Metamorphic lectures 2022 Dr Sarmad Asi Ali.pdf
Document Details
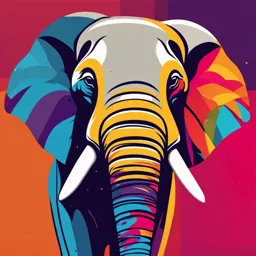
Uploaded by HolyIdiom
University of Kirkuk
2022
Tags
Full Transcript
Metamorphic Petrology Co-ordinator and lecturer Prof.Dr. Sarmad Asi Ali Fresh basalt and weathered basalt TEXTBOOKS “An Introduction To Igneous And Metamorphic Petrology” by John D. Winter (publisher: Prentice Hall) http://www.whitman....
Metamorphic Petrology Co-ordinator and lecturer Prof.Dr. Sarmad Asi Ali Fresh basalt and weathered basalt TEXTBOOKS “An Introduction To Igneous And Metamorphic Petrology” by John D. Winter (publisher: Prentice Hall) http://www.whitman.edu/geology/winter/ Principles of Igneous and Metamorphic Petrology by A.R. Philpotts and J.J. Ague (publisher: Cambridge) Introduction to Mineralogy by W.D. Nesse (Oxford University Press) Objectives Of Metamorphic Petrology ❖Understand that metamorphism occur on Earth because it is still a living planet, with Earth’s material (rocks) constantly experiencing changing pressure (P) and temperature (T) conditions. ❖Appreciate that the production/crystallisation metamorphic mineral assemblages is understood through principles imported from physics and chemistry. In this course this specifically involves (a) and (b). ❖(a) Overview of equilibrium thermodynamics – why rocks melt and why a rock will form different minerals at different metamorphic P,T conditions. ❖(b) Introduction to phase diagrams to describe compositional- mineralogical-melt relationships in common silicate rocks. Week 1 Lecture 1 Introduction to metamorphism The IUGS has proposed the following definition of metamorphism: “Metamorphism is a subsolidus process leading to changes in mineralogy and/or texture (for example grain size) and often in chemical composition in a rock. These changes are due to physical and/or chemical conditions that differ from those normally occurring at the surface of planets and in zones of cementation and diagenesis below this surface. They may coexist with partial melting.” However, strictly Speaking the most intense metamorphic regimes involve The presence of some silicate melt as well. Why Study Metamorphism? Interpretation of the conditions and evolution of metamorphic bodies, mountain belts, and ultimately the state and evolution of the Earth's crust Metamorphic rocks may retain enough inherited information from their protolith to allow us to interpret much of the pre-metamorphic history as well The Limits of Metamorphism: Temperature Low-temperature limit grades from diagenesis Metamorphism begins in the range of 100-150oC for the more unstable types of protolith Some zeolites are considered diagenetic and others metamorphic – pretty arbitrary In the border line between diagenesis and metamorphism new minerals may not form, and relative temperatures are determimned by methods such as crystallinity of illite (a clay mineral) The Limits of Metamorphism High-temperature grades into igneous rocks (anywhere between 650-800ºC depending on the rock chemical composition and fluid(s) present. The high end of metamorphism is marked by melt-producing metamorphic reactions such as: muscovite + plagioclase + quartz = K-spar + aluminosilicate + melt Such rocks are called migmatites The Limits of Metamorphism: Pressure Low-pressure limit Strictly speaking, the lower limit of metamorphism is atmospheric pressure – such as seashells being ‘baked’ by a lava flow However, generally at least some depth of burial is regarded as necessary for the rocks to be considered as metamorphosed if affected by heat The high-pressure limit is variable with temperature, and is governed by the minimum geothermal gradient (TºCkm-1) realised on Earth. Note in Earth’s earlier hotter history this might have been different. The Limits of Metamorphism: Pressure High--pressure limit Lowest geothermal gradient of ~10ºCkm-1 The high-pressure limit is variable with temperature, and is governed by the minimum geothermal gradient (TºCkm-1) realised on Earth. Note that in Earth’s earlier hotter history this might have been different The Divisions of Metamorphism Basic divisions (grades) are low, medium/intermediate and high These divisions are strongly temperature dependant The facies of metamorphism Low grade Medium High The three grades of metamorphism contain several facies (i.e. subdivisions) You will see that many names contain mineral, rock or texture terms The Divisions of of Metamorphism P,T field of metamorphism spans a range of average geothermal gradients. The different average geothermal gradients imply different settings Lowest geothermal gradient is subduction-related Lowish to medium is in regional collision-related metamorphism High geothermal gradient is related to large amounts of magma emplacement at low to moderate pressures The Divisions of of Metamorphism Metamorphic Agents and Changes Lithostatic pressure - uniform stress (hydrostatic) Deviatoric stress = pressure unequal in different directions Resolved into three mutually perpendicular stress (s) components: s1 is the maximum principal stress s2 is an intermediate principal stress s3 is the minimum principal stress In hydrostatic situations all three are equal Metamorphic Agents and Changes Stress