Miscellaneous Techniques (Without Instrumentation).pdf
Document Details
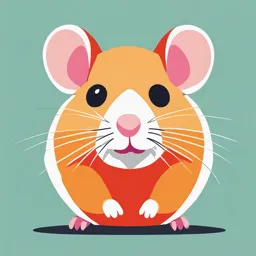
Uploaded by BestSellingDobro2830
Mapúa University
Tags
Full Transcript
NOTE: This is merely a general comparison of some spectroscopic methods, thermoanalytical methods, radiochemical methods, light scattering techniques, and separation techniques. NO INSTRUMENTATION NO SUCH TYPES (except for MS, NMR) Comparison between EXAFS, N...
NOTE: This is merely a general comparison of some spectroscopic methods, thermoanalytical methods, radiochemical methods, light scattering techniques, and separation techniques. NO INSTRUMENTATION NO SUCH TYPES (except for MS, NMR) Comparison between EXAFS, NMR, and Raman Spectroscopy Extended X-ray absorption fine structure (EXAFS), NMR spectroscopy, and Raman spectroscopy are different analytical techniques used to study the structure and properties of materials. Each technique is based on distinct physical principles and is useful for different types of information. 1. Extended X-ray Absorption Fine Structure (EXAFS) Principle: EXAFS involves the absorption of X-rays by atoms in a sample. When an X-ray photon has enough energy, it can eject a core electron from an atom. The resulting photoelectron wave is scattered by neighboring atoms, creating interference patterns (fine structure) in the X-ray absorption spectrum. This interference provides information about the local atomic environment. Information Provided: o Local atomic structure, bond lengths, coordination numbers. o Type of neighboring atoms (elemental identification). o Information on disordered or amorphous materials. Typical Applications: o Determining the local chemical environment of specific elements in a complex system. o Investigating metal complexes, catalysts, and amorphous solids. 2. Nuclear Magnetic Resonance (NMR) Spectroscopy Principle: NMR is based on the magnetic properties of certain atomic nuclei (like hydrogen- 1, carbon-13, etc.). When placed in a magnetic field, these nuclei absorb electromagnetic radiation at a frequency specific to their magnetic environment. By analyzing the resonance frequency, one can infer details about the chemical environment of the atoms. Information Provided: o Chemical structure, molecular dynamics, and conformation. o Information on how atoms are bonded and their spatial arrangement in organic or biomolecules. o Molecular interactions and dynamic processes. Typical Applications: o Organic chemistry (determining the structure of small molecules). o Biochemistry (studying proteins, nucleic acids). o Medical imaging (MRI is an application of NMR). 3. Raman Spectroscopy Principle: Raman spectroscopy is based on inelastic scattering of light (usually from a laser). When light interacts with the molecules, most of it is scattered elastically (Rayleigh scattering), but a small portion is scattered inelastically, leading to a shift in energy. This shift corresponds to vibrational, rotational, or other low-frequency modes in the molecules. Information Provided: o Molecular vibrations and rotational information. o Chemical composition, structural information, and molecular interactions. o Non-destructive and applicable to both crystalline and amorphous materials. Typical Applications: o Identifying molecular structures, particularly in inorganic and organic materials. o Studying molecular vibrations and phonons in materials. o Analyzing biological tissues and detecting contaminants. Key Differences: Feature EXAFS NMR Spectroscopy Raman Spectroscopy Energy Source X-rays Radio waves Visible or near-infrared light Target Local atomic Magnetic nuclei Molecular vibrations environment (usually (commonly H, C, N, P) metals) Type of Local atomic structure Molecular structure Vibrational modes, Information and dynamics chemical composition Sample Type Solid, liquid, or Liquid (typically), solid Solids, liquids, gases amorphous materials Common Use Coordination chemistry, Organic molecules, Chemistry, biology, and materials science biomolecules materials science Each technique has unique strengths depending on the type of material being analyzed and the kind of structural or compositional information required. Among the three techniques—EXAFS, NMR spectroscopy, and Raman spectroscopy—their potential for being destructive or non-destructive depends on how the sample interacts with the energy source and whether the sample remains intact after analysis. 1. Extended X-ray Absorption Fine Structure (EXAFS): Non-destructive: In most cases, EXAFS is considered non-destructive, as the sample remains chemically and physically intact after analysis. However, very intense X-rays can cause damage to delicate or sensitive materials (such as biological samples or polymers), leading to degradation over time. For metals and robust materials, EXAFS is typically non- destructive. 2. Nuclear Magnetic Resonance (NMR) Spectroscopy: Non-destructive: NMR spectroscopy is generally non-destructive. It does not alter the chemical composition or structure of the sample because it only uses low-energy radio waves in the presence of a magnetic field. Samples can usually be recovered and reused after analysis. However, samples dissolved in specific solvents for liquid-state NMR might undergo some changes due to solvent effects. 3. Raman Spectroscopy: Non-destructive: Raman spectroscopy is typically non-destructive, especially when using low-power lasers. It provides structural information without altering or damaging the sample. However, high-power lasers or prolonged exposure to the laser can cause heating, degradation, or even burning of certain sensitive materials, which may result in partial damage to the sample. Summary: Technique Destructive or Non-destructive? EXAFS Non-destructive (but high X-ray doses could damage delicate samples) NMR Non-destructive (sample is recoverable) Raman Non-destructive (low-power lasers); may be slightly destructive with high-power lasers on sensitive samples In general, all three techniques are non-destructive for most applications, but care should be taken when analyzing sensitive or delicate materials, especially under high-energy conditions (EXAFS and Raman). Thermogravimetric analysis (TGA), differential thermal analysis (DTA), and differential scanning calorimetry (DSC) are thermal analysis techniques used to study the properties of materials as they change with temperature. They are primarily used to evaluate thermal stability, phase transitions, and material composition, but each technique provides different types of information. Comparison between TGA, DTA, and DSC 1. Thermogravimetric Analysis (TGA) Principle: TGA measures the change in mass of a sample as it is heated, cooled, or held at a constant temperature over time. The mass changes can be due to decomposition, oxidation, reduction, or the loss of volatile components. Information Provided: o Mass loss or mass gain with temperature. o Thermal stability of materials. o Decomposition temperatures, moisture content, or oxidation processes. o Composition of materials (e.g., organic/inorganic content). Typical Applications: o Studying polymers, composites, and organic materials. o Determining moisture, ash content, or the amount of volatile compounds in a material. o Analyzing thermal degradation of materials. 