Muscle 2K24 PDF
Document Details
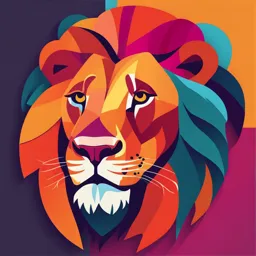
Uploaded by MagnificentRecorder
Tags
Summary
This document is a lecture or course material covering skeletal muscle. It details the functions of muscle tissue, the different types, and their properties. The document includes various diagrams to illustrate the key concepts.
Full Transcript
Chapter 12 Muscle Lecture Topics: The functions of muscle tissue. The three types of muscle tissue found in the human body. Skeletal muscle terminology. Skeletal muscle structure. Skeletal muscle fiber structure and ultrastructure. The Sliding Filament Theor...
Chapter 12 Muscle Lecture Topics: The functions of muscle tissue. The three types of muscle tissue found in the human body. Skeletal muscle terminology. Skeletal muscle structure. Skeletal muscle fiber structure and ultrastructure. The Sliding Filament Theory of Muscle Contraction. Molecular events of the sliding filament theory The roles of Troponin and Tropomyosin Excitation Contraction Coupling Events Skeletal Muscle Fiber Types The Motor Unit and Skeletal Muscle Contraction Isotonic vs Isometric Skeletal Muscle Contraction ATP and Muscle Contraction Factors that Contribute to Muscle Fatigue Characteristics of Skeletal vs. Cardiac vs. Smooth Muscle 3 Primary Functions of Muscle: 1) To produce movement. 2) To generate force for doing work Moving loads (objects) from one place to another. 3) To generate heat. The Three Types of Muscle Tissue Skeletal muscle Aka: “Striated” muscle Cardiac muscle Smooth muscle This banding pattern is a result of the organization of the proteins that produce contraction in this type of muscle. Skeletal muscle = somatic muscle The Three Types of Muscle Tissue Again this banding pattern is a result of the organization of the proteins that produce contraction in this type of muscle. Cardiac muscle = heart muscle The Three Types of Muscle Tissue The same proteins are involved in contraction of smooth muscle as in the types of striated muscle tissue, but the proteins are not as organized as in striated muscle tissue hence the banding pattern is not seen. Smooth muscle = visceral muscle Skeletal Muscle Usually attached to bones by tendons (but some attach to soft tissues, like the lips). Skeletal MuscleTerminology: Origin: attachment point to the skeletal element that doesn’t move when the muscle contracts. Usually close to the torso of the body. Triceps Insertion: attachment point to the skeletal muscle Biceps muscle element that moves when the muscle contracts. Usually more distal along the limb. Flexor: reduces the joint angle. Ex. Biceps muscle flexes the elbow joint. Extensor: increases the joint angle. Ex. Triceps muscle extends the elbow joint. Antagonistic muscle groups: flexor- extensor pairs move skeletal elements in opposite directions at a joint. (Ex. Biceps- Triceps muscles). Antagonistic Muscle Groups Contraction of the biceps muscle decreases the angle of the joint between the humerus and radius Humerus bone Joint angle Radius (~900) bone Antagonistic Muscle Groups Contraction of the triceps increases Joint the angle of the angle joint between the (~1800) humerus and radius Anatomy Summary: Skeletal Muscle Contractile component of muscle. Attaches to the tendon at each end of the muscle. *Connective tissue of the muscle is the elastic component. Both the elastic and contractile components are important in normal muscle functioning. The Muscle Fibers: Result of fusion of individual muscle cells during development of the muscle. Long and cylindrical in shape. Has multiple nuclei. Each muscle cell that fused to create the fiber contributes its nucleus. Ends of each muscle fiber are attached to the ends of the muscle. When muscle fibers contract, the muscle contracts. Ultrastructure of a Muscle Fiber Inside of a muscle fiber * * T-tubules and the Sarcoplasmic Reticulum * T-tubules are small infoldings of the sarcolemma which have terminal cisternae of sarcoplasmic reticulum on both sides (all of which is referred to as a triad). The t-tubules conduct the muscle action potential deep into the muscle fiber, which triggers the release of calcium from the terminal cisternae leading to the contraction of the muscle. Ultrastructure of Muscle Fibers: The Myofibril The myofibrils of a muscle fiber are composed of the proteins that interact to produce muscle contraction. The proteins compose thick and thin filaments that are organized into repeating units called sarcomeres that are linked to form a myofibril. So, a myofibril is a chain of sarcomeres. Thin filaments (pink) Thick filaments (purple) Each end of a myofibril is attached to the ends of the muscle fiber. The Proteins of the Sarcomere: Proteins of the Thick Filament Myosin is a “motor protein” consisting of a tail region, a hinge region, and a head region. 