Miscon 2-1.pdf
Document Details
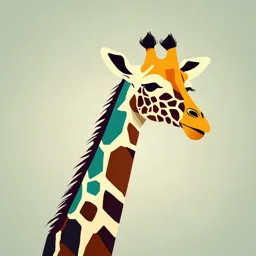
Uploaded by SpectacularRocket
Tags
Full Transcript
Summer Capacity Building Program Misconceptions and Realities (Truths) (High School Physics) – WU Part 2 Chapter 5 to Chapter 8 1|Page Unit 5 Mechanical Oscillation and Sound Wave 5.1. Prop...
Summer Capacity Building Program Misconceptions and Realities (Truths) (High School Physics) – WU Part 2 Chapter 5 to Chapter 8 1|Page Unit 5 Mechanical Oscillation and Sound Wave 5.1. Propagation of Waves Common Misconceptions About Wave Propagation Misconceptions about wave propagation are quite common, especially when it comes to understanding the fundamental nature of waves. Let's explore some of the most frequent ones: Misconception 1: Waves are Particles Reality: Waves are disturbances that propagate through a medium, transferring energy without transferring matter. While they can exhibit particle-like behavior under certain conditions (wave-particle duality), they are fundamentally different from particles. Misconception 2: Medium is Carried Along with the Wave Reality: The medium particles oscillate around their equilibrium positions, but they do not travel with the wave. It's the disturbance, or energy, that moves through the medium. Misconception 3: Sound Waves Travel Faster in Air Than in Solids Reality: Sound waves actually travel faster in solids than in liquids, and faster in liquids than in gases. The speed of sound depends on the density and elasticity of the medium. Misconception 4: All Waves Require a Medium Reality: While most waves require a medium to propagate (mechanical waves), electromagnetic waves can travel through a vacuum. Examples include light, radio waves, and X-rays. Misconception 5: Amplitude Determines Pitch Reality: Amplitude is related to the loudness of a sound wave, while pitch is determined by the frequency. Higher frequency corresponds to a higher pitch. Misconception 6: Waves Only Travel in Straight Lines 2|Page Reality: While waves can travel in straight lines, they can also bend, diffract, and interfere. These phenomena are essential to understanding many wave behaviors. 5.2. Sound Waves Common Misconceptions About Sound Waves Sound waves are often misunderstood, leading to several common misconceptions. Let's explore some of the most prevalent ones: Misconception 1: Sound can travel through a vacuum. Truth: Sound requires a medium (solid, liquid, or gas) to travel. It cannot propagate in a vacuum, as there are no particles to vibrate. This is why there is no sound in space. Misconception 2: Loudness and pitch are the same thing. Truth: Loudness and pitch are distinct qualities of sound. Loudness is related to the amplitude of the sound wave, while pitch is determined by its frequency. A loud sound can be high or low in pitch, and vice versa. Misconception 3: Hitting an object harder makes a higher-pitched sound. Truth: The force with which an object is struck primarily affects the loudness of the sound, not its pitch. The pitch is determined by the object's size, shape, and material properties. Misconception 4: Sound travels in a straight line. Truth: While sound waves can travel in a straight line under ideal conditions, they can also be reflected, refracted, and diffracted. This is why we can hear sounds around corners or obstacles. Misconception 5: Sound waves are like water waves. Truth: While both are types of waves, sound waves and water waves have significant differences. Sound waves are longitudinal waves, meaning the particles vibrate parallel to the direction of wave propagation. Water waves, on the other hand, are transverse waves, with particles moving perpendicular to the wave direction. 5.3. Superposition of Waves Common Misconceptions About Superposition of Waves 3|Page Superposition is a fundamental principle in physics that states that when two or more waves meet at a point, the resultant displacement at that point is the algebraic sum of the displacements of the individual waves. While this principle is straightforward, there are some common misconceptions about it. Misconception 1: Waves Physically Combine Truth: When waves superpose, they do not physically combine or interact with each other. The displacements of the individual waves simply add algebraically at each point in space and time. After the superposition, the waves continue to propagate independently. Misconception 2: Interference is a Destructive Process Truth: Interference is a phenomenon arising from superposition, but it's not necessarily destructive. It can be constructive (resulting in a larger amplitude), destructive (resulting in a smaller amplitude), or somewhere in between (resulting in a complex pattern). Misconception 3: Superposition Only Applies to Waves of the Same Type Truth: While superposition is often demonstrated with waves of the same type (eg, two sound waves), it actually applies to any type of wave, including light waves, water waves, and even quantum waves. Misconception 4: Superposition is Limited to Two Waves Truth: Superposition can occur with any number of waves. The principle states that the net displacement at a point is the algebraic sum of the displacements of all individual waves at that point. 