Strain → deformation Deviatoric stress affects the textures and structures, but not the equilibrium mineral assemblage Strain energy may overcome kinetic barriers to reactions Foliation is a common result, which allows us to estimate the orientation of s1 s1 Strain ellipsoid s1 > s2 = s3 → foliation and no lineation s1 = s2 > s3 → lineation and no foliation s1 > s2 > s3 → both foliation and lineation Figure 21.3. Flattening of a ductile homogeneous sphere (a) containing randomly oriented flat disks or flakes. In (b), the matrix flows with progressive flattening, and the flakes are rotated toward parallelism normal to the predominant stress. Winter (2001) An Introduction to Igneous and Metamorphic Petrology. Prentice Hall. Metamorphic Agents and Changes Shear motion occurs along planes at an angle to s1 s1 Figure 21.2. The three main types of deviatoric stress with an example of possible resulting structures. b. Shear, causing slip along parallel planes and rotation. Winter (2001) An Introduction to Igneous and Metamorphic Petrology. Prentice Hall. The Types of Metamorphism Different approaches to classification 1. Based on principal process or agent Dynamic Metamorphism Thermal Metamorphism Dynamo-thermal Metamorphism The Types of Metamorphism Different approaches to classification 2. Based on setting Contact Metamorphism ▪ Pyrometamorphism Regional Metamorphism ▪ Orogenic Metamorphism ▪ Burial Metamorphism ▪ Ocean Floor Metamorphism Hydrothermal Metamorphism Fault-Zone Metamorphism Impact or Shock Metamorphism The Types of Metamorphism Contact Metamorphism The size and shape of an aureole is controlled by: The nature of the pluton ▪ Size ▪ Temperature ▪ Shape ▪ Composition ▪ Orientation The nature of the country rocks ▪ Composition ▪ Depth and metamorphic grade prior to intrusion ▪ Permeability Contact Metamorphism Adjacent to igneous intrusions Thermal (± metasomatic) effects of hot magma intruding cooler shallow rocks Occurs over a wide range of pressures, including very low Contact aureole The Types of Metamorphism Contact Metamorphism Most easily recognized where a pluton is introduced into shallow rocks in a static environment → Hornfelses (granofelses) commonly with relict textures and structures The Types of Metamorphism Contact Metamorphism Polymetamorphic rocks are common, usually representing an orogenic event followed by a contact one Spotted phyllite (or slate) Overprint may be due to: ▪ Lag time for magma migration ▪ A separate phase of post-orogenic collapse magmatism (Chapter 18) The Types of Metamorphism Pyrometamorphism Very high temperatures at low pressures, generated by a volcanic or sub-volcanic body Also developed in xenoliths The Types of Metamorphism Regional Metamorphism sensu lato: metamorphism that affects a large body of rock, and thus covers a great lateral extent Three principal types: ▪ Orogenic metamorphism ▪ Burial metamorphism ▪ Ocean-floor metamorphism The Types of Metamorphism Orogenic Metamorphism Figure 21.6. Schematic model for the sequential (a → c) development of a “Cordilleran-type” or active continental margin orogen. The dashed and black layers on the right represent the basaltic and gabbroic layers of the oceanic crust. From Dewey and Bird (1970) J. Geophys. Res., 75, 2625-2647; and Miyashiro et al. (1979) Orogeny. John Wiley & Sons. The Types of Metamorphism Orogenic Metamorphism Q1Metamorphism grades into what at the high-grade end? Q2 What is metasomatism? Week2 The Types of Metamorphism Orogenic Metamorphism Polymetamorphic patterns Continental collision Batholiths are usually present in the highest grade areas If plentiful and closely spaced, may be called regional contact metamorphism The Types of Metamorphism Burial metamorphism Southland Syncline in New Zealand: thick pile (> 10 km) of Mesozoic volcaniclastics Mild deformation, no igneous intrusions discovered Fine-grained, high-temperature phases, glassy ash: very susceptible to metamorphic alteration Metamorphic effects attributed to increased temperature and pressure due to burial Diagenesis grades into the formation of zeolites, prehnite, pumpellyite, laumontite, etc. The Types of Metamorphism Hydrothermal metamorphism Hot H2O-rich fluids Usually involves metasomatism Difficult type to constrain: hydrothermal effects often play some role in most of the other types of metamorphism The Types of Metamorphism Burial metamorphism occurs in areas that have not experienced significant deformation or orogeny Restricted to large, relatively undisturbed sedimentary piles away from active plate margins ▪ The Gulf of Mexico? ▪ Bengal Fan? The Types of Metamorphism Burial metamorphism occurs in areas that have not experienced significant deformation or orogeny Bengal Fan → sedimentary pile > 22 km Geothermal gradient → 250-300oC at the base (P ~ 0.6 GPa) Passive margins often become active Areas of burial metamorphism may thus become areas of orogenic metamorphism The Types of Metamorphism Ocean-Floor Metamorphism affects the oceanic crust at ocean ridge spreading centers Considerable metasomatic alteration, notably loss of Ca and Si and gain of Mg and Na Highly altered chlorite-quartz rocks- distinctive high-Mg, low-Ca composition Exchange between basalt and hot