Olson, College of Veterinary Medicine, University of Minnesota.) Hematogenous spread of bacteria (similar list as those described previously for osteomyelitis as well as Brucella canis) is one of the most common causes. Vertebral infections caused by grass awn migration are often associated with mixed bacterial infections. Fungi, viruses, and protozoa also can cause bony lesions. Mycotic agents, such as Coccidioides immitis and Blastomyces dermatitidis, frequently spread hematogenously to bone to produce granulomatous and pyogranulomatous osteomyelitis, accompanied by bone lysis and variable amounts of new bone formation. The viruses of hog cholera (classical swine fever; pestivirus) and infectious canine hepatitis (canine adenovirus type 1 [CAV-1]) can cause endothelial damage, resulting in metaphyseal hemorrhage, necrosis, and acute inflammation. Osseous localization of the canine distemper virus injures osteoclasts, resulting in disrupted metaphyseal modeling and producing a growth retardation lattice (described previously). A variant of the FeLV has been associated with myelosclerosis (increased density of medullary bone) in cats; however, neither canine distemper virus nor FeLV causes inflammation of bone. Canine hepatozoonosis caused by Hepatozoon americanum (an apicomplexan protozoal organism) is an emerging tick-borne disease of dogs in North America. In addition to the characteristic skeletal and cardiac myositis, there is disseminated periosteal new bone formation (proliferation) in many affected dogs. It is thought that cytokines elaborated by the animal in response to the infection are involved, and the bone lesions have been attributed to periosteal stimulation by humoral factors rather than local ones. Growth plate: Epiphyseal cartilage (Fig. 16.57) (cartilage of the epiphysis that has yet to undergo endochondral ossification) and physeal cartilage can be eroded by invasion of inflammation from adjacent bone or may undergo direct bacterial embolization via cartilage canal blood vessels or metaphyseal vessels at sites of endochondral ossification (see the section on Portals of Entry/Pathways of Spread). Growth cartilage may appear thickened secondary to osteomyelitis because of disruption of endochondral ossification by the inflammatory process and the resulting failure to replace cartilage with bone. In growing animals, articular cartilage can undergo lysis by extension of osteomyelitis from the subjacent epiphyseal cartilage of the AECC, a lesion that may be mistaken for primary arthritis (see Fig. 16.34). Trabecular bone: The composition of the exudate in metaphyseal osteomyelitis is determined by the infectious agent and typically is purulent in bacterial infections in domestic animals. Exudate in the medullary cavity increases the intramedullary pressure and can cause compression of blood vessels, resulting in thrombosis and infarction of intramedullary fat, hematopoietic marrow, and bone. In areas of inflammation, bone resorption is mediated primarily by osteoclasts that are stimulated by prostaglandins and cytokines released by local tissue and inflammatory cells. Reduced blood flow through large vessels also promotes osteoclastic bone resorption, possibly by altering electrostatic charges in bone. Proteolytic enzymes released by inflammatory cells and activation of matrix metalloproteinases by the acidic environment of inflammation also assist in resorbing matrix. Lack of drainage and persistence of the offending agent in areas of necrotic bone account for the chronicity of the process, which may continue for years. Inflammation in the medullary cavity also may penetrate into and through cortical bone and undermine the periosteum, where it can further disrupt the blood supply to the bone at the nutrient foramen and nutrient canal. Cortical bone: The lesions involving cortical bone that occur with infectious osteomyelitis may vary based on the route of entry of the organism and the nature of the exudates. Bone lysis is expected with suppurative inflammation and is subperiosteal for bacteria induced traumatically via the periosteum and endosteal in cases of embolic osteomyelitis. Lysis within the cortex begins within existing vascular channels and can occur with either route of entry. Periostitis can develop by direct inoculation from trauma (e.g., puncture wounds) or by centrifugal spread of inflammation from the marrow cavity and through the cortex. Chronic bacterial periostitis is characterized by multiple coalescing pockets of