4|Page Unit 6 Fluid Mechanics 6.1. Density and Specific Gravity Common Misconceptions About Density and Specific Gravity Misconception 1: Density and Specific Gravity are the same thing. Truth: While closely related, density and specific gravity are not identical. o Density is the mass of a substance per unit volume, typically measured in kg/m³ or g/cm³. o Specific gravity is the ratio of the density of a substance to the density of a reference substance (usually water). It is a dimensionless quantity. Misconception 2: Heavier objects always have a higher density. Truth: Weight and density are separate properties. A large, low-density object can weigh more than a small, high-density object. For example, a large balloon filled with helium is lighter than a small lead weight, but the lead has a much higher density. Misconception 3: Objects with higher density always sink in water. Truth: While generally true, there are exceptions. For instance, a hollow steel ball might float due to the trapped air, even though steel has a higher density than water. The concept of buoyancy plays a role here. Misconception 4: Density is a constant property of a substance. Truth: While density is generally considered constant for a given substance under specific conditions, it can vary with temperature and pressure. For example, the density of water changes with temperature. 6.2. The Young Modulus Common Misconceptions About Young's Modulus Misconception 1: Young's Modulus is a measure of material strength. Truth: Young's modulus is a measure of a material's stiffness, not its strength. A material with a high Young's modulus will deform less under stress but might still fail at a lower load than a material with a lower Young's modulus. 5|Page Misconception 2: Young's Modulus is constant for a given material. Truth: While Young's modulus is generally considered a material property, it can vary with factors like temperature, stress level, and the direction of the applied force. For instance, some materials exhibit anisotropic behavior, meaning their properties vary with direction. Misconception 3: A higher Young's modulus always implies a better material. Truth: This depends on the application. For example, a high Young's modulus is desirable for materials used in springs or beams, as they need to resist deformation. However, for applications requiring flexibility, a lower Young's modulus might be preferred. Misconception 4: Young's Modulus is the same as elasticity. Truth: Elasticity is a broader concept referring to a material's ability to return to its original shape after deformation. Young's modulus is just one parameter used to characterize the elastic behavior of a material. 6.3. Fluid Statics Common Misconceptions About Fluid Statics Fluid statics, while seemingly straightforward, often leads to misunderstandings. Let's address some common misconceptions: Misconception 1: Pressure in a fluid depends only on depth. Truth: While depth is a significant factor, pressure in a fluid also depends on the fluid's density and the external pressure applied to its surface (such as atmospheric pressure). Misconception 2: Pressure acts only downwards. Truth: Pressure in a fluid act in all directions equally. This is why fluid pressure can exert forces on the walls of a container. Misconception 3: Buoyant force is determined by the object's weight. Truth: Buoyant force depends on the weight of the fluid displaced by the object, not the object's weight itself. This is why objects less dense than the fluid float. Misconception 4: Pressure at a point in a fluid is directly proportional to the total weight of the fluid above that point. 6|Page Truth: While there is a relationship, it's more complex. Pressure depends on the fluid's density, the height of the fluid column, and the acceleration due to gravity. 6.4. Properties of Pressure in fluids Common Misconceptions About Pressure in Fluids Understanding pressure in fluids is essential in various fields, from physics to engineering. However, several misconceptions often arise. Let's explore some of the most common ones: 1. Pressure is Directly Proportional to Depth Only Misconception: Pressure in a fluid increases only with depth. Reality: While depth is a significant factor, pressure also depends on the fluid's density and the acceleration due to gravity. The formula P = ρgh (where P is pressure, ρ is density, g is acceleration due to gravity, and h is depth) clearly illustrates this. 2. Pressure Acts Only Downward Misconception: Pressure in a fluid acts solely downward. Reality: Pressure in a fluid act in all directions equally. This is why fluids transmit pressure equally in all directions, a principle fundamental to hydraulic systems. 