250 myosin molecules are bundled to form the thick filaments of a sarcomere. Flex points The Proteins of the Sarcomere: Proteins of the Thin Filament Thin filaments of the sarcomere are composed four proteins: Actin, Tropomyosin, Troponin, and Nebulin. 1) G-Actin: Globular Protein that polymerizes into F-actin chains that twist together to form the thin filaments. 2) Tropomyosin: covers myosin binding sites on the actin to prevent complete binding by the myosin head. 3) Troponin: involved in shifting the tropomyosin to expose the myosin binding sites during contraction. 4) Nebulin: aligns and supports the thin filament. It is not involved in contraction. Actin and Myosin form crossbridges During contraction myosin heads of the thick filaments bind to the G-actin molecules of the thin filaments at myosin-head binding sites, forming what are called crossbridges between the thick and thin filaments. Crossbridges Thin filament Thick filament Ultrastructure of Muscle Fibers: The Sarcomere Sarcomere Terminology: A repeating unit of the myofibril. Includes a Z disk at each end, ½ of an I band, 1 A band, 1 H zone, and 1 M line. Z disk: Attachment site for thin filaments on the ends of the sarcomere and to the thin filaments of the adjacent sarcomere. I band: Region containing only thin filaments. A band: Encompasses the entire length of a thick filament. H zone: Clear zone in the A band that consists of thick filaments only. M line: Represents proteins that form the attachment site for thick filaments. Sarcomeres (c) A band Sarcomere Z disk Z disk Myofibril M line I band H zone (d) Z disk Z disk M line During a muscle contraction, the interaction between the myosin heads and G-actin pulls the Z disks towards the M line…. Review: Ultrastructure of Muscle Fibers (c) A band Sarcomere Z disk Z disk Myofibril M line I band H zone (d) Titin Z disk Z disk M line M line Thick filaments Review: Ultrastructure of Muscle Fibers (c) A band Sarcomere Z disk Z disk Myofibril M line I band H zone (d) Titin Z disk Z disk M line M line Thick filaments (e) Myosin heads Hinge Myosin tail region Myosin molecule Review: Ultrastructure of Muscle Fibers (c) A band Sarcomere Z disk Z disk Myofibril M line I band H zone (d) Titin Z disk Z disk M line M line Thick filaments Thin filaments (e) Myosin heads Hinge Myosin tail region Myosin molecule Review: Ultrastructure of Muscle Fibers (c) A band Sarcomere Z disk Z disk Myofibril M line I band H zone (d) Z disk Z disk M line M line Thick filaments Thin filaments (e) (f) Myosin heads Hinge Myosin tail region G-actin molecule Myosin molecule Actin chain Accessory Proteins: Titin and Nebulin Titin acts like a spring that returns the sarcomere to its original length after contraction has occurred. Nebulin helps align and support the thin filament. * * Sliding Filament Theory of Muscle Contraction According to this theory of how muscle contraction occurs muscle contraction (following an action potential at the neuromuscular junction that spreads along the sarcolemma): 1. The thin filaments of the sarcomeres slide along the thick filaments 2. The Z disks on each end of the sarcomere are pulled towards the M line (starts midway along a muscle fiber and proceeds towards each end). 3. Because the sarcomeres of myofibrils are shortened in length. 4. Because the myofibrils are attached to each end of the muscle fiber the muscle fiber is shortened in length. 5. Because the ends of the muscle fibers are attached to the tendons at each end of the muscle overall muscle length is shortened. The molecular events that result in sliding of thin filament along thick filament: (Six Steps) Myosin filament Tight binding in the rigor Myosin ATP binds to its binding site 1 45° 2 state. The crossbridge is binding ATP on the myosin. Myosin then at a 45° angle relative to sites binding dissociates from actin. the filaments. site 1 2 3 4 G-actin molecule ADP ATP 1 2 3 4 1 2 3 4 5 At the end of the power stroke, 6 The ATPase activity of myosin the myosin head releases ADP 3 hydrolyzes the ATP. ADP and and resumes the tightly bound Pi remain bound to myosin. rigor state. ADP Contraction- Pi relaxation Pi Sliding 1 2 3 4 1 2 3 4 5 