exudate and areas with variable amounts of periosteal new bone formation and cortical lysis. A classic example of this entity is mandibular or maxillary osteomyelitis occurring secondary to infection with Actinomyces bovis, also known as “lumpy jaw” (Fig. 16.58). Additional sequelae of osteomyelitis include extension of inflammation to adjacent bone, hematogenous CHAPTER 16 Bones, Joints, Tendons, and Ligaments Table 16.2 1071 Lesions of Inflammatory Joint Disease Time/Exudate Synovial Fluid Synovial Membrane Articular Cartilage Acute suppurative Reduced viscosity Neutrophils Reduced viscosity Neutrophils Reduced viscosity Neutrophils Hyperemia Edema Hyperplasia Lymphoplasmacytic inflammation Hyperplasia Lymphoplasmacytic inflammation Fibrosis Hyperemia Edema Hyperplasia Lymphoplasmacytic inflammation Hyperemia Lymphoplasmacytic inflammation Fibrin Pannus No lesions Subacute suppurative Chronic suppurative Acute fibrinous Reduced viscosity Fibrin Reduced viscosity Fibrin Reduced viscosity Fibrin Subacute fibrinous Chronic fibrinous * Figure 16.56 Vertebral Abscess (Suppurative Osteomyelitis), Bovine. The necrotic vertebral body in the center of the image has been disrupted by yellow-white exudate. The overlying spinal cord (asterisk) is focally compressed. The bone flanking the abscess is sclerotic. (Courtesy Dr. M.D. McGavin, College of Veterinary Medicine, University of Tennessee.) Figure 16.57 Embolic (Suppurative) Epiphysitis, Bone, Distal Femur, Foal. Bacterial emboli in the articular-epiphyseal cartilage complex (AECC) have produced suppurative inflammation that has destroyed both the subchondral bone and the overlying articular cartilage of the right condyle. (Courtesy Dr. S.E. Weisbrode, College of Veterinary Medicine, The Ohio State University.) Usually no lesions Erosion Ulceration No lesions Usually no lesions Erosion Ulceration Pannus spread to other bones and to soft tissues, pathologic fractures, and development of sinus tracts that penetrate cortical bone and drain to the exterior (Fig. 16.59). Occasionally, fragments of dead bone become isolated from their blood supply and surrounded by exudate (bone sequestrum; plural, sequestra). Sequestra can form when bone fragments are contaminated at the site of a compound fracture, when the fragments at a fracture site become infected hematogenously, or when fragments of necrotic bone become isolated (and thus avascular) in osteomyelitis (Fig. 16.60). These sequestra and associated exudates can become surrounded by a dense collar of reactive bone, which is termed the involucrum. Extracellular matrix is not living tissue; therefore, it cannot be resorbed in an area in which the cells (bone or marrow) are necrotic. For this reason, relatively large sequestra can persist for long periods of time and may interfere with repair. Grossly, they often become pale and chalky and lack the glistening appearance of normal bone (see Fig. 16.60). Aseptic Necrosis Aseptic necrosis of bone in human beings occurs in a variety of clinical conditions, including occlusive vascular disease (bone infarction), hyperadrenocorticism, fat embolism, nitrogenous embolism, sickle cell anemia, and intramedullary neoplasms, all of which likely result in arterial or venous infarction of the bone (Table 16.3). In domestic animals, aseptic necrosis of bone has been associated with intramedullary neoplasms and various nonneoplastic lesions, which likely result in decreased venous outflow from the bone and increased bone marrow pressure. The long-term use of corticosteroids in human beings has been associated with necrosis of the femoral head in adults, and this lesion has been reproduced experimentally in several animal species (e.g., mice and rabbits). The gross appearance of necrotic bone varies with the extent of the affected area and the response of the body to it. Microscopically, the hallmark of bone necrosis is cell death and loss of osteocytes from their lacunae, which must be distinguished from similar changes that may occur secondary to over-decalcification of the sample. After an episode of ischemia leading to infarction, the cellular elements of the marrow lose their differential staining, and circular spaces (pooled lipid) develop within a few days. If the region of dead bone remains avascular, the coagulated tissue and mineralized matrix can persist for some time. Dead osteocytes elicit little reaction; their nuclei become pyknotic and their cytoplasm becomes eosinophilic, but their disappearance from lacunae is slow and may not be complete for 2 to 4 weeks. 