3. Pressure in a Wider Pipe is Higher Misconception: A wider pipe holds more fluid, so the pressure is higher. Reality: The pressure in a fluid at a specific depth is independent of the pipe's width. It depends only on the fluid's density, depth, and gravity. However, the total force exerted by the fluid on the pipe wall will be greater in a wider pipe due to the larger area. 4. Pressure is a Force Misconception: Pressure and force are the same. Reality: Pressure is force per unit area. It's a measure of how concentrated a force is. Force is the push or pull exerted on an object. 5. Water Pressure is Higher Than Air Pressure 7|Page Misconception: Water, being denser than air, exerts more pressure. Reality: While water pressure can be significantly higher than atmospheric pressure at certain depths, it depends on the depth. At the surface, air pressure and water pressure are essentially equal. 6. Pressure Increases Indefinitely with Depth Misconception: Pressure keeps increasing as you go deeper into a fluid. Reality: While pressure generally increases with depth, it's not infinite. Factors like fluid compressibility and the immense pressure at the bottom of oceans limit this increase. 6.5. Archimedes Principle Common Misconceptions About Archimedes' Principle Archimedes' principle is a fundamental concept in physics, yet it's often misunderstood. Let's clarify some common misconceptions: 1. Buoyant Force Equals Weight of the Object Misconception: Many believe the buoyant force acting on an object is equal to the object's weight. Reality: The buoyant force is equal to the weight of the fluid displaced by the object. This is a crucial distinction. If the buoyant force is greater than the object's weight, it floats; if less, it sinks. 2. Bigger Objects Experience More Buoyant Force Misconception: Larger objects automatically experience a greater buoyant force. Reality: The buoyant force depends on the volume of fluid displaced, not the object's overall size. A large, low-density object might displace less fluid than a smaller, denser one, resulting in a smaller buoyant force. 3. Buoyant Force Acts Only on Completely Submerged Objects Misconception: The buoyant force only affects objects fully immersed in a fluid. 8|Page Reality: Any object partially or fully submerged in a fluid experiences a buoyant force. This is why ships float even though most of their volume is below the waterline. 4. Denser Objects Always Sink Misconception: Objects with a higher density than the fluid will always sink. Reality: While generally true, the shape of the object also plays a role. A dense, flat object might float due to its shape displacing a large volume of fluid. 5. The Buoyant Force Acts Upward at the Object's Center Misconception: The buoyant force is applied directly upward at the object's center. Reality: The buoyant force acts upward through the center of buoyancy of the displaced fluid, which is not necessarily the same as the object's center. Unit 7 Temperature and Thermometry 7.1. Temperature and our life Common Misconceptions About Temperature and Our Lives Temperature, a seemingly simple concept, often leads to misunderstandings. Let's explore some common misconceptions: 1. Heat and Temperature are the Same Misconception: Many people equate heat with temperature. Reality: Heat is a form of energy that can be transferred between objects, while temperature is a measure of the average kinetic energy of the particles in an object. 2. Hot Objects Contain More Heat Misconception: A larger, hotter object contains more heat than a smaller, cooler one. Reality: The amount of heat in an object depends on its mass, specific heat capacity, and temperature. A small, high-temperature object can contain a significant amount of heat. 3. Cold is an Absence of Heat 9|Page Misconception: Cold is often perceived as the opposite of heat. Reality: Cold is simply a lower level of heat energy. It's not a separate entity. Absolute zero is the theoretical lowest temperature, where particles have minimal kinetic energy. 4. The Thermometer Measures Heat Misconception: Thermometers directly measure the amount of heat in an object. Reality: Thermometers measure temperature, which is related to the average kinetic energy of particles. 5. Warmer Objects Always Lose Heat to Cooler Ones Misconception: Heat always flows from warmer to cooler objects. Reality: While this is generally true, heat transfer can also occur due to other factors like work and radiation. For instance, refrigerators work by transferring heat from a cold space to a warmer environment. 7.2. The concept of heat and its transfer mechanisms Common Misconceptions About Heat and Its Transfer Heat, a fundamental concept in physics, often leads to misunderstandings. Let's clarify some common misconceptions: Misconceptions about Heat Heat is a substance: Many people believe heat is a fluid-like substance that flows from hot to cold objects. o Reality: Heat is a form of energy transferred due to temperature differences. Hot objects contain more heat: This misconception is related to the first one. o Reality: The amount of heat in an object depends on its mass, specific heat capacity, and temperature. Cold is the absence of heat: People often think of cold as an opposite to heat. o Reality: Cold is simply a lower level of heat energy. It's not a separate entity. Absolute zero is the theoretical lowest temperature. 