seawater Another example of hydrothermal metamorphism The Types of Metamorphism Fault-Zone and Impact Metamorphism High rates of deformation and strain with only minor recrystallization Impact metamorphism at meteorite (or other bolide) impact craters Both correlate with dynamic metamorphism, based on process (a) Shallow fault zone with fault breccia (a broken & crushed filling in fault zonees) (b) Slightly deeper fault zone (exposed by erosion) with some ductile flow and fault mylonite Figure 21.7. Schematic cross section across fault zones. After Mason (1978) Petrology of the Metamorphic Rocks. George Allen & Unwin. London. Prograde Metamorphism Prograde: increase in metamorphic grade with time as a rock is subjected to gradually more severe conditions ▪ Prograde metamorphism: changes in a rock that accompany increasing metamorphic grade Retrograde: decreasing grade as rock cools and recovers from a metamorphic or igneous event ▪ Retrograde metamorphism: describe any accompanying changes The Progressive Nature of Metamorphism A rock at a high metamorphic grade probably progressed through a sequence of mineral assemblages rather than hopping directly from an unmetamorphosed rock to the metamorphic rock that we find today The Progressive Nature of Metamorphism Retrograde metamorphism typically of minor significance Prograde reactions are endothermic(they consume heat) and easily driven by increasing Temperature. Devolatilization reactions are easier than reintroducing the volatiles Geothermometry indicates that the mineral compositions commonly preserve the maximum temperature Retrograde is usually detectable by observing textures, such as the incipient replacement of high-grade minerals by low-grade ones at their rims Types of Protolith Lump the common types of sedimentary and igneous rocks into six chemically based- groups 1. Ultramafic - very high Mg, Fe, Ni, Cr 2. Mafic - high Fe, Mg, and Ca 3. Shales (pelitic) - high Al, K, Si 4. Carbonates - high Ca, Mg, CO2 5. Quartz - nearly pure SiO2. 6. Quartzo-feldspathic - high Si, Na, K, Al What are the six most common types of metamorphic protolith? What chemically characterizes each? Week3 Causes of Metamorphism Indicator minerals: Diagnostic of certain temperature and pressure regimes. Appearance of these minerals is dependent on the bulk composition of a rock. For example, a pure quartzite (100% silica) can never develop garnet at high temperature Garnet will only appear if the rock has a suitable chemical composition!! Metamorphic Intensity Metamorphism occurs over a T and P range. Different T and P conditions produce different minerals. Metamorphic grade is a measure of the degree of metamorphic alteration. Low grade – Slight. High grade – Intense. Mafic Rock Indicator minerals A word first about the state of the mafic rock: Wet altered basalt. This will show Fresh ‘dry’ plagioclase + pyroxene dolerite. progressive change of mineral Its mineral assemblage is metastable under assemblages (and less water content) atmospheric conditions. When metamorphosed until finally at high temperature there is to high T (800ºC) its plagioclase + pyroxene a dry metamorphic assemblage of mineralogy will not change as these are the plagioclase + pyroxene. stable minerals under those conditions Basic rocks (all with + quartz): Chlorite + epidote + low-Al amphibole (actinolite (Fe) to cummingtonite (Mg) + albite Hornblende + less sodic plagioclase Hornblende + orthopyroxene (hypersthene) + clinopyroxene + less sodic plagioclase ± garnet Basic rocks (all with + quartz): Chlorite + epidote + low-Al amphibole (actinolite (Fe) to cummingtonite (Mg) + albite Chlorite (Mg,Fe,Al)12(Si,Al)8(OH)16 (H20=12wt%) Is a greenish brittle mica. Shown here as replacing biotite (in an altered igneous rock) Basic rocks (all with + quartz): Chlorite + epidote + low-Al amphibole (actinolite (Fe) to cummingtonite (Mg) + albite Epidote family Ca-Fe-Al silicates (H20=2wt%) Epidote + albite (Na-plagioclase is stable, with Ca of protolith’s plagioclase now in epidote Basic rocks (all with + quartz): Chlorite + epidote + low-Al amphibole (tremolite) + albite tremolite (Mg) –actinolite (Fe) amphiboles Ca2(Mg,Fe)5(Si8O22)(OH)2 (H2O = 2 wt%) is an Al-free amphibole. As temperature increases, Al enters the amphibole and it becomes more hornblendic. Chlorite disappears at about 500ºC Basic rocks (all with + quartz): Amphibolite facies Hornblende + less sodic plagioclase ± garnet ± clinopyroxene (you already have met these minerals) Normal, still slightly wet granulite facies (some water held in hornblende) Hornblende + orthopyroxene (hypersthene) + clinopyroxene + less sodic plagioclase ± garnet Rare, completely anhydrous granulite facies Orthopyroxene + clinopyroxene + plagioclase ± garnet (completely dry – rare occurrence) Completely ‘dry’ high grade basic rock (extreme granulite facies). The same assemblage as an igneous rock, but textures are different Pelitic rocks (all with + quartz): Low grade (greenschist facies) Chlorite ± albite + muscovite + biotite Muscovite (mica) colourless in plane polarised light, but brilliant colours in crossed polars. K2Al4(Si6Al2O20)(OH)4 (H2O=4wt%) – note, unlike biotite there is no Fe,Mg. Pelitic rocks (all with + quartz): Medium grade (amphibolite facies) Significance of the aluminosilicate polymorphs kyanite, sillimanite, andalusite (Al2SiO5) They help to resolve P,T of metamorphism in Al-rich rocks like pelites. They will not occur during metamorphism of mafic or granitic rocks of common composition. Pelitic rocks (all with + quartz): Medium grade (amphibolite facies) significance of the aluminosilicate polymorphs kyanite, sillimanite, andalusite (Al 2SiO5) Kyanite = relatively high pressure at a given temperature Kyanite tends to appear blue with bladed habit in hand specimen Pelitic rocks (all with + quartz): Medium grade (amphibolite facies) significance of the aluminosilicate polymorphs kyanite, sillimanite, andalusite (Al 2SiO5) Andalusite = relatively low pressure and not highest temperatures Andalusite in slate showing characteristic ‘X’ in cross section. Sometimes it is Known as chiastolite. It commonly gives spotting in highest grade Kyanite tends to appear blue with bladed slates transforming to schists. habit in hand specimen Pelitic rocks (all with + quartz): Medium grade (amphibolite facies) significance of the aluminosilicate polymorphs kyanite, sillimanite, andalusite (Al 2SiO5) Sillimanite = relatively low pressure at a higher temperature Tends to be acicular in thin section (can be called fibrolite). Dull brown to brilliant purple in X-polars, Kyanite tends to appear blue with bladed colourless to pale-buff in plane habit in hand specimen polarised light Pelitic rocks (all with + quartz): Medium grade (amphibolite facies) Upper medium grade staurolite (Fe,Mg)2(Al,Fe)9O6(SiO4)4(OH)2 (H2O=2 wt%) In some pelitic rocks, only at middle-upper amphibolite facies. In hand specimen appears as reddish-brown ‘bricks’. Yellow colour distinctive in thin section. Bottom picture shows it occurring with sillimanite Metamorphic Intensity and index minerals Particular indicator minerals (appearing and disapearing) are used in rocks of different composition. Shown here are mafic rocks (basalt-gabbro), shale and impure sandstone (pelitic and semi-pelitic) Pelitic rocks – Barrovian sequence (regional metamorphism): Staurolite then kyanite the sillimanite in medium to high grade Pelitic rocks – Buchan sequence (high heat input from magma): Andalusite then sillimanite in medium to high grade Week 4 Lecture 1 Textures, fabrics and recrystallisation in metamorphic rocks Staurolite schist Metamorphism is a dynamic process, with P,T (and fluids) changing with time Metamorphism is a dynamic process, with P,T (and fluids) changing with time Rocks can have microstructures preserved from their protoliths. These are readily destroyed by ductile deformation during metamorphism Preferred mineral orientation is a very common feature of metamorphic rocks: Mechanisms of fabric development in metamorphic rocks Metamorphic reactions need all three Porphyroblasts are larger than average minerals in a metamorphic rock Poikiloblastic texture is when the new minerals engulfs and isolates small remnants of the minerals that were breaking down. As they are isolated from each other, they can no longer react. The large grains are porphyroblasts. Not all porphyroblasts have a poikiloblastic texture shearing flattening folding Transport of ions during reactions has to be by solid state diffusion rather than carried by hydrous fluids 1- What is the difference between rock texture and rock structure? As used in this text, texture refers to small-scale features in a rock that are penetrative, meaning that the texture occurs in virtually all of the rock body at the microscopic scale. Structures are larger-scale features that occur on the hand sample, outcrop, or regional scale. 2- What is recrystallization, and what processes aid it? Recrystallization involves the movement of grain boundaries or the development of new boundaries, both of which produces a different configuration of grains (not subgrains). Processes that aid recrystallization are grain boundary migration, subgrain rotation, solid-state diffusion creep, grain boundary sliding, and grain boundary area reduction. 3- What is the difference between a porphyroblastic, a porphyroclastic, and a blastoporphyritic feldspar in a metamorphic rock? Porphyroblastic texture is one in which a mineral grew metamorphically to form larger crystals (the porphyroblasts) embedded in a finer groundmass. In porphyroclastic texture, larger crystals are also embedded in a finer groundmass, but these are pre- existing crystals that resisted abrasion and cataclasis that reduced the groundmass but left the partly ground and rounded larger remnants. Blastoporphyritic texture refers to relict igneous porphyritic texture that is not entirely obliterated by later metamorphic crystallization. 4- Briefly describe the various types of foliations (including three primary/inherited types). Cleavage: a type of penetrative foliation (affecting rocks to a very fine scale). There are many types, including continuous (slaty), spaced, rough, smooth, parallel, anastomosing, conjugate, gradational, discrete, etc. Crenulation cleavage is two foliations, one the initial cleavage and the other the plane of fold axes that crenulate the first one. Schistosity: parallel-aligned crystals large enough to see. Layering: gneissose texture if the layers represent metamorphic segregation. Primary inherited types: Shaly partings resulting from aligned clays still present in a low-grade slate. Flow banding in a lightly metamorphosed lava flow. Sedimentary bedding in a schist. 