2. Differential Thermal Analysis (DTA) Principle: DTA measures the temperature difference between a sample and an inert reference as both are heated or cooled. The temperature difference reflects the occurrence of endothermic (heat absorption) or exothermic (heat release) events in the sample. Information Provided: o Detection of phase transitions (melting, crystallization). o Endothermic and exothermic reactions such as dehydration, decomposition, or oxidation. o No mass change information (unlike TGA). Typical Applications: o Identifying melting points, crystallization, and other thermal events. o Characterizing phase changes in metals, ceramics, and polymers. o Analyzing reactions like oxidation or combustion. 3. Differential Scanning Calorimetry (DSC) Principle: DSC measures the heat flow required to maintain the sample and reference at the same temperature as they are heated or cooled. It is similar to DTA but provides more quantitative data on the amount of heat absorbed or released during thermal events. Information Provided: o Heat flow related to phase transitions (melting, glass transitions, crystallization). o Quantitative data on enthalpy changes (ΔH) for thermal events. o Thermal stability and specific heat capacity. o Both endothermic and exothermic processes. Typical Applications: o Studying melting points, crystallization, and glass transitions in polymers and pharmaceuticals. o Analyzing curing reactions of resins and thermosetting polymers. o Determining the heat capacity and phase transition enthalpies of materials. Key Differences: Feature TGA DTA DSC Measured Mass change vs. Temperature difference Heat flow vs. Property temperature between sample and temperature reference Type of Mass loss/gain (e.g., Thermal events Thermal events with Information decomposition, (endothermic/exothermic) quantitative heat evaporation) flow data Sample Decomposition, Phase transitions, reactions, Phase transitions, Information oxidation, thermal thermal effects heat capacity, stability enthalpy changes Quantitative or Quantitative (mass Qualitative (temperature Quantitative (heat Qualitative change) changes) flow and enthalpy) Common Use Thermal stability, Identifying phase transitions Measuring enthalpy composition changes, specific heat, glass transitions Mass Change Yes No No Information Heat Flow No Indirect (temperature Yes (direct heat flow Measurement difference) measurement) Summary: TGA: Measures mass loss or gain, useful for studying decomposition, oxidation, and thermal stability. DTA: Measures temperature differences to detect endothermic or exothermic processes, useful for identifying phase transitions. DSC: Measures heat flow to quantify the heat absorbed or released during thermal transitions, providing detailed thermal analysis with enthalpy data. Each technique serves different purposes and is chosen based on the type of information required about a material's thermal behavior. The destructive or non-destructive nature of Thermogravimetric Analysis (TGA), Differential Thermal Analysis (DTA), and Differential Scanning Calorimetry (DSC) depends on how the sample interacts with the applied heat during analysis. Here's a breakdown: 1. Thermogravimetric Analysis (TGA) Destructive: TGA is typically destructive, as it measures mass changes due to processes like decomposition, oxidation, or evaporation. These processes often involve irreversible chemical reactions, meaning the sample is consumed or altered during the analysis. For example, polymers might decompose, or organic materials may burn off, making recovery of the original sample impossible. 2. Differential Thermal Analysis (DTA) Destructive/Non-destructive: DTA can be destructive or non-destructive, depending on the type of material being analyzed and the thermal events it undergoes. If the sample undergoes physical changes (e.g., phase transitions like melting or crystallization), the analysis might be non-destructive. However, if chemical changes (e.g., decomposition or combustion) occur, the sample is usually destroyed. The sample often undergoes more drastic changes in DTA when studying reactions such as oxidation or decomposition. 3. Differential Scanning Calorimetry (DSC) Non-destructive: DSC is generally non-destructive, as it primarily measures heat flow associated with phase transitions like melting, crystallization, or glass transitions without altering the sample’s chemical composition. Most samples can be recovered intact after analysis, as DSC typically does not lead to chemical degradation. However, if DSC is used to study decomposition reactions or thermal curing processes, the sample may be altered, leading to destructive results. Summary: Technique Destructive or Non-destructive? TGA Destructive (due to decomposition, oxidation, mass loss) DTA Destructive or Non-destructive (depends on whether the process involves physical or chemical changes) DSC Non-destructive (for most phase transitions); Destructive if chemical changes or decomposition occur In general, TGA is destructive due to the nature of mass loss, while DSC is typically non- destructive, especially for phase transitions like melting and crystallization. DTA can be either, depending on the specific thermal event being analyzed. Comparison between NAA, Isotope Dilution, and Radioimmunoassay Neutron Activation Analysis (NAA), Isotope Dilution, and Radioimmunoassay (RIA) are analytical techniques used to quantify elements, isotopes, or specific biological molecules. These methods differ significantly in their principles, applications, and the type of information they provide. 1. Neutron Activation Analysis (NAA): Principle: NAA is based on the interaction between neutrons and atomic nuclei. When a sample is exposed to neutrons, some nuclei capture these neutrons and become radioactive isotopes. The radioactive decay of these isotopes emits gamma rays, which can be detected and measured. The energy and intensity of the emitted gamma rays are unique to each element and can be used to determine the elemental composition of the sample. Information Provided: o Quantitative elemental analysis without destroying the sample. o Can detect elements in trace amounts, even in complex matrices. o Particularly useful for determining the presence and concentration of trace elements and heavy metals. Typical Applications: o Geological and environmental studies (e.g., trace element analysis of soils, rocks). o Forensic analysis (e.g., analysis of trace elements in gunshot residues). o Archaeology (e.g., composition of ancient artifacts). Destructive or Non-destructive: Usually non-destructive, as the sample remains intact after the analysis. 