10 | P a g e Misconceptions about Heat Transfer Heat only travels upwards: This misconception is often related to the observation of hot air rising. o Reality: Heat can be transferred in all directions through conduction, convection, and radiation. Conductors are always hot: People might think that materials that conduct heat well are always hot. o Reality: Conductors efficiently transfer heat but their temperature doesn't necessarily need to be high. Insulators prevent heat generation: Some believe insulators stop heat from being created. o Reality: Insulators only slow down heat transfer. They don't prevent heat generation or absorption. Vacuum is a perfect insulator: While it's true that vacuum is an excellent insulator for conduction and convection, it doesn't completely block radiation. 7.3. Heat capacity and specific heat capacity Common Misconceptions About Heat Capacity and Specific Heat Capacity Heat capacity and specific heat capacity are often confused due to their similar names. Let's clarify some common misconceptions: Misconceptions about Heat Capacity Heat capacity is a property of a substance: This is a common error. o Reality: Heat capacity is a property of a specific object, not a substance. It depends on the object's mass and the substance it's made of. A larger object always has a higher heat capacity: This isn't necessarily true. o Reality: Heat capacity depends on both mass and the substance. A small object made of a material with a high specific heat capacity can have a higher heat capacity than a larger object made of a material with a low specific heat capacity. 11 | P a g e Misconceptions about Specific Heat Capacity Specific heat capacity is a measure of how hot an object gets: This is incorrect. o Reality: Specific heat capacity is a measure of how much heat energy is required to raise the temperature of one unit mass of a substance by one degree. All substances have the same specific heat capacity: This is untrue. o Reality: Different substances have different specific heat capacities. Water, for example, has a very high specific heat capacity compared to many other substances. 7.4. Thermal expansion Common Misconceptions About Thermal Expansion Thermal expansion, the tendency of matter to change its shape, area, and volume in response to a change in temperature, is a concept often misunderstood. Let's clarify some common misconceptions: Misconceptions about Thermal Expansion All substances expand when heated: While this is generally true for most substances, there are exceptions. Water, for example, contracts when heated from 0°C to 4°C. The expansion of a solid is uniform in all directions: This is not always accurate. Solids can expand differently in different directions, a phenomenon known as anisotropic expansion. The size of the expansion depends only on the temperature change: While temperature change is a significant factor, the material's coefficient of thermal expansion also plays a crucial role. Holes in objects shrink when heated: This is a common misconception. Actually, holes in objects expand when heated, just like the rest of the object. 12 | P a g e Thermal expansion is only significant for solids: While it's more noticeable in solids, all states of matter (solids, liquids, and gases) experience thermal expansion. 7.5. Change of Phase and Calorimetry Common Misconceptions About Change of Phase and Calorimetry Change of phase and calorimetry are often misunderstood concepts. Let's clarify some common misconceptions: Misconceptions about Change of Phase Temperature changes during phase change: Many believe that the temperature of a substance continues to rise or fall during a phase change. o Reality: The temperature remains constant during a phase change (e.g., melting, boiling). The energy added or removed is used to overcome intermolecular forces. All substances have the same latent heat values: Different substances require different amounts of energy to undergo phase changes. o Reality: Latent heat of fusion and vaporization are specific to each substance. Sublimation is rare: Sublimation (solid to gas) is often thought of as an unusual process. o Reality: Sublimation occurs in many substances, especially under low- pressure conditions. Dry ice is a common example. Misconceptions about Calorimetry Calorimetry only applies to temperature changes: Calorimetry is often associated solely with measuring temperature changes. 