5- What determines whether a lineation or a foliation develops?. The type of stress during alignment. If s1 > s2 = s3 then a foliation will develop. If s1 = s2 > s3 a lineation will develop. If s1 > s2 > s3 then both will develop. Week 5 Metamorphism of pelites Metamorphism of pelites (mud ± sand stone) 1: what rocks look like 2: progressive minerals at different apparent geotherms Hornfels = only magmatic heat, no tectonic thickening ‘Hornfels’ contact met. series Abukuma= much magmatic heat, some ‘Abukuma’ series tectonic Moderate apparent thickening geothermal gradients in regional metamorphism Barrovian= mostly tectonic ‘Barrovian’ series thickening, regional heat conduction Moderate pressure metamorphism Remember – the shown geothermal gradients are not the true P,T,t paths. Instead they are a locus joining peak T for different depths in the crust Apparent geothermal gradient = Metamorphic field gradient (most assemblages locked-in at highest T) Start of metamorphism: Pelites-semipelite compositions = Mudstones-shales (+ some sand) These contain mostly clay minerals (from weathering) + detrital biotite + muscovite + quartz At 360-390ºC clay minerals break down to give new (tiny) metamorphic muscovite + biotite + chlorite. Furthermore, in Al-rich compositions, the Al-Si only clay mineral kaolinite will undergo reaction with quartz to form the hydrous aluminosilicate pryophyillite (Al4Si8O20OH4). This is essentially a ‘watery’ aluminosilicate (kyanite, andalusite, silliminate are ‘dry’ aluminosilicates) Thus 360-390ºC marks entry into low grade (greenschist) facies metamorphism Sedimentary rock Metamorphic equivalent Limestone Marble Mudstone Pelite Siltstone Semi-pelite Sandstone Psammite, Quartzite Conglomerate Metaconglomerate Change in metamorphic grade with depth Increasing directed & confining pressure and increasing temperature => slate Slate=lowest grade very fine-grained dark or black Parent rock: shale The main fabric is already tectonic, although on the limbs of folds this is approximately parallel to bedding Form slate-belts – much of the metasedimentary rocks in the Lachlan orogen are of this nature phyllite phyllite fine- to medium-grained, fine crystals of muscovite or chlorite rock now has ‘sheen’ whereas slate does not phyllite slate In thin section dominated by new aligned metamorphic minerals – particularly muscovite and chlorite schist Care needed to distinguish any schist remaining sedimentary coarse-grained, versus strongly foliated metamorphic/ micas seen with tectonic naked eye layering easy to split in flakes/slabs gneiss (para)gneiss coarse-grained, elongated/banded minerals, alternating bands metapelitic gneisses typically carry sillimanite, or kyanite, and garnet Muscovite-consuming reactions, K-spar becoming dominant Al-K bearing phase Orthogneiss derived from an igneous rock paragneiss derivedfrom a sedimentary rock 2: progressive minerals at different apparent geotherms (This shows general division into low-medium- high grade metamorphism) Hornfels = only magmatic heat, no tectonic thickening ‘Hornfels’ contact met. series Abukuma= much magmatic heat, some ‘Abukuma’ series tectonic Moderate apparent thickening geothermal gradients in regional metamorphism Barrovian= mostly tectonic ‘Barrovian’ series thickening, regional heat conduction Indicator minerals, but can do better by looking at mineral assemblages. This takes into account differences due to bulk chemical composition and pressure Thus equivalent B to previous diagram flipped in horizontal (increasing pressure now upwards) A C B = Barrovian, A = Abukuma, C = Contact (Hornfels) Pyrophyllite kaolinite Application of AKF to metapelites Molecular proportions chloritoid Quartz + water always present. The scheme also accounts for plagioclase (Na-Ca) or epidote minerals (Ca) - these can also be present, although not portrayed in this diagram Ky=kyanite, Sil=sillimanite, And=andalusite, St=staurolite, Grt=almandine garnet, Chl=chlorite Bt=bioite, Kfs=K-feldspar, Ms=muscovite, Kln=kaolinite, Prl=pyrophyllite B = Barrovian pyrophyllite B chloritoid A C (1) Lowermost greenschist facies: metapelites dominated by micaceaous minerals (+ quartz + albite) but no biotite. They will have pyrophyllite if they are very aluminous Ky=kyanite, Sil=sillimanite, And=andalusite, St=staurolite, Grt=almandine garnet, Chl=chlorite Bt=biotite, Kfs=K-feldspar, Ms=muscovite, Kln=kaolinite, Prl=pyrophyllite B = Barrovian pyrophyllite B chloritoid A C (2) Mid greenschist facies: appearance of biotite. Metapelites still dominated by micaceaous minerals, but K-feldspar + chlorite are no longer stable Ky=kyanite, Sil=sillimanite, And=andalusite, St=staurolite, Grt=almandine garnet, Chl=chlorite Bt=biotite, Kfs=K-feldspar, Ms=muscovite, Kln=kaolinite, Prl=pyrophyllite B = Barrovian (pyrophyllite) breaks down B chloritoid A C (3) Upper greenschist facies: appearance of garnet and kyanite. Metapelites still dominated by