1072 SECTION II Pathology of Organ Systems t * A B Figure 16.58 Chronic Pyogranulomatous Osteomyelitis, Actinomycosis (Actinomyces bovis), Maxilla, “Lumpy Jaw,” Cow. A, Transverse section of the maxilla. The nodules apparent within the mass in the maxilla represent pockets of pyogranulomatous inflammation that are surrounded by fibrous tissue and woven bone (asterisk); tooth (t). B, Macerated and bleached specimen of the mandible. Note the spicules of woven bone radiating from the mandible. Within the spaces formed by this reactive bone were nodules of pyogranulomatous inflammation and colonies of Actinomyces bovis. (A courtesy University of Minnesota Veterinary Diagnostic Laboratory Archive. B courtesy Dr. S.E. Weisbrode, College of Veterinary Medicine, The Ohio State University.) Figure 16.59 Chronic-Active (Suppurative) Osteomyelitis, Bone, Mandible, Transverse Section, Sheep. Chronic suppurative osteomyelitis has caused a fistulous tract (arrows) that penetrates through the full dorsoventral thickness of the mandible. This lesion likely began as a periodontal bacterial infection. (Courtesy Dr. S.E. Weisbrode, College of Veterinary Medicine, The Ohio State University.) Figure 16.60 Suppurative Periostitis and Osteomyelitis, Phalanges, Horse. Trauma to the dorsal aspect of the hoof inoculated bacteria into the subcutis, causing suppurative cellulitis and periostitis and, subsequently, cortical osteolysis of the dorsal aspect of the distal first phalanx and the entire dorsal surface of the second phalanx. From there, the infection spread to the distal interphalangeal joint and then to the third phalanx, where it caused suppurative osteomyelitis, loss of articular cartilage, and formation of a sequestrum in the proximal portion of the third phalanx (arrow). The viable tissue immediately adjacent to the sequestrum is not different from that which is more distant, implying that in this case an involucrum (reactive bone surrounding the exudate around a sequestrum) was not formed. (Courtesy Dr. S.E. Weisbrode, College of Veterinary Medicine, The Ohio State University.) CHAPTER 16 Bones, Joints, Tendons, and Ligaments Table 16.3 1073 Necrosis of Bone Cause/Duration of Necrosis Lesion Acute aseptic (ischemic) necrosis Clinical Significance Death of bone cells and marrow; bone structure intact Chronic aseptic (ischemic) necrosis 1. If no revascularization, dead bone can remain intact structurally for a long time but will accumulate microcracks. 2. If revascularization takes place, dead bone can be resorbed slowly and replaced by new bone. 3. If revascularization takes place, dead bone may be resorbed slowly and NOT be replaced by bone but by fibrous tissue. Acute septic necrosis Exudate, usually suppurative, forms at junction of dead bone and viable tissue with subsequent bone lysis and reactive bone formation. Chronic septic necrosis 1. If the focus of dead bone is relatively small, it can be completely resorbed by the inflammatory process and osteoclastic bone lysis; there can be marked modeling of bone, usually with resorption predominating and replacement by fibrous tissue; in some cases, excess formation can occur. 2. If the focus of dead bone is relatively large, it can be sequestered (sequestrum) by a peripheral wall of reactive fibrous tissue or reactive bone (involucrum). Reaction to and repair of necrotic bone requires revascularization that is associated with infiltration of macrophages and invasion by fibrous tissue that advances from the margins of the lesion. The bone marrow may eventually regenerate entirely, or a scar may form and remain. The matrix of necrotic bone remains fully mineralized and may even “hypermineralize” because of calcification of the dead osteocytes and their lacunae. This mineralization is only possible if there is vascularization that brings additional calcium to the region. Dead bone is slowly removed by osteoclasts. The resorption of necrotic bone with simultaneous replacement by new bone is termed creeping substitution. The process is slow and often incomplete. Small areas of remaining bone necrosis might not be detected clinically or radiographically. Growth plate: Ischemic necrosis of metaphyseal bone and bone marrow can result in retained growth cartilage. The metaphyseal necrosis would not directly affect the zones of proliferative and hypertrophic chondrocytes; however, the physeal thickness would increase because the conversion of epiphyseal cartilage to bone by endochondral