13 | P a g e o Reality: Calorimetry can also be used to determine latent heat values and specific heat capacities. Insulation is perfect: Calorimeters are assumed to be perfectly insulated, preventing any heat exchange with the surroundings. o Reality: There's always some heat loss, which is why experimental values often differ from theoretical ones. Specific heat capacity is constant: It's assumed that specific heat capacity remains the same regardless of temperature and pressure. o Reality: Specific heat capacity can vary with temperature and pressure, especially for gases. Unit 8 Electricity and Magnetism 8.1. Discharging a Body Common Misconceptions About Discharging a Body Disclaimer: If you're referring to the discharge of a human body, please consult with a healthcare professional. The information provided here is focused on the physical concept of discharging a body in the context of electricity. Misconceptions about Discharging a Body (Electrical Context) When we talk about "discharging a body" in a scientific context, we generally refer to removing excess electrical charge from an object. This is commonly encountered in electronics and electrostatic discharge (ESD) protection. Here are some common misconceptions: Touching a grounded object always discharges you completely: While grounding is effective in reducing static charge, it might not remove all the charge. 14 | P a g e Discharging only happens through a spark: While a spark is a visible sign of discharge, it's not the only way. A gradual discharge can also occur without a spark. Humidity prevents all static electricity: While humidity can reduce static electricity, it doesn't eliminate it completely. Anti-static sprays permanently remove charge: These sprays provide temporary relief and need to be reapplied. 8.2. Coulomb’s law of electrostatics Common Misconceptions About Coulomb's Law Coulomb's law, a fundamental principle in electrostatics, describes the force between two charged objects. Despite its simplicity, there are common misconceptions about it. Misconceptions about Coulomb's Law The force is always attractive: While opposite charges attract, like charges repel. The law accounts for both scenarios through the sign of the charges. The force depends only on the magnitude of the charges: The distance between the charges is equally important. The force decreases with the square of the distance. Coulomb's law applies only to point charges: While it's derived for point charges, it can be applied to spherical charge distributions with the center-to- center distance used. The force is a constant: The force is not constant; it changes with the distance between the charges and the magnitudes of the charges. The permittivity of free space is a constant related to the charges: The permittivity of free space, ε₀, is a property of the medium and is independent of the charges involved. 8.3. The electric field Common Misconceptions About the Electric Field 15 | P a g e The electric field, a fundamental concept in physics, is often misunderstood. Let's clarify some common misconceptions: Misconceptions About the Electric Field Electric field lines are paths of particles: This is a common misconception. Field lines represent the direction of the force on a positive test charge, not the actual path of particles. The electric field exists only around point charges: Electric fields exist around any charged object, regardless of its shape. Electric field strength is constant throughout space: The strength of an electric field varies with distance from the source charge. Electric field lines never cross: This is a fundamental rule. If field lines crossed, it would imply two different forces acting on a charge at that point, which is impossible. Electric field is a physical entity: The electric field is a mathematical construct used to describe the influence of a charge on its surroundings. It's not a physical substance. 8.4. Potential difference Common Misconceptions About Potential Difference Potential difference, often referred to as voltage, is a fundamental concept in electricity. However, there are several common misconceptions about it. Misconceptions about Potential Difference Voltage is a measure of the amount of charge: Voltage is actually a measure of the energy per unit charge. It's like the pressure in a water pipe, not the amount of water. Higher voltage means more current: While voltage can influence current, it's not the only factor. Resistance also plays a crucial role (Ohm's law: V = IR). 16 | P a g e Voltage is consumed in a circuit: Voltage is not consumed. It's converted into other forms of energy (like heat, light, or mechanical energy) as it drives current through a circuit. A battery is a source of charge: A battery is a source of energy, not charge. It provides the energy to move charges around a circuit. Ground is always at zero potential: This is a common reference point, but it's not always true. Ground can be assigned any potential value for convenience. 