2. Isotope Dilution: Principle: Isotope dilution is a quantitative technique in which a known amount of an isotopically enriched version of the analyte (the “spike”) is added to the sample. The ratio of the natural isotope to the spiked isotope is then measured using a mass spectrometer. This ratio allows for the accurate determination of the concentration of the analyte in the original sample. Information Provided: o Quantitative determination of element or compound concentrations with very high accuracy and precision. o Especially useful for compounds or elements that are difficult to quantify due to matrix effects. Typical Applications: o Environmental monitoring (e.g., measuring pollutants or contaminants). o Clinical chemistry (e.g., determining drug levels or nutrient concentrations). o Analytical chemistry (e.g., quantifying metals, organic compounds). Destructive or Non-destructive: Generally destructive, since the sample is often consumed during the preparation and analysis, especially when measuring isotopic ratios. 3. Radioimmunoassay (RIA): Principle: RIA is a highly sensitive technique used to measure concentrations of biological molecules (like hormones, proteins, or drugs) in a sample. It is based on the use of a radioactive-labeled version of the analyte (antigen) and its specific antibody. The sample is mixed with the radiolabeled antigen and the antibody, leading to competition between the labeled and unlabeled (from the sample) antigen for antibody binding. The amount of radioactivity in the bound antigen-antibody complex is inversely proportional to the concentration of the analyte in the sample. Information Provided: o Quantitative and highly sensitive detection of biological molecules in small concentrations (e.g., nanograms or picograms). o Primarily used to detect biomolecules such as hormones, proteins, drugs, or antigens. Typical Applications: o Clinical diagnostics (e.g., measuring hormone levels like insulin, thyroid hormones). o Pharmaceutical studies (e.g., monitoring drug levels in blood). o Research (e.g., studying immune responses and disease biomarkers). Destructive or Non-destructive: Destructive, as the sample is consumed during the immunoassay process. Key Differences: Feature Neutron Activation Isotope Dilution Radioimmunoassay (RIA) Analysis (NAA) Principle Neutron Spiking sample with Competition between bombardment and isotopically enriched labeled and unlabeled gamma-ray analyte and antigen for binding to a detection from measuring isotopic specific antibody radioactive isotopes ratios Analyte Elements (metals, Elements or specific Biological molecules trace elements) molecules (e.g., (hormones, proteins, drugs, metals) antigens) Application Trace element Quantitative Quantifying biomolecules analysis in geology, determination of in clinical and archaeology, and chemicals and pharmaceutical research forensics elements in complex samples Sensitivity Very sensitive for Highly accurate and Extremely sensitive for low trace elements precise for isotope concentrations of ratios biological molecules Destructive/Non- Non-destructive Destructive Destructive destructive Typical Gamma-ray Mass spectrometry Radioactivity detection Measurement spectroscopy Technique Summary: NAA is ideal for non-destructive analysis of trace elements in a variety of materials, particularly in fields like geology and forensics. Isotope Dilution is a highly accurate and precise destructive technique used for quantifying elements or compounds, especially in complex mixtures, and is often used with mass spectrometry. Radioimmunoassay is a destructive method used primarily in biological and clinical applications for measuring very small concentrations of hormones, proteins, or other antigens using radioisotopes. Here's a breakdown of the destructive or non-destructive nature of Neutron Activation Analysis (NAA), Isotope Dilution, and Radioimmunoassay (RIA): 1. Neutron Activation Analysis (NAA) Non-destructive: NAA is typically non-destructive. The sample remains chemically and physically intact during analysis, as the process involves exposing the sample to neutrons and measuring gamma radiation emitted by the activated isotopes without altering the sample itself. This makes NAA useful for analyzing precious or irreplaceable materials, such as archaeological artifacts and geological samples. 2. Isotope Dilution Destructive: Isotope dilution is generally destructive, as the sample is typically consumed during preparation and analysis. The method requires adding an isotopically enriched "spike" to the sample and measuring the isotopic ratio using techniques like mass spectrometry, which often involves dissolving or chemically processing the sample. This process irreversibly alters the sample, so it cannot be recovered afterward. 3. Radioimmunoassay (RIA) Destructive: RIA is destructive, as the sample is consumed in the process of binding antigens to antibodies and the subsequent radioactive measurement. The sample cannot be recovered once it has been exposed to the radiolabeled antigens and undergone the competitive binding reactions. Summary: Technique Destructive or Non-destructive? NAA Non-destructive (sample remains intact) Isotope Dilution Destructive (sample is consumed) Radioimmunoassay (RIA) Destructive (sample is consumed) In short, NAA is non-destructive, while isotope dilution and RIA are destructive techniques. Comparison between Nephelometry, and Turbidimetry Nephelometry and turbidimetry are both analytical techniques used to measure the scattering of light by particles in a suspension. They are often used to analyze colloidal systems or to quantify concentrations of particles (such as proteins, bacteria, or other suspended materials) in a solution. Despite their similarities, they differ in how they measure the light scattering and the type of information they provide. 1. Nephelometry Principle: Nephelometry measures the intensity of light scattered at an angle (usually 90°) to the direction of the incident light beam. This scattered light is detected and correlated to the concentration of particles in the solution. The more particles there are, the more light is scattered. Information Provided: o Quantifies the scattered light, which is directly related to the number and size of particles in the suspension. o More sensitive to small particles and low concentrations, especially in clear suspensions. Typical Applications: o Measurement of antigen-antibody complexes in immunoassays. o Monitoring protein concentrations in biological samples. o Turbidity in water (e.g., for environmental testing). Measurement: The detector is positioned at an angle (usually 90°) to the incident light source, capturing the light scattered by the particles. 