filament Actin filament moves toward M line. 90° Pi 1 2 3 4 5 Release of Pi initiates the power The myosin head swings over and stroke. The myosin head rotates 4 binds weakly to a new actin molecule. on its hinge, pushing the actin The crossbridge is now at 90º relative filament past it. to the filaments. Tight binding in the rigor 1 state. The crossbridge is at a 45° angle relative to the filaments. Myosin 45 ° filament Myosin ATP binding binding sites site 1 2 3 4 G-actin molecule Tight binding in the rigor ATP binds to its binding site 1 state. The crossbridge is 2 on the myosin. Myosin then at a 45° angle relative to dissociates from actin. the filaments. Myosin 45 ° filament Myosin ATP ATP binding binding sites site 2 3 4 1 2 3 4 1 G-actin molecule The ATPase activity of myosin 3 hydrolyzes the ATP. ADP and Pi remain bound to myosin. ADP Pi 1 2 3 4 The ATPase activity of myosin The myosin head swings over 3 hydrolyzes the ATP. ADP and 4 and binds weakly to a new Pi remain bound to myosin. actin molecule. The cross- bridge is now at 90º relative to the filaments. ADP 90° Pi Pi 1 2 3 4 1 2 3 4 Release of Pi initiates the power 5 stroke. The myosin head rotates on its hinge, pushing the actin filament past it. Pi 1 2 3 4 5 Actin filament moves toward M line. Release of Pi initiates the power At the end of the power stroke, 5 stroke. The myosin head rotates 6 the myosin head releases ADP on its hinge, pushing the actin and resumes the tightly bound filament past it. rigor state. ADP Pi 1 2 3 4 1 2 3 4 5 5 Actin filament moves toward M line. The Regulatory Role of Tropomyosin and Troponin in Muscle Contraction IN RELAXED STATE FUNCTION OF MUSCLE FIBER: Tropomyosin partially covers the myosin binding sites Prevents myosin from binding fully and releasing Pi So myosin head can’t undergo power stroke Regulatory Role of Tropomyosin and Troponin in Muscle Contraction (b) Initiation of contraction 1 Ca2+ levels increase in cytosol. Ca2+ binds to 4 Power stroke 2 troponin. 3 Tropomyosin shifts, Pi Troponin-Ca2+ exposing binding ADP 3 complex pulls site on G-actin tropomyosin away from G-actin binding site. TN Myosin binds 4 to actin and completes power stroke. 2 5 Actin filament G-actin moves 5 moves. 1 Cytosolic Ca2+ Excitation-Contraction Coupling: The link between the muscle action potential and muscle contraction. Somatic motor neuron 1 releases ACh at neuro- muscular junction. (a) 1 Axon terminal of Muscle fiber ACh somatic motor neuron Motor end plate T-tubule Sarcoplasmic reticulum Ca2+ DHP receptor Tropomyosin Troponin Z disk Actin M line Myosin head Myosin thick filament Excitation-Contraction Coupling Somatic motor neuron Net entry of Na+ through ACh 1 releases ACh at neuro- 2 receptor-channel initiates muscular junction. a muscle action potential. (a) 1 Axon terminal of Muscle fiber ACh somatic motor neuron potential K+ 2 Action potential Na+ Motor end plate T-tubule Sarcoplasmic reticulum Ca2+ DHP receptor Tropomyosin Troponin Z disk Actin M line Myosin head Myosin thick filament Excitation-Contraction Coupling Action potential in 3 t-tubule alters conformation of DHP receptor. (b) 3 Ca2+ Excitation-Contraction Coupling Action potential in DHP receptor opens Ca2+ 3 t-tubule alters 4 release channels in conformation of sarcoplasmic reticulum DHP receptor. and Ca2+ enters cytoplasm. (b) 4 3 Ca2+ Ca2+ released Excitation-Contraction Coupling Action potential in DHP receptor opens Ca2+ 3 t-tubule alters 4 5 Ca2+ binds to troponin, release channels in conformation of allowing strong actin- sarcoplasmic reticulum DHP receptor. myosin binding. and Ca2+ enters cytoplasm. (b) 4 3 Ca2+ Ca2+ released 5 Excitation-Contraction Coupling Action potential in DHP receptor opens Ca2+ 3 t-tubule alters 4 5 Ca2+ binds to troponin, release channels in conformation of allowing strong actin- sarcoplasmic reticulum DHP receptor. myosin binding. and Ca2+ enters cytoplasm. (b) 4 3 Ca2+ Ca2+ released 5 6 Myosin thick filament M line 6 Myosin heads execute power stroke. Excitation-Contraction Coupling Action potential in DHP receptor opens Ca2+ 3 t-tubule alters 4 5 Ca2+ binds to troponin, release channels in conformation of allowing strong actin- sarcoplasmic reticulum DHP receptor. myosin binding. and Ca2+ enters cytoplasm. (b) 4 3 Ca2+ Ca2+ released 5 7 6 Myosin thick filament M line Distance actin moves Actin filament slides 6 Myosin heads execute 7 toward center of power stroke. sarcomere. A muscle fiber contracts completely and then repolarizes. That is it is