8.5. Ohm’s law Common Misconceptions About Ohm's Law Ohm's Law is a fundamental principle in electrical circuits, but it's often misunderstood. Let's clarify some common misconceptions: Misconceptions about Ohm's Law Ohm's Law applies to all materials: This is incorrect. Ohm's Law is valid only for ohmic materials, where the resistance remains constant regardless of the applied voltage. Many materials, especially non-metals, do not follow Ohm's Law. Increasing voltage always increases current proportionally: While this is true for ohmic materials according to Ohm's Law, it's not universally applicable. In non- ohmic materials or complex circuits, the relationship between voltage and current can be more complex. Resistance is constant in all conditions: Resistance can change with factors like temperature, light intensity (in photoresistors), and applied voltage (in non- linear components). Ohm's Law can explain all electrical phenomena: Ohm's Law is a simplified model that works well for many circuits, but it cannot explain complex behaviors like those found in semiconductors or AC circuits. 8.6. Voltmeter and ammeter connection in a circuit Common Misconceptions About Voltmeter and Ammeter Connections 17 | P a g e Voltmeters and ammeters are essential tools for measuring electrical quantities in circuits, but their correct connection is often misunderstood. Misconceptions Voltmeter connected in series: A voltmeter should always be connected in parallel with the component whose voltage is being measured. Connecting it in series would significantly alter the circuit's resistance. Ammeter connected in parallel: An ammeter should be connected in series with the component whose current is being measured. Connecting it in parallel would bypass the main circuit and could damage the meter. Voltmeter and ammeter polarity doesn't matter: Both meters have polarity. Connecting them incorrectly can either give incorrect readings or damage the meter. 8.7. Electric projects Common Misconceptions About Electrical Projects Misconceptions about electrical projects can lead to safety hazards and inefficient systems. Let's address some common ones: Misconceptions About DIY Electrical Work DIY is always cheaper: While it might seem cost-effective initially, mistakes can lead to expensive repairs or even fires. Electrical work requires specialized knowledge and permits. Basic electrical knowledge is enough: Even simple projects can involve complex wiring and safety considerations. Professional electricians have in-depth training. Older homes have simpler wiring: Older homes often have outdated and potentially hazardous wiring. Modern electrical systems are more complex and require expert handling. 18 | P a g e Misconceptions About Electrical Systems More outlets is always better: Overloading outlets can cause fires. It's essential to distribute electrical loads evenly. Grounding is optional: Grounding is a safety feature that protects people and equipment from electrical shocks. It's mandatory in most areas. Circuit breakers prevent all electrical problems: Circuit breakers protect against overloads and short circuits, but they won't prevent all electrical issues. Regular inspections are necessary. Misconceptions About Electrical Equipment All extension cords are safe: Overloading extension cords can cause overheating and fires. Use the correct gauge for the intended load. GFCIs protect against all electrical hazards: Ground Fault Circuit Interrupters (GFCIs) protect against shocks but not overloads. Older appliances are less efficient: Modern appliances are often more energy- efficient, but older models might still function safely. However, it's essential to inspect them regularly for wear and tear. 8.8. Magnetic field Common Misconceptions About Magnetic Fields The concept of magnetic fields can be quite intriguing, but also confusing. Here are some common misconceptions: Misconceptions about Magnetic Fields Magnets create magnetic fields: While magnets are often used to demonstrate magnetic fields, the fields are actually produced by moving electric charges. 19 | P a g e Magnetic field lines are physical entities: Magnetic field lines are a visual representation of the direction and strength of a magnetic field. They are not physical objects. Only iron is attracted to magnets: While iron is strongly attracted to magnets, other materials like nickel and cobalt are also magnetic. The Earth's magnetic field is constant: The Earth's magnetic field is constantly changing, and it has reversed polarity many times in the past. Magnetic force acts only on magnets: Magnetic force acts on any moving charged particle, not just magnets. 8.9. The Earth’s magnetic field and the compass Common Misconceptions About the Earth's Magnetic Field and the Compass The Earth's magnetic field and the compass are closely related, yet there are common misunderstandings about how they work together. Misconceptions The compass needle points directly to the geographic North Pole: This is incorrect. The compass needle points to the magnetic North Pole, which is different from the geographic North Pole. This difference is known as declination. The magnetic North Pole is a fixed point: The magnetic poles are constantly moving. This is why declination values change over time. Magnetic fields are only found on Earth: Magnetic fields are produced by moving electric charges and are found throughout the universe, not just on Earth. A compass will always work: Strong magnetic interference, like that from large electrical equipment or magnetic rocks, can disrupt a compass's readings. 