ossification at the chondro-osseous junction would be impaired. Ischemic necrosis of the epiphysis could result in premature closure of the growth plate because of the death of the proliferating chondrocytes, which depend on the epiphyseal blood vessels. Endochondral ossification would continue normally if the metaphyseal blood supply was not affected, and the growth plate would close because of failure to generate new proliferating zone chondrocytes. Trabecular bone: In the femoral heads of young, small, and miniature breed dogs, aseptic necrosis of the femoral head (LeggCalvé-Perthes disease) is associated with clinical signs because of the collapse of the articular cartilage as a result of resorption of the necrotic subchondral bone that occurs late in the course of the disease. Apparently, the initial infarction is asymptomatic (Fig. 16.61; E-Fig. 16.23). The cause of the infarction is usually not determined; the etiology is thought to be multifactorial, with disruption of blood supply to the femoral head playing a key role in development of ischemic necrosis. In clinical cases of aseptic necrosis, the dead bone is not replaced by creeping substitution but is ultimately resorbed and replaced by fibrous tissue. The fibrous tissue does not provide adequate support for the articular cartilage, and the femoral head Can be clinically silent 1. Can remain clinically silent, but risk of microcrack progressing to complete clinical fracture increases with time and mechanical use 2. Can remain clinically silent from initiation of lesion to completion of repair 3. Can cause structural failure and collapse of the bone (e.g., chronic idiopathic necrosis of the femoral head) Pain caused by cytokines from the inflammation and pressure in marrow cavity and periosteum Pain caused by cytokines and pressure in marrow cavity and periosteum; draining tracts can form; strength of the bone can be weakened because of resorption, or its function can be affected by exuberant new bone formation * Figure 16.61 Bone Necrosis (Chronic Osteochondrosis Dissecans Lesion), Femur, Distal End, Adult Human Being. Osteocyte necrosis represented by empty osteocyte lacunae (arrows); marrow spaces filled with necrotic cellular debris (asterisk). Hematoxylin and eosin (H&E) stain. (Courtesy Dr. C.S. Carlson and Dr. E.J. Olson, College of Veterinary Medicine, University of Minnesota.) collapses. In stages preceding complete resorption, it is common, in revascularized necrotic medullary bone, for reactive new bone to be deposited on trabeculae of necrotic bone (Fig. 16.62). This “sandwich” of central dead bone covered by viable reactive woven bone can persist for months and may give (along with osteocyte mineralization described previously) the affected region a radiodense appearance. Ultimately, in clinical cases of aseptic necrosis of the femoral head, even these foci of new bone formed over dead bone are resorbed and replaced by fibrous tissue. CHAPTER 16 Bones, Joints, Tendons, and Ligaments A B E-Figure 16.23 Femoral Head, Necrosis (Experimental), Bone, Femur, Pig. Necrosis was produced experimentally by a ligature placed around the femoral neck. Several days after the procedure, the only difference between the control (A) and the infarcted bones (B) is the coagulation necrosis of the marrow and bone cells. The hard tissue of the cartilage and bone remains unaffected. Hematoxylin and eosin (H&E) stain. (Courtesy Dr. S.E. Weisbrode, College of Veterinary Medicine, The Ohio State University.) 1073.e1 1074 SECTION II Pathology of Organ Systems Figure 16.62 Revascularization, Ischemic Necrosis (Experimentally Induced), 1-Month Duration, Femoral Head, Epiphyseal (Cancellous) Bone, Pig. Reactive woven bone (between arrows) has been deposited on the surface of the necrotic bone in which lacunae are empty or contain pale eosinophilic nuclear remnants. Fibrovascular repair tissue in the marrow surrounds the bone. Hematoxylin and eosin (H&E) stain. (Courtesy Dr. S.E. Weisbrode, College of Veterinary Medicine, The Ohio State University.) Cortical bone: Large areas of necrotic cortical bone have a dry, chalky gross appearance, and the periosteum can be removed easily. These areas can remain as subclinical lesions for years. The formation of sequestra almost always requires inflammation; sequestra formation after sterile necrosis of bone is unusual. Proliferative and Neoplastic Lesions Surprisingly, bone as a tissue offers little resistance to an expanding or invading neoplasm, and many skeletal