an all-or-none event. When a muscle fiber repolarizes… The Ca2+ channels on SR close Ca2+ pumps on SR pump Ca2+ back into the SR This pulls Ca2+ off troponin Tropomyosin shifts back to partially cover myosin binding sites on G-actin molecules. Sarcomere is returned to its original length with help of titin (like a spring!). As the sarcomeres are returned to their original length, the muscle fiber returns to original length (it “relaxes”). As the muscle fibers relax the muscle relaxes. Skeletal Muscle Fiber Types There are three skeletal muscle fiber types: 1) Slow-twitch oxidative fibers 2) Fast twitch oxidative fibers 3) Fast-twitch glycolytic fibers Characteristics of the Three skeletal muscle fiber types Fiber type Slow Twitch Fast Twitch Fast twitch Oxidative oxidative glycolytic Characteristic Fibers (Type I) fibers fibers (Type IIa) (Type IIb) Myosin type Slow (0.1ms) Fast Fast (0.1ms) (0.01ms) Twitch Long (75ms) Short Short (7.5ms) duration (7.5ms) Fatigue High moderate low resistance resistance Color Deep red Pinkish White (lots of myoglobin) (less myoglobin) (little myoglobin) Used for Endurance Walking Fast postural (moderate) movements Muscle Fibers and Motor Units Most skeletal muscles are a mixture of muscle fiber types. Muscle fibers in a skeletal muscle are organized into motor units: A motor unit is composed of the motor neuron and all the muscle fibers it innervates. It is the basic functional unit of a skeletal muscle. The nervous system works in terms of motor units. The smallest possible contraction you can make with a skeletal muscle involves activation of a motor unit. Motor Units Recall that the cell body of a somatic motor neuron is located in the ventral horn of the spinal cord. The motor neurons send their axons out through the ventral root to innervate a group of muscle fibers in a skeletal muscle (color coded here). In this illustration this muscle has three motor units (MU 1, MU 2, and MU 3). Most muscles consist of 100s or even 1000s of motor units. Motor Units and Muscle Movements When a motor unit is activated, all the muscle fibers in that unit contract completely and then relax (i.e. it is an all-or-none event). The number of muscle fibers in a motor unit determines the amount of force generated when that motor unit is activated. The more muscle fibers in a motor unit, the more force it generates when it is activated. Muscles used for fine movements (eg. finger muscles) have few muscle fibers per motor unit (small motor units). These small motor units allow you to make fine movements with your fingers. Muscles used for gross movements (hip or shoulder muscles) have large numbers of muscle fibers per motor unit (large motor units). This allows these muscles to generate enough force to move your whole arm or leg. If each motor unit contracts in an “all-or-none” fashion, how can muscles create graded contractions of varying force? The nervous system can vary the force of contraction of a muscle in two ways: 1. By varying the type of motor units being activated in the muscle Small motor units have fewer muscle fibers and thus produce less force. So, if the task requires only a little force the nervous system will activate small motor units. Large motor units have many muscle fibers and thus produce greater force. So, if the task requires a greater force the nervous system will activate larger motor units. …The Nervous System Can Vary The Force of Contraction in Two Ways… 2. The nervous system can change the force of muscle contraction by changing the number of motor units that are being activated at any one time during the contraction. The force of contraction can be increased by recruiting additional motor units into the contraction. This is called “motor unit recruitment”. Motor Unit Recruitment occurs in a stereotyped way during a muscle contraction: During a contraction: Nervous system first activates a few small motor units in a muscle to produce a small amount of force. These small motor units are composed of slow twitch oxidative fibers. To increase the force of contraction the nervous system increases the number and size of the motor units being activated. As max force of contraction for the muscle is approached, the largest motor units in the muscle are activated, which are composed of fast twitch glycolytic fibers. Motor Unit Recruitment The fast twitch glycolytic fibers can’t sustain contraction for long period, so force of contraction drops as these motor units fatigue and drop out of the