8.10. Magnetic field of a current-carrying conductor Common Misconceptions About the Magnetic Field of a Current-Carrying Conductor 20 | P a g e The magnetic field produced by a current-carrying conductor is a fundamental concept in electromagnetism, yet it's often misunderstood. Let's clarify some common misconceptions: Misconceptions The magnetic field is static: The magnetic field around a current-carrying conductor is dynamic. If the current changes, the magnetic field also changes. The magnetic field only exists around straight conductors: While the magnetic field around a straight conductor is often used as a simple example, it can be generated by any shape of conductor, including coils and loops. The direction of the magnetic field is determined by the direction of the current: While the direction of the current does influence the direction of the magnetic field, the specific orientation is determined by the right-hand rule. The strength of the magnetic field is directly proportional to the distance from the conductor: The strength of the magnetic field decreases as the distance from the conductor increases, but the relationship is not linear. It follows an inverse relationship. 8.11. Magnetic force on a current-carrying wire Common Misconceptions About Magnetic Force on a Current-Carrying Wire The magnetic force acting on a current-carrying wire is a fundamental concept in electromagnetism, yet it often leads to misunderstandings. Let's clarify some common misconceptions: Misconceptions The force is always perpendicular to the wire: While it's true that the force is perpendicular to both the current and the magnetic field, the orientation of the wire relative to the field can affect the direction of the force. 21 | P a g e The force depends only on the current: The force also depends on the strength of the magnetic field, the length of the wire in the field, and the angle between the current and the magnetic field. A stronger current always results in a larger force: While increasing the current generally increases the force, other factors like the magnetic field strength and the orientation of the wire can influence the overall force. The force is always attractive: The direction of the force depends on the direction of the current and the magnetic field. It can be attractive, repulsive, or zero. 8.12. Electromagnetic induction Common Misconceptions About Electromagnetic Induction Electromagnetic induction is a fascinating phenomenon, but it's also a source of common misunderstandings. Let's clarify some of these: Misconceptions About Electromagnetic Induction A static magnetic field induces a current: A changing magnetic field is required to induce an electric current. A stationary magnet will not induce a current in a nearby conductor. The strength of the induced current is proportional to the magnetic field strength: While the strength of the induced current is related to the magnetic field, it's primarily determined by the rate of change of the magnetic field. Lenz's Law is optional: Lenz's Law is a fundamental principle that determines the direction of the induced current. It states that the induced current creates a magnetic field that opposes the change in the original magnetic field. Electromagnetic induction only works with coils of wire: While coils are often used to increase the induced voltage, induction can occur in any conductor exposed to a changing magnetic field. 22 | P a g e Induced current is always AC: While it's true that alternating current is commonly generated through electromagnetic induction, direct current can also be induced under specific conditions, such as in generators with commutators. 8.13. Transformers Common Misconceptions About Transformers Transformers are essential components in power systems, but there are several misconceptions about how they work. Let's clarify some of these: Misconceptions About Transformers Transformers create electricity: Transformers do not generate electricity; they change the voltage of an existing alternating current (AC) power supply. Transformers work with direct current (DC): Transformers rely on electromagnetic induction, which requires a changing magnetic field. DC current produces a constant magnetic field, so transformers cannot operate with it. Higher voltage means more power: While high voltage is used for efficient power transmission, it doesn't mean there's more power. Power (P) is equal to voltage (V) multiplied by current (I). Transformers maintain power, but they can change the voltage and current inversely proportional to each other. Transformers are 100% efficient: While modern transformers are highly efficient, they do incur losses due to factors like eddy currents, hysteresis, and copper losses. All transformers are the same: Transformers come in various types, sizes, and configurations, each designed for specific applications. 23 | P a g e