2. Turbidimetry Principle: Turbidimetry measures the reduction in intensity (attenuation) of the light as it passes straight through the suspension. Particles in the suspension scatter and absorb the light, reducing the intensity that reaches the detector. Information Provided: o Quantifies the loss of light intensity due to scattering and absorption by particles. o More useful for higher concentrations of particles, where the solution becomes visibly turbid (cloudy). Typical Applications: o Monitoring bacterial growth in liquid media. o Measuring the concentration of suspended solids in wastewater. o Testing turbidity in various liquid samples (e.g., water quality testing). Measurement: The detector is positioned directly in line with the light source, measuring the amount of light that passes through the sample. Key Differences: Feature Nephelometry Turbidimetry Measurement Measures scattered light (typically Measures attenuated light (decrease in Focus at 90° to the incident beam) transmitted light through the sample) Detector At an angle (usually 90°) to the In line with the incident light (straight Position incident light through the sample) Sensitivity More sensitive to low particle More suitable for higher concentrations and small concentrations and larger particles particles Typical Protein quantification, Bacterial growth, wastewater analysis, Application immunoassays, low-concentration high-concentration suspensions suspensions Ideal for Clear or slightly turbid solutions Highly turbid solutions with dense with low particle content suspensions Effectiveness Better for detecting light Better for detecting the loss of scattering by small particles transmitted light due to large particle concentrations Summary: Nephelometry measures scattered light, making it more sensitive for detecting smaller particles or low concentrations in clear solutions. It is often used in immunoassays and for quantifying proteins or antigens. Turbidimetry measures transmitted light, focusing on the reduction in light intensity caused by scattering and absorption in turbid (cloudy) solutions. It is ideal for analyzing more concentrated suspensions, such as bacterial cultures or wastewater samples. Both techniques are valuable for quantifying suspended particles, but they excel in different concentration ranges and types of solutions. Both nephelometry and turbidimetry are typically non-destructive techniques. Here's why: 1. Nephelometry: Non-destructive: Nephelometry measures the intensity of scattered light from particles in suspension without altering or damaging the sample. The sample remains intact and can be recovered after analysis. Therefore, it is a non-destructive technique. 2. Turbidimetry: Non-destructive: Turbidimetry measures the reduction in light intensity passing through a sample, but it also does not chemically or physically alter the sample. The suspension remains unchanged after the analysis, so this method is also considered non-destructive. Summary: Technique Destructive or Non-destructive? Nephelometry Non-destructive Turbidimetry Non-destructive Both nephelometry and turbidimetry are non-destructive methods, meaning the samples can typically be reused after the analysis. Comparison between FIA, and Electrophoresis Flow Injection Analysis (FIA) and Electrophoresis are two distinct analytical techniques used for separation, detection, and analysis of chemical or biological substances. They differ in their underlying principles, applications, and the type of information they provide. Here's a detailed comparison: 1. Flow Injection Analysis (FIA) Principle: FIA is a continuous flow technique in which a sample is injected into a carrier stream (a flowing liquid), and the resulting mixture is passed through a detector. The interaction of the sample with reagents in the flow stream allows for chemical reactions, producing measurable signals (like absorbance, fluorescence, or electrochemical signals). Key Features: o Automation and Speed: FIA is highly automated and allows for rapid analysis of multiple samples in a short period. o Reagent Mixing: The injected sample mixes with reagents as it flows, allowing reactions to take place in a controlled environment. o Measurement: The reaction products are detected as the flow moves through a detector, providing real-time monitoring. Information Provided: o Quantitative and sometimes qualitative information about chemical species in a sample (e.g., concentration of analytes). Typical Applications: o Environmental analysis (e.g., determining nitrate, phosphate in water). o Clinical chemistry (e.g., glucose or ion concentration in blood). o Food and pharmaceutical analysis (e.g., vitamins, drugs). Separation Process: FIA is not primarily a separation technique but rather a method for rapid chemical analysis. Speed: FIA is known for its fast throughput, allowing analysis of multiple samples in quick succession. 2. Electrophoresis Principle: Electrophoresis is a separation technique based on the movement of charged particles through a medium (usually a gel or capillary) under the influence of an electric field. The particles move at different rates depending on their charge, size, and shape. Key Features: o Separation Based on Charge and Size: Molecules like DNA, proteins, or small ions are separated as they migrate through a medium, with smaller or more highly charged particles moving faster. o Electric Field: The technique requires applying an electric field, which forces the particles to move through the medium. o Medium: Gel electrophoresis (e.g., agarose or polyacrylamide gels) or capillary electrophoresis is commonly used as the separation medium. Information Provided: o Primarily used for separating and identifying macromolecules (e.g., DNA, RNA, proteins) or ions based on their charge-to-mass ratio. Typical Applications: o DNA/RNA analysis (e.g., genetic fingerprinting, DNA sequencing). o Protein analysis (e.g., protein purity, protein size). o Clinical diagnostics (e.g., hemoglobin electrophoresis for sickle cell anemia). Separation Process: Electrophoresis is mainly a separation technique, distinguishing particles or molecules based on their migration rates under an electric field. Speed: Electrophoresis can take longer than FIA, especially in the case of gel electrophoresis, which may require hours for separation. Key Differences: Feature Flow Injection Analysis (FIA) Electrophoresis Principle Continuous flow of a sample through Movement of charged particles in an a carrier stream with reagents and electric field for separation detection Main Purpose Rapid chemical analysis of specific Separation of particles (e.g., DNA, analytes proteins) based on charge and size Separation Not a separation technique; focuses Primarily a separation technique on reaction and detection Detection Based on the reaction of analytes with Based on particle migration and reagents in the flow stream subsequent detection (e.g., staining) Sample Type Typically used for chemical analysis Often used for biological of liquids macromolecules (e.g., DNA, proteins) and