micaceaous minerals, but chlorite no longer stable (gradual consumption, e.g. Fe-Mg chlorite + qtz = almandine + Mg-chlorite) Ky=kyanite, Sil=sillimanite, And=andalusite, St=staurolite, Grt=almandine garnet, Chl=chlorite Bt=biotite, Kfs=K-feldspar, Ms=muscovite, Kln=kaolinite, Prl=pyrophyllite B = Barrovian B A C (4) Lower amphibolite facies: appearance staurolite with disapearance of chloritoid and final consumption of Mg-chlorite. Ky=kyanite, Sil=sillimanite, And=andalusite, St=staurolite, Grt=almandine garnet, Chl=chlorite Bt=biotite, Kfs=K-feldspar, Ms=muscovite, Kln=kaolinite, Prl=pyrophyllite B = Barrovian B A C (5) Mid amphibolite facies: breakdown of staurolite giving common grt + ky + ms assemblage. e.g. staurolite + qtz = almandine garnet + kyanite + water (thus Grt + Ky now stable) Ky=kyanite, Sil=sillimanite, And=andalusite, St=staurolite, Grt=almandine garnet, Chl=chlorite Bt=biotite, Kfs=K-feldspar, Ms=muscovite, Kln=kaolinite, Prl=pyrophyllite B = Barrovian B A C (6) Mid-upper amphibolite facies: sillimanite replaces kyanite giving common grt + sil + ms assemblage – can have start of some muscovite breakdown – K-feldspar producing reactions Ky=kyanite, Sil=sillimanite, And=andalusite, St=staurolite, Grt=almandine garnet, Chl=chlorite Bt=biotite, Kfs=K-feldspar, Ms=muscovite, Kln=kaolinite, Prl=pyrophyllite B = Barrovian B chloritoid A C (7) Upper amphibolite facies: breakdown of muscovite – often with first melts forming giving common Grt + Sil + Kfs (orthoclase) assemblage. e.g. muscovite + quartz = orthoclase + sillimanite + water Ky=kyanite, Sil=sillimanite, And=andalusite, St=staurolite, Grt=almandine garnet, Chl=chlorite Bt=biotite, Kfs=K-feldspar, Ms=muscovite, Kln=kaolinite, Prl=pyrophyllite Barrovian Key indicators are: B (a) garnet appears in upper greenschist facies & (b) kyanite before sillimanite B = Barrovian pyrophyllite B chloritoid A C Next lecture will look at Abukuma (A) and Contact (C) (hornfels) series Ky=kyanite, Sil=sillimanite, And=andalusite, St=staurolite, Grt=almandine garnet, Chl=chlorite Bt=biotite, Kfs=K-feldspar, Ms=muscovite, Kln=kaolinite, Prl=pyrophyllite A A This can be achieved in two ways: (a) In an accretionary-wedge into subduction zone environment, where essentially a cold finger of material is being forced to depth faster than it can thermally equilibrate (b) Double (or more) tectonic thickening of continental crust during a continent-continent or continent-arc collision Week6 – pelites continued A = Abukuma, C = Contact (Hornfels) And some words about quartzofeldspathic rocks B Week6 – pelites continued A C Moderate pressure metamorphism Remember – the shown geothermal gradients are not the true P,T,t paths. Instead they are a locus joining peak T for different depths in the crust Apparent geothermal gradient = Metamorphic field gradient (most assemblages locked-in at highest T) Pyrophyllite kaolinite Application of AKF to metapelites Molecular proportions chloritoid Quartz + water always present. The scheme also accounts for plagioclase (Na-Ca) or epidote minerals (Ca) - these can also be present, although not portrayed in this diagramA Ky=kyanite, Sil=sillimanite, And=andalusite, St=staurolite, Grt=almandine garnet, Chl=chlorite Bt=biotite, Kfs=K-feldspar, Ms=muscovite, Kln=kaolinite, Prl=pyrophyllite A = Abukuma pyrophyllite B chloritoid A C (1) Lowermost greenschist facies: metapelites dominated by micaceaous minerals (+ quartz + albite). They will have pyrophyllite if they are very aluminous. Thus they are like the lowest greenschist facies in Barrovian series (no And or Ky to distinguish) Ky=kyanite, Sil=sillimanite, And=andalusite, St=staurolite, Grt=almandine garnet, Chl=chlorite Bt=biotite, Kfs=K-feldspar, Ms=muscovite, Kln=kaolinite, Prl=pyrophyllite A = Abukuma pyrophyllite B chloritoid A C (2) Mid greenschist facies: Andalusite appears. Metapelites dominated by micaceaous minerals. Andalusite produced by pyrophyllite breakdown: Pyrophyllite + quartz = andalusite + water Ky=kyanite, Sil=sillimanite, And=andalusite, St=staurolite, Grt=almandine garnet, Chl=chlorite Bt=biotite, Kfs=K-feldspar, Ms=muscovite, Kln=kaolinite, Prl=pyrophyllite A = Abukuma B chloritoid A C (3) Upper greenschist to lower amphibolite facies: Cordierite appears – chlorite, chloritoid dissapear. Note that with this higher geothermal gradient that garnet not formed in uppermost greenschist (unlike Barrovian series) Ky=kyanite, Sil=sillimanite, And=andalusite, St=staurolite, Grt=almandine garnet, Chl=chlorite Bt=biotite, Kfs=K-feldspar, Ms=muscovite, Kln=kaolinite, Prl=pyrophyllite A = Abukuma B chloritoid A C (4) Low amphibolite facies: andalusite-sillimanite inversion. Note that biotite + muscovite still coexist Ky=kyanite, Sil=sillimanite, And=andalusite, St=staurolite, Grt=almandine garnet, Chl=chlorite Bt=biotite, Kfs=K-feldspar, Ms=muscovite, Kln=kaolinite, Prl=pyrophyllite A = Abukuma B chloritoid A C (6) Mid-upper amphibolite facies: Muscovite breakdown (often metl producing reaction). K-feldspar + cordierite + sillimanite or K-feldspar + cordierite + biotite Ky=kyanite, Sil=sillimanite, And=andalusite, St=staurolite, Grt=almandine garnet, Chl=chlorite Bt=biotite, Kfs=K-feldspar, Ms=muscovite, Kln=kaolinite, Prl=pyrophyllite