neoplasms are accompanied by bone resorption as well as new bone formation. Pain, hypercalcemia, increased serum alkaline phosphatase activity, pathologic fracture, and distant metastases are other possible manifestations of a skeletal neoplasm. New bone formation occurs, at least in part, in response to mechanical stress on a weakened cortex and is prominent in neoplasms that have a marked fibrous stroma. Neoplasms with little stroma, such as plasma cell myeloma and lymphoma, are associated with minimal reactive bone formation, even though bone may be destroyed by marked bone lysis. Tumor-associated bone destruction is largely accomplished by osteoclasts, but prostaglandins, cytokines, acid metabolic by-products, and lytic enzymes released by inflammatory or neoplastic cells can also be responsible for local bone resorption and formation. Hypercalcemia, a result in part of bone resorption induced by release of bone-resorbing factors from extraskeletal neoplasms, is well documented and is termed humoral hypercalcemia of malignancy (HHM). The best-known examples in animals occur in dogs secondary to adenocarcinoma of the apocrine glands of the anal sac and T-cell lymphoma, in which the neoplastic cells produce parathyroid hormone-related protein (PTHrP). Other tumors include myeloma, leiomyosarcoma, and squamous cell carcinoma (especially in horses); other humoral effectors of HHM include IL-1, IL-6, TGF-α, TGF-β, and TNF-α. Nonneoplastic Proliferative and Cystic Lesions. The nonneoplastic proliferative and cystic lesions considered here vary widely in their cause, structure, and ultimate effect on the host. Reactive bone formation, sometimes exuberant, can occur in fracture repair, chronic osteomyelitis, and DJD in the form of periarticular osteophytes. An exostosis is a nodular, benign, bony growth projecting outward from the surface of a bone. An osteophyte is a similar growth that occurs at the margins of a diarthrodial (movable) joint. An enthesophyte is the ossification of a tendon or ligament (forming abnormal bony projections) at the point of its insertion into the bone. In addition to bone, these proliferations may include variable amounts of cartilage. The bone component can be woven and/or lamellar, depending on rate of growth and duration of lesion. The term hyperostosis usually is used to indicate that the diameter of the bone has increased and implies more uniform thickening on the periosteal surface rather than the nodular appearance of an osteophyte or exostosis. An enostosis is a bony growth within the medullary cavity, usually originating from the cortical-endosteal surface, and can result in obliteration of the medullary cavity. These are nonneoplastic proliferative lesions in which growth is seldom continuous. Some exostoses can remodel (e.g., an osteophyte may become indistinguishable from the preexisting bone over time, and its presence is only recognizable by a change in shape of the affected area), and some regress. Nonneoplastic proliferative lesions can be mistaken for skeletal neoplasia, particularly in small biopsy specimens. Conversely, a malignancy may be missed when small superficial biopsies contain only nonneoplastic reactive bone that is present adjacent to the tumor. These statements serve to highlight the problem of making a morphologic diagnosis from a small biopsy specimen, without the benefit of a clinical history, radiographic findings, and other laboratory data. One must also remember that more than one process may be active at any one site (e.g., osteosarcoma may be complicated by fracture repair or by osteomyelitis). Hypertrophic Osteopathy (Hypertrophic Pulmonary Osteopathy). Hypertrophic osteopathy (HO/HPO) occurs in human beings (known as Marie’s disease; hypertrophic pulmonary osteoarthropathy) and a variety of domestic animal species, with dogs being the most commonly affected. The disease is characterized by progressive, often bilateral, periosteal, new bone formation in the diaphyseal and metaphyseal regions, particularly of the distal limbs, that occurs as a secondary reaction to a primary space-occupying lesion (Figs. 16.63 and 16.64; E-Fig. 16.24). The earliest bony involvement (radiographic evidence) often includes the abaxial aspects of the second and fifth metacarpal and/or metatarsal bones. The word “pulmonary” is sometimes included because most cases occur in association with intrathoracic neoplasms (as a paraneoplastic syndrome) or inflammation. Other, less commonly associated lesions or ag