ions Typical Environmental, food, clinical Genetic analysis, protein analysis, Applications chemistry (e.g., nutrient or drug clinical diagnostics concentration) Analysis Speed Rapid, high throughput Slower, especially for complex samples (e.g., DNA gels) Automation Highly automated Can be automated (e.g., capillary electrophoresis) but less so than FIA Separation Carrier stream (liquid) Gel (e.g., agarose or polyacrylamide) Medium or capillary Use of Requires reagents for chemical Typically doesn't use reagents except Reagents reactions for visualization (e.g., staining) Summary: Flow Injection Analysis (FIA) is a rapid, automated method used for chemical analysis. It does not separate molecules but instead allows for real-time detection based on chemical reactions in a flowing stream. Electrophoresis is a separation technique that sorts molecules (like DNA or proteins) based on their charge and size using an electric field. It is commonly used in biological and genetic analysis. While FIA focuses on analyzing the concentration of substances through reactions in a flow system, electrophoresis is designed to separate and then identify different charged particles or biomolecules based on their migration rates in an electric field. Here's an analysis of the destructive or non-destructive nature of Flow Injection Analysis (FIA) and Electrophoresis: 1. Flow Injection Analysis (FIA) Non-destructive (usually): FIA is typically non-destructive. The sample is injected into a flowing carrier stream, where it reacts with reagents, but in many cases, the sample remains largely intact after analysis. This makes FIA non-destructive for most chemical analysis purposes. However, depending on the nature of the chemical reactions taking place (e.g., if the analyte is altered during the reaction), the sample might not be fully recoverable. 2. Electrophoresis Destructive: Electrophoresis is generally considered destructive. During the separation process, the sample (e.g., DNA, RNA, or proteins) migrates through a gel or capillary under the influence of an electric field. While the molecules themselves are not chemically destroyed, the sample is often altered (e.g., denatured) or bound to dyes/stains for visualization, making it difficult to recover the original sample in its unaltered form. Furthermore, some techniques like SDS-PAGE denature proteins, making recovery of the original functional form impossible. Summary: Technique Destructive or Non-destructive? Flow Injection Analysis (FIA) Non-destructive (in most cases) Electrophoresis Destructive (sample is altered or difficult to recover) In short, FIA is generally non-destructive, while electrophoresis is typically destructive, particularly when denaturing agents or staining are used. MS and types of MS Mass spectrometry (MS) is an analytical technique used to measure the mass-to-charge ratio (m/z) of ions. It helps identify the amount and type of chemicals present in a sample by generating charged particles (ions) from the sample and then separating them based on their mass and charge. The technique provides detailed information about the molecular composition, structure, and sometimes the quantity of the components within a sample. Key Components of Mass Spectrometry: 1. Ion Source: o The sample is introduced and converted into ions, typically by ionization methods such as electron ionization (EI), electrospray ionization (ESI), or matrix-assisted laser desorption ionization (MALDI). 2. Mass Analyzer: o The ions are separated based on their mass-to-charge ratio (m/z). Different mass analyzers include time-of-flight (TOF), quadrupole, ion trap, Orbitrap, and magnetic sector. Each analyzer has specific advantages in resolution, accuracy, or speed. 3. Detector: o The separated ions are detected, and the signal is converted into a mass spectrum, which shows the relative abundance of ions at different mass-to-charge ratios. This spectrum is used to deduce the molecular weight and sometimes the structure of the analyte. How Mass Spectrometry Works: 1. Ionization: The sample is introduced into the mass spectrometer, where it is ionized to form charged particles. This can be done through methods like: o Electron Ionization (EI): Common for small molecules. o Electrospray Ionization (ESI): Useful for large biomolecules like proteins and peptides. o MALDI: Often used for large and fragile molecules like biomolecules. 2. Mass Analysis: The ions are separated based on their mass-to-charge ratio in a mass analyzer. The time or path taken by the ions to reach the detector varies depending on their mass, allowing them to be separated and detected. 3. Detection: A detector measures the ions, producing a mass spectrum that shows the intensity of ions (corresponding to their abundance) versus their mass-to-charge ratio. Applications of Mass Spectrometry: Chemical Analysis: Identification of unknown compounds, determination of molecular weights, and elucidation of molecular structures. Proteomics: Identification and quantification of proteins and peptides in biological samples. Environmental Analysis: Detecting pollutants and contaminants in air, water, and soil. Pharmaceuticals: Quality control and drug discovery by analyzing drugs and metabolites. Isotope Ratio Analysis: Determining isotopic compositions for applications in geology, archaeology, and forensics. Types of Information Provided: Molecular Weight: The molecular weight of an analyte can be determined based on the mass-to-charge ratio. Molecular Structure: Fragmentation patterns in tandem MS (MS/MS) provide information about the structure of molecules. Quantitative Data: The intensity of signals in the mass spectrum can be used to quantify the amount of an analyte in a sample. Advantages of Mass Spectrometry: Sensitivity: MS is extremely sensitive, capable of detecting trace amounts of substances. Specificity: It can identify compounds based on their unique mass spectra. Versatility: Applicable to a wide variety of fields, from chemistry to biology and environmental science. Summary: Mass spectrometry is a versatile and powerful technique for identifying the composition and structure of molecules by analyzing their mass-to-charge ratios. It has broad applications across science, including chemistry, biology, environmental studies, and medicine. Types of MS Mass spectrometry (MS) is a powerful analytical technique used to measure the mass-to-charge ratio (m/z) of ions. Different types of mass spectrometry are designed to optimize for different analytical goals, such as resolution, sensitivity, and speed, or to handle different types of samples (e.g., small molecules, large biomolecules). The main types of mass spectrometry vary in the way ions are generated, separated, or detected. Below are key types of mass spectrometry: 1. Time-of-Flight Mass Spectrometry (TOF-MS) Principle: In TOF-MS, ions are accelerated by an electric field into a flight tube. The time it takes for ions to reach the detector depends on their mass-to-charge ratio (m/z), with lighter ions reaching the detector faster than heavier ions. Key Features: o Provides high resolution and broad mass range. o Useful for analyzing large biomolecules like proteins and peptides. Typical Applications: o Proteomics (protein identification). o Biomolecule analysis. Strengths: o Can measure a wide range of masses in a single run. o High sensitivity and good resolution. Limitations: o Lower mass accuracy compared to some other techniques. 