C = contact (hornfels) pyrophyllite B chloritoid A C (1) Lowermost greenschist facies: metapelites dominated by micaceaous minerals. They will have pyrophyllite if they are very aluminous. Thus like in the Barrovian and Abukuma series (no And or Ky to distinguish) Ky=kyanite, Sil=sillimanite, And=andalusite, St=staurolite, Grt=almandine garnet, Chl=chlorite Bt=biotite, Kfs=K-feldspar, Ms=muscovite, Kln=kaolinite, Prl=pyrophyllite C = contact (hornfels) pyrophyllite B chloritoid A C (2) Mid greenschist facies: metapelites dominated by micaceaous minerals. Andalusite appears by breakdown breakdown of pyrophyllite Ky=kyanite, Sil=sillimanite, And=andalusite, St=staurolite, Grt=almandine garnet, Chl=chlorite Bt=biotite, Kfs=K-feldspar, Ms=muscovite, Kln=kaolinite, Prl=pyrophyllite C = contact (hornfels) B A C (3) Upper greenschist facies: Breakdown of chlorite and chloritiod and appearance of cordierite. Ky=kyanite, Sil=sillimanite, And=andalusite, St=staurolite, Grt=almandine garnet, Chl=chlorite Bt=biotite, Kfs=K-feldspar, Ms=muscovite, Kln=kaolinite, Prl=pyrophyllite C = contact (hornfels) B A C (4) Upper amphibolite facies: Muscovite breaks down in the stability field of andalusite (in Abukuma it breaks down in sillimanite field). Ky=kyanite, Sil=sillimanite, And=andalusite, St=staurolite, Grt=almandine garnet, Chl=chlorite Bt=biotite, Kfs=K-feldspar, Ms=muscovite, Kln=kaolinite, Prl=pyrophyllite C = contact (hornfels) B chloritoid A C (5) Upper amphibolite facies: Andalusite inversion to sillimanite. This will only happen against gabbroic intrusions (~1100ºC). Most granites (< 800ºC) are too cool for this to happen. Ky=kyanite, Sil=sillimanite, And=andalusite, St=staurolite, Grt=almandine garnet, Chl=chlorite Bt=biotite, Kfs=K-feldspar, Ms=muscovite, Kln=kaolinite, Prl=pyrophyllite pyrophyllite B chloritoid A C Integration of series to try and indicate pressure-related differences B = Barrovian, A = Abukuma, C = Contact (Hornfels) Ky=kyanite, Sil=sillimanite, And=andalusite, St=staurolite, Grt=almandine garnet, Chl=chlorite Bt=biotite, Kfs=K-feldspar, Ms=muscovite B (pyrophyllite) breaks down A chloritoid chloritoid pyrophyllite chloritoid C Ky=kyanite, Sil=sillimanite, And=andalusite, St=staurolite, Grt=almandine garnet, Chl=chlorite Bt=biotite, Kfs=K-feldspar, Ms=muscovite, Kln=kaolinite, Prl=pyrophyllite Remember: Red lines are apparent geothermal gradients – a locus joining the peak T (highest energy) assemblages B locked-in during prograde metamorphism. In regional metamorphism these highest temperatures are commonly achieved during decompression in a clockwise P,T,t loop (related to tectonic C crustal thickening) – when erosion and thermal equilibration have taken- over from tectonic thickening. Therefore in regional metamorphism. many high grade mineral appearances, e.g. cordierite formation occur via high- temperature decompression reactions. However, in contact metamorphism, solely heating is the most important factor Remember: Therefore, in regional metamorphism The actual P,T,t path (grey line) Will be very different from the apparent P,T gradient (red line = geothermal B gradient), created by linking max P,T of samples from the same metamorphic terrane but at different grade (i.e. low grade = greenschist facies to high grade = amphibolite and C granulite facies). On the other hand, in contact Metamorphism there will be much More congruence between the actual P,T,t path and the apparent P,T path (geothermal gradient) A word about quartzo-feldspathic rocks (arkosic sandstones, granitic rocks dacites – rhyloites etc.) These are not particularly useful in pinning-down metamorphic conditions, particularly in low-medium-lower-high grade. This is because of the uninspiring, small variation of assemblages that are produced. This is illustrated here by looking again at the Barrovian sequence: (pyrophyllite) breaks down chloritoid 300-400ºC +quartz 700ºC +albite (plag) 500-550ºC +quartz +epidote loosing epidote (not apparent in diagram) +plagioclase What are the Key indicators in upper greenschist? Week7 (1) Metamorphism of mafic rocks (2) High pressure metamorphism (eclogites and blueschists) Mafic Rock Indicator minerals A word first about the state of the mafic rock: Fresh ‘dry’ plagioclase + pyroxene dolerite. Wet altered basalt. This will show Its mineral assemblage is metastable under progressive change of mineral atmospheric conditions. When metamorphosed assemblages (and less water content) to high T (800ºC) its plagioclase + pyroxene mineralogy until finally at high temperature there is will not change as these are the a dry metamorphic assemblage of stable minerals under those conditions plagioclase + pyroxene. This lecture will consider the ‘wetted’ case, where the progressive reactions with increasing temperatures under different geothermal gradients can be monitored ACF diagram is used for tracking mineral assemblages in mafic rocks. Note that for these there is always quartz in excess, plus water in excess apart from completely anhydrous granulite facies rocks Ky=kyanite, Sil=sillimanite, And=andalusite, St=staurolite, Crd=cordierite Grt=garnet (Alm=almadine – Fe, Prp=pyrope – Mg, Grs=grossular – Ca), Chl=chlorite, Ath=anthophyllite (Ca-Al free amphibole), Opx=orthopyroxene, Tlc=talc,Act=actinolite, Hbl=hornblende, Di=diopside clinopyroxene, Ep=epidote, An=anorthite, Lws=lawsonite, Czo=clinozoisite B = Barrovian Plagioclase is sodic (50) + clinopyroxene or garnet + hornblende (buffered by a water-rich fluid phase). A = Abukuma Plagioclase is calcic (>An50) + hornblende + garnet or diopside B A C Diopside rather than garnet will be present in very Ca-rich compositions (5b) Low pressure granulite facies: If completely anhydrous (e.g. presence of very hydroscopic melt), the final assemblage will be garnet or clinopyroxene + plagioclase + orthopyroxene (+quartz). Onset and/or progression of granulite facies is commonly associated with melt-producing reactions. In this example an orthopyroxene + plagioclase assemblage is being produced by a reaction giving a tonalitic melt (2717±3 Ma granulite facies metamorphism in mafic rock showing earlier higher pressure metamorphism (garnet + clinopyroxene coexist) HP = High pressure metamorphism Generally shown by the presence of glaucophane (an amphibole) at lower temperatures and by omphacite (a special kind of clinopyroxene) at higher temperatures. Garnet is another important mineral High pressure metamorphism can only be achieved by rapid and extreme pressure increase, either as an extreme case of HP Barrovian style metamorphism in collisional orogeny or within accretionary wedge - subduction zone environments Garnet becomes more Ca-rich with increasing pressure B A A This can be achieved in two ways: (a) In an accretionary-wedge into subduction zone environment, where essentially a cold finger of material is being forced to depth faster than it can thermally equilibrate (b) Double (or more) tectonic thickening of continental crust during a continent-continent or continent-arc collision Blueschists: glaucophane A feature of high pressure metamorphism is the coupled Na,Al solution into amphiboles to give glaucophane and into clinopyroxene to give omphacite Glaucophane: Na2(Mg,Fe)3(Al,Fe3+)2Si8O22(OH)2 Blueschists Generally shown by the presence of omphacite at lower temperatures and wetter conditions. Ca held in epidote-clinozoisite phases. Garnet already common in blueschists. Omphacitic clinopyroxene may already be present in blueschist facies HP B glaucophane Eclogites: omphacite Coupled Na,Al solution into clinopyroxene to give omphacite Jadeite Na,Al(Si2O6) Component in omphacite clinopyroxene Na,Al(Si2O6) + Ca(Mg,Fe)(Si2O6) Eclogites consist of omphacite + Ca-rich garnet ± glaucophane + quartz Omphacite (Na,Al) substitution, Additional Al-substitution remember ACF formulation! Eclogites Generally shown by the dominance of Ca-rich garnet and Al-rich clinopyroxene omphacite. Until anhydrous, glaucophane will be present. Kyanite can occur in most aluminous mafic rocks. Epidote-clinozoisite breaking down with increasing temperature. Quartz present in ‘low’ Pressure eclogites, coesite in ultra-high pressure metamorphism. HP B anhydrous eclogite High pressure & ultra-high pressure metamorphism In the past 20 years rare relicts of very high pressure metamorphism have been found in exhumed subduction zone rocks in collisional orogens. Diagnostic phases are coesite - the high pressure form of quartz, and (metamorphic) diamond, the high pressure form of graphite Boundary between normal regional metamorphism and eclogite is the disappearance of plagioclase. Above that, at high temperatures diamond are first quartz-eclogites and graphite then coesite-eclogites and then coesite+diamond bearing rocks. At low temperatures diamond UHP becomes stable at lower pressures than coesite - note the crossover Eclogite HP in the polymorph phase boundaries Normal regional Coesite+ diamond bearing metamorphic rocks are the rarest of all metamorphic rocks! Ultra-high pressure metamorphism Ultra-high pressure metamorphism requires exceptionally rapid burial, and only occurs in subduction zones. The problem then is it them heating up, in a clockwise P,T,t loop. In order to preserve the assemblages from retrogression during exhumation, this part of the loop has to be as rapid. As it is, most of the HP evidence is lost by recrystallisation at high temperatures and dropping pressure diamond graphite UHP Eclogite HP Normal regional exsolution of albite (dark) out of clinopyroxene (light), leaving eclogite facies at high temperature. Originally was omphacite Ultra-high pressure metamorphism Ultra-high pressure metamorphism requires exceptionally rapid burial, and only occurs in subduction zones. The problem then is it then heating up, in a clockwise P,T,t loop. In order to preserve the assemblages from retrogression during exhumation, this part of the loop has to be as rapid. As it is, most of the HP evidence is lost by recrystallisation at high temperatures and dropping pressure diamond graphite UHP Eclogite HP Normal regional Inversion of coesite to quartz, as inclusion protected in garnet. radial fractures are because of expansion to lower density quartz UHP metamorphism Real example: Kokchetav massif - Kazakstan. Integration Double-thickened of micro- crust metamorphic petrology with base of stable zircon dating crust B Metamorphic zircon rim with diamond 25 µm inclusion