contraction. However, for most skeletal muscles it is possible to sustain submaximal contractions for relatively long periods. This is accomplished by “asynchronous recruitment”. In asynchronous recruitment: The nervous system alternates activation of motor units so that different motor units are maintaining the contraction at different times. This allows a sustained submaximal contraction of the muscle for relatively long periods. Two types of muscle contraction generate force: 1. Isotonic contractions: Muscle contracts, generates force, shortens in length, and moves a load. Two subtypes: Concentric action the muscle generates force during shortening. Eccentric action the muscle generates force during lengthening. 2. Isometric contractions: Muscle contracts, and generates force, but doesn’t shorten in length, and doesn’t move a load. Isotonic vs Isometric Contractions Series Elastic Elements in Muscle Connective tissue Muscle fibers ATP is required in steady supply during muscle contraction: ATP is needed: During contraction for release of myosin heads from the actin, To provide the energy to move of the myosin head from 45o to 90o angle. During relaxation of muscle fiber to provide energy for the Ca2+ pump to move Ca2+ ions back into the sarcoplasmic reticulum After excitation-contraction coupling to provide energy to the Na+/K+ ATPase pumps in the sarcolemma that restore the Na+ and K+ concentration gradients between the inside and outside of the muscle fiber. Muscle Fatigue: Fatigue is defined as a condition in which an active muscle is no longer able to generate its expected power output. Cause of fatigue are uncertain and are actively being researched. It is probably the result of a combination of factors. Classically fatigue has been divided into: - Central Fatigue (i.e. fatigue of the CNS and Motor Neurons). - Peripheral Fatigue (i.e. fatigue due to factors in the muscle fiber) Factors in Central Fatigue Maybe due to psychological factors (i.e. bored with repeated activity). It has also been proposed that lowered blood pH due to lactic acid production by muscles may contribute. Diminished supply of ACh at the neuromuscular junction. Factors in Peripheral Fatigue Extended submaximal exercise. – Thought to cause depletion of glycogen stores in the muscle which may affect Ca2+ from the SR. Short-duration maximal exertion. – Believed to cause increased levels of inorganic phosphate (Pi) in the muscle fibers which: – May slow Pi release from myosin head during contraction resulting in altering the powerstroke. – Another possibility is that the Pi combines with Ca2+ preventing the Ca2+ from being taken back up into the SR and so decreases calcium release from the SR during subsequent contractions. Ion imbalances resulting from prolonged muscle activity. – With each muscle action potential K+ leaves the muscle fiber hyperpolarizing the muscle fiber membrane potential and so moving it away from the threshold for triggering subsequent muscle fiber action potential. Characteristics of Skeletal vs. Cardiac vs. Smooth Muscle Skeletal Muscle Characteristics: Appearance under microscope: Striated Where found in body: Attached to skeletal elements Control: Voluntary (somatic motor subdivision) Morphology of cellular components: Muscle fiber is long, cylindrical with multiple nuclei Contraction is all-or-none. Contraction Speed: Faster than smooth and cardiac Internal cellular structure: Sarcoplasmic reticulum & T-tubules Characteristics of Skeletal vs. Cardiac vs. Smooth Muscle Cardiac Muscle Characteristics: Appearance under microscope: Striated Where found in body: heart Control: Involuntary (ANS innervation) Morphology of cellular components: Individual muscle cells, branched with single nucleus Contraction is graded (is NOT all-or-none) Contraction Speed: Intermediate Internal cellular structure: Sarcoplasmic reticulum & T-tubules Characteristics of Skeletal vs. Cardiac vs. Smooth Muscle Smooth Muscle Characteristics: Appearance under microscope: No striations Where found in body: walls of digestive tract, blood vessels, bronchioles, ducts of glands, ureter, and urinary bladder. Control: Involuntary (ANS innervation) Morphology of cellular components: Individual muscle cells, fusiform in shape with single nucleus Contraction is graded (is NOT all-or-none) Contraction Speed: Slowest of the three types of muscle Internal cellular structure: Little Sarcoplasmic reticulum & NO T-tubules (Caveloae bring Ca2+ in from extracellular fluid to supplement SR)