2. Quadrupole Mass Spectrometry (QMS) Principle: In QMS, ions are filtered based on their m/z as they travel through a set of four parallel rods (quadrupole). By varying the electric fields applied to the quadrupole, only ions of a specific m/z reach the detector. Key Features: o Common in many types of instruments, such as triple quadrupole MS for tandem MS experiments. o Suitable for routine analysis and quantitation. Typical Applications: o Environmental analysis. o Quantitative analysis of small molecules (e.g., drugs, pesticides). Strengths: o Good for quantitation and targeted analysis. o Easy to couple with other techniques (e.g., gas chromatography, liquid chromatography). Limitations: o Lower resolution and sensitivity compared to other techniques like TOF or Orbitrap. 3. Ion Trap Mass Spectrometry (IT-MS) Principle: Ions are trapped in an oscillating electric field inside a three-dimensional "trap" or a linear trap. By changing the field, ions of specific m/z are selectively ejected and detected. Key Features: o Allows for tandem mass spectrometry (MS/MS) experiments within the same trap, enabling fragmentation of ions for structure elucidation. Typical Applications: o Structural analysis of small molecules. o Peptide and protein sequencing. Strengths: o MS/MS capability in a single instrument. o Useful for small samples and complex mixtures. Limitations: o Lower resolution compared to TOF and Orbitrap. o Limited dynamic range and sensitivity. 4. Orbitrap Mass Spectrometry Principle: Ions are trapped in an electrostatic field between a central spindle-shaped electrode and an outer barrel-shaped electrode. The ions move in an orbital motion, and their frequencies are used to determine the m/z. Key Features: o Known for high mass accuracy and high resolution. Typical Applications: o Proteomics, metabolomics. o Complex mixture analysis. Strengths: o Extremely high resolution and mass accuracy. o Ideal for identifying unknown compounds in complex samples. Limitations: o Higher cost and complexity. o May have longer analysis times compared to other types. 5. Fourier-Transform Ion Cyclotron Resonance Mass Spectrometry (FT-ICR-MS) Principle: Ions are trapped in a magnetic field and their motion generates a cyclotron frequency. The frequency is detected, and the m/z is calculated using Fourier-transform (FT) algorithms. Key Features: o Provides ultra-high resolution and mass accuracy. o Often used in high-end applications requiring extreme precision. Typical Applications: o Proteomics, lipidomics. o Complex molecular structures in small molecules. Strengths: o Exceptional resolution and accuracy, higher than Orbitrap. o Useful for exact mass determination of complex molecules. Limitations: o Expensive and complex instrumentation. o Longer analysis times and lower throughput. 6. Magnetic Sector Mass Spectrometry Principle: Ions are separated by their mass-to-charge ratio as they pass through a magnetic field. The magnetic field bends the ion path, with heavier ions being bent less and lighter ions more. Key Features: o Provides high mass accuracy and resolution. o Useful for both small molecule analysis and isotopic ratio determination. Typical Applications: o Isotope ratio mass spectrometry (IRMS) for geological or environmental studies. Strengths: o Excellent for high-precision isotope analysis. Limitations: o Expensive, and generally less common for routine laboratory use. 7. MALDI-TOF (Matrix-Assisted Laser Desorption Ionization Time-of-Flight) Principle: A laser is used to ionize the sample embedded in a matrix, causing desorption of the sample into the gas phase. The ions are then analyzed by a TOF mass analyzer. Key Features: o Ideal for analyzing large biomolecules such as proteins and peptides. Typical Applications: o Protein identification and characterization. o Analysis of large biomolecules like peptides, DNA, and synthetic polymers. Strengths: o Soft ionization technique that preserves large, fragile molecules. o Can analyze high molecular weight species (e.g., proteins). Limitations: o Not suitable for small, volatile molecules. o Requires the sample to be embedded in a matrix, which can limit its versatility. 8. Electrospray Ionization Mass Spectrometry (ESI-MS) Principle: In ESI-MS, the sample is ionized by passing it through a fine needle to create charged droplets in the presence of a strong electric field. The solvent evaporates, leaving behind charged ions, which are then analyzed. Key Features: o Produces multiply charged ions, which allows even large biomolecules to be analyzed by mass spectrometers with limited mass ranges. Typical Applications: o Analysis of proteins, peptides, nucleotides, and other macromolecules. Strengths: o Very soft ionization technique, preserving the native structure of fragile molecules. o Can be coupled with LC-MS for high-throughput analysis of complex mixtures. Limitations: o Sensitive to ion suppression, especially in complex mixtures. Comparison Table: Mass Principle Key Strengths Typical Limitations Spectrometry Applications Type TOF-MS Ions separated High resolution, Biomolecule Lower mass based on flight broad mass analysis (e.g., accuracy than time range proteins) some other types Quadrupole Ions filtered by Good for Environmental Lower resolution MS electric field quantitation, analysis, clinical and sensitivity through rods easy to couple chemistry with LC/GC Ion Trap MS Ions trapped in MS/MS Structural analysis Lower resolution, an oscillating capability, of molecules limited sensitivity field structural analysis Orbitrap MS Ions orbit High mass Proteomics, Higher cost, around a accuracy and metabolomics longer analysis central resolution times electrode FT-ICR-MS Ions trapped in Ultra-high Exact mass Expensive, a magnetic field resolution and determination, complex mass accuracy complex structures instrumentation Magnetic Ions bent in a High mass Isotope ratio Expensive, less Sector MS magnetic field accuracy, analysis common for isotope analysis routine use MALDI-TOF Laser ionization Good for large Protein Not suitable for with matrix- molecules like identification, small molecules assisted proteins biomolecules desorption ESI-MS Ions generated Soft ionization, Proteins, peptides, Sensitive to ion by electrospray useful for large macromolecules suppression in an electric biomolecules field Summary: TOF-MS and MALDI-TOF are ideal for analyzing large biomolecules. Quadrupole MS and Ion Trap MS are versatile and often used for routine analysis and MS/MS experiments. Orbitrap MS and FT-ICR-MS provide extremely high mass accuracy and resolution, making them ideal for complex or high-precision applications. Magnetic Sector MS is excellent for isotope ratio analysis. ESI-MS is widely used for biomolecule analysis due to its soft ionization technique that preserves molecular integrity Non-Destructive Techniques: Electrospray Ionization Mass Spectrometry (ESI-MS): o Non-destructive: ESI is a soft ionization technique that generates ions from liquid samples without fragmenting large molecules like proteins or peptides. The sample typically remains intact in terms of its molecular structure, though some analyte may be consumed in the process. Matrix-Assisted Laser Desorption Ionization Time-of-Flight (MALDI-TOF): o Non-destructive (in some cases): MALDI is another soft ionization technique that gently ionizes large molecules such as proteins or peptides without causing significant fragmentation. Some material may be consumed, but the overall molecular structure is preserved. Destructive Techniques: Time-of-Flight Mass Spectrometry (TOF-MS): o Destructive: TOF-MS can be destructive depending on how the ions are generated (e.g., electron ionization, which often fragments molecules). It breaks the molecules into ions, and thus the original sample is typically destroyed. Quadrupole Mass Spectrometry (QMS): o Destructive: QMS often uses ionization techniques like electron ionization (EI), which can fragment the molecules. As a result, the sample may not remain intact, making it destructive. Ion Trap Mass Spectrometry (IT-MS): o Destructive: IT-MS generally involves ionization techniques that break down the molecules, and in the MS/MS mode, selected ions are fragmented further for structural analysis. Therefore, it is considered destructive. Orbitrap Mass Spectrometry: o Destructive: While the orbitrap analyzer itself doesn’t fragment ions, the ionization techniques used with Orbitrap MS, such as electron or collision-induced ionization, tend to fragment the sample, making it a destructive technique. Fourier-Transform Ion Cyclotron Resonance Mass Spectrometry (FT-ICR-MS): o Destructive: FT-ICR-MS is highly sensitive and provides high resolution, but like other forms of mass spectrometry, the ionization processes used (e.g., electron ionization) can cause fragmentation of the molecules, leading to sample destruction. Magnetic Sector Mass Spectrometry: o Destructive: This technique typically uses ionization methods that fragment the molecules before mass analysis, making it a destructive process. It is widely used for precise isotope ratio analysis, where the sample is consumed. Summary Table: Mass Spectrometry Type Destructive or Non-destructive Electrospray Ionization (ESI-MS) Non-destructive (soft ionization) MALDI-TOF Non-destructive (soft ionization) TOF-MS Destructive Quadrupole MS (QMS) Destructive Ion Trap MS (IT-MS) Destructive Orbitrap MS Destructive FT-ICR-MS Destructive Magnetic Sector MS Destructive Conclusion: Non-destructive techniques like ESI-MS and MALDI-TOF are generally preferred when analyzing large biomolecules or when maintaining molecular integrity is important. Destructive techniques, such as Quadrupole MS, TOF-MS, and Ion Trap MS, involve fragmentation and are useful for structural elucidation but consume the sample in the process. Types of NMR Nuclear Magnetic Resonance (NMR) spectroscopy is a powerful analytical technique used to determine the structure of molecules, study dynamics, and investigate interactions at the atomic level. The different types of NMR focus on the specific nuclei being studied, the dimensionality of the experiment, and the type of information being extracted (e.g., structural, dynamic, or quantitative). Here’s an overview of the main types of NMR: 1. Proton NMR (¹H NMR) Principle: This type of NMR focuses on detecting hydrogen nuclei (protons, ¹H) in a molecule. Applications: o Commonly used to determine the structure of organic compounds. o Provides information on the number of hydrogen atoms, their environment, and the connectivity in the molecule. Key Features: o Produces chemical shifts that indicate the electronic environment of hydrogen atoms. o The splitting patterns (multiplets) give insight into the number of neighboring protons (via spin-spin coupling). o Integration of peaks provides the relative number of protons in different environments. 2. Carbon-13 NMR (¹³C NMR) Principle: Detects the carbon-13 isotope (¹³C), which is present at a natural abundance of about 1% in organic molecules. Applications: o Used for the structural determination of organic compounds, specifically for carbon atom environments. Key Features: o Provides chemical shift information on the carbon atoms, allowing for the identification of functional groups and the skeleton of the molecule. o ¹³C spectra typically don’t show spin-spin coupling with neighboring atoms unless intentionally decoupled. o Due to the low natural abundance of ¹³C, sensitivity is lower than ¹H NMR, often requiring longer acquisition times. 3. 2D NMR (Two-Dimensional NMR) Principle: Extends NMR into two dimensions to study interactions between different nuclei or correlations between chemical shifts within the molecule. Applications: o Common in complex structure determination (proteins, small organic molecules, natural products). o Resolves overlapping signals in 1D NMR spectra. Types of 2D NMR: o COSY (Correlation Spectroscopy): Identifies coupling between protons that are close in space. o HSQC (Heteronuclear Single Quantum Coherence): Correlates hydrogen and heteronuclei (like ¹³C or ¹⁵N) directly bonded to each other. o NOESY (Nuclear Overhauser Effect Spectroscopy): Provides information on spatial proximity between protons, useful for determining the 3D structure of molecules. Key Features: o Reveals correlations between atoms and helps identify connectivity in the molecule. o Offers a higher level of detail for larger, more complex molecules like proteins or large organic structures. 4. Solid-State NMR Principle: Used for studying samples in the solid phase rather than solutions. Applications: o Commonly used to study polymers, inorganic materials, and biological solids like proteins in membranes or amyloid fibrils. Key Features: o Uses techniques like magic angle spinning (MAS) and cross-polarization (CP) to overcome line broadening and improve resolution in solids. o Provides detailed structural information in materials that are difficult to analyze using solution NMR. 5. Heteronuclear NMR Principle: Detects nuclei other than hydrogen (¹H) and carbon (¹³C), such as nitrogen (¹⁵N), phosphorus (³¹P), or fluorine (¹⁹F). Applications: o ¹⁵N NMR: Often used in protein NMR to provide information about the backbone of the protein. o ³¹P NMR: Useful for studying phospholipids, nucleic acids, and organophosphorus compounds. o ¹⁹F NMR: Frequently used in drug development due to the high sensitivity of ¹⁹F and its presence in many pharmaceuticals. Key Features: o Provides information about heteroatoms in molecules, which is important for compounds like proteins, nucleic acids, and organophosphates. o Often used in combination with ¹H NMR for more detailed analysis (e.g., HSQC or HMBC for heteronuclear correlations). 6. Dynamic NMR (DNMR) Principle: Used to study molecular dynamics and how different parts of a molecule move or interact over time. Applications: o Ideal for studying conformational changes, chemical exchange, and reaction mechanisms. o Analyzes kinetics of exchange between different states (e.g., equilibrium between conformers, tautomerism, or ligand binding in proteins). Key Features: o Provides insights into dynamic processes at the atomic level, including molecular motion, interactions, and reaction rates. o Used for understanding time-dependent phenomena like chemical exchange or conformational changes in molecules. 7. Quantitative NMR (qNMR) Principle: Focuses on the quantitative measurement of the amount of a substance in a sample. Applications: o Used in purity assessment, reaction monitoring, and drug formulation. Key Features: o Provides absolute quantification by integrating the signal intensity, which is directly proportional to the number of nuclei being observed. o Can be used for precise quantification of compounds in mixtures without the need for calibration curves. 8. Protein NMR Principle: Specializes in the structural determination of proteins, especially in their native or near-native states in solution. Applications: o Used to determine 3D structures of proteins, protein-ligand interactions, and dynamics. Key Features: o Uses 2D, 3D, or even 4D NMR techniques (like NOESY, HSQC, and TROSY) to study proteins. o Provides detailed atomic-level structural information about proteins in solution, which is essential for studying protein folding, interactions, and dynamics. Comparison Table: NMR Type Principle Applications Key Features ¹H NMR (Proton Focuses on Structure determination Chemical shifts, NMR) detecting of organic compounds. spin-spin coupling, hydrogen nuclei integration. (¹H). ¹³C NMR Detects carbon-13 Structural elucidation of Lower sensitivity, no (Carbon-13) nuclei. carbon environments. coupling unless decoupled. 2D NMR Correlates shifts Complex structure COSY, HSQC, between different determination, protein NOESY—reveal nuclei. NMR. connectivity and spatial proximity. Solid-State NMR of solid- Polymers, materials, MAS, cross- NMR phase samples. biological solids. polarization to improve resolution. Heteronuclear Detects nuclei Proteins, ¹⁵N, ³¹P, ¹⁹F NMR— NMR other than organophosphates, analyzes hydrogen and nucleic acids. heteroatoms. carbon. Dynamic NMR Studies molecular Kinetics, conformational Provides insights into (DNMR) dynamics and exchange, reaction chemical exchange time-dependent mechanisms. rates. processes. Quantitative Quantitative Purity assessment, Directly quantifies NMR (qNMR) analysis of reaction monitoring. substance substance concentration. amounts. Protein NMR NMR applied to Protein structure and 2D, 3D, 4D NMR for study proteins in dynamics. protein folding and solution. interactions. Summary: ¹H NMR and ¹³C NMR are the most common types used for structural elucidation of organic molecules. 2D NMR provides detailed information on connectivity and is essential for studying large molecules and complex mixtures. Solid-state NMR is used when the sample is in a solid state, providing detailed structural information for materials and biological solids. Heteronuclear NMR detects nuclei other than hydrogen, which is particularly useful in studying biological molecules like proteins and nucleic acids. Dynamic NMR allows for the study of molecular motion and reactions over time, and Protein NMR is a specialized technique for detailed structural analysis of proteins. Most Nuclear Magnetic Resonance (NMR) techniques are considered non-destructive because they generally leave the sample intact during analysis. The sample can be recovered after the measurement, unlike other analytical methods (e.g., mass spectrometry) that might consume or alter the sample. Here's an overview of the destructiveness of different types of NMR: Non-Destructive NMR Techniques: ¹H NMR (Proton NMR): o Non-destructive: This is a soft analytical technique, and the sample is not chemically altered during analysis. After the measurement, the sample can usually be reused. ¹³C NMR (Carbon-13 NMR): o Non-destructive: Like ¹H NMR, ¹³C NMR does not destroy the sample. It is used to study carbon atoms in organic compounds and can be repeated on the same sample. 2D NMR (e.g., COSY, HSQC, NOESY): o Non-destructive: These advanced techniques provide correlation information between nuclei without consuming the sample, making them non-destructive. Solid-State NMR: o Non-destructive: While solid samples might be placed in a special rotor for analysis, the technique generally does not chemically alter or destroy the sample. However, in some cases, the sample may not be easily recoverable in the exact form (due to sample packing or mechanical handling), but it remains chemically intact. Heteronuclear NMR (e.g., ¹⁵N, ³¹P, ¹⁹F NMR): o Non-destructive: These NMR techniques are non-destructive, allowing the study of nuclei like nitrogen, phosphorus, and fluorine without altering the chemical structure of the sample. Dynamic NMR (DNMR): o Non-destructive: DNMR is used to observe molecular dynamics over time and does not destroy the sample. It measures how different parts of the molecule change their environment dynamically. Quantitative NMR (qNMR): o Non-destructive: Since it relies on NMR signals to quantify components, this technique does not harm the sample. Protein NMR: o Non-destructive: Used to analyze proteins in solution (often in a buffered aqueous solution). Protein NMR does not destroy the sample, and the same sample can often be reused for additional measurements or analysis. Exception - Destructive Scenarios: NMR typically requires a solvent (usually deuterated) for solution-based samples, which could make the solvent unsuitable for some sample recovery processes, but the sample molecules themselves remain intact. In solid-state NMR, sometimes samples are packed tightly into a rotor, and while the sample itself is not chemically destroyed, retrieving it in its original form may be challenging, especially for soft materials or complex mixtures. Radioactive decay: In rare cases where radioisotopes are used in labeling (e.g., for specialized research), the radioactive sample might decay over time, but this is not typical in standard NMR analysis. Summary: All common NMR techniques (¹H, ¹³C, 2D NMR, solid-state NMR, heteronuclear NMR, DNMR, qNMR, and protein NMR) are non-destructive. The sample remains chemically unchanged, and in most cases, can be reused after the experiment.