Metabolism I Lecture Notes PDF
Document Details
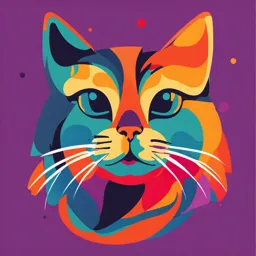
Uploaded by CredibleRationality
Helwan University
2025
Manar Seleem
Tags
Summary
This document provides lecture notes on carbohydrate metabolism, focusing on catabolic and anabolic pathways, glycolysis, and glucose transport. The notes cover topics like glucose-phosphorylation, different types of glucose transporters (GLUT isoforms), and the Na+-monosaccharide co-transporter system. The content appears to be part of a Biotechnology and Genetic Engineering course, likely targeting an undergraduate level.
Full Transcript
Biotechnology and Genetic Engineering Metabolism I BIO 2101 2nd Level Lect. (1) 2024- 2025 Biotechnology and Genetic Engineering Presented By: Assoc. Prof. Manar Seleem Biotechnology and Genetic...
Biotechnology and Genetic Engineering Metabolism I BIO 2101 2nd Level Lect. (1) 2024- 2025 Biotechnology and Genetic Engineering Presented By: Assoc. Prof. Manar Seleem Biotechnology and Genetic Engineering Chapter 1 Contents Carbohydrate Metabolism: 1-Introduction 2-Catabolic pathways 3-Anabolic pathways 4-OVERVIEW OF GLYCOLYSIS 5-Transport of Glucose into Cell a- Tissue-specificity of GLUT gene expression b- 2. Specialized functions of GLUT isoforms c- Na+-monosaccharide cotransporter system 6-REACTIONS OF GLYCOLYSIS 7-Glucose-phosphorylation a- Hexokinase b- Glucokinase Biotechnology and Genetic Engineering A-Introduction A-Investigating metabolism through the examination of its component pathways is a convenient method. Each pathway is comprised of multienzyme sequences, and each enzyme may possess significant catalytic or regulatory characteristics. This map is beneficial for the purpose of visualizing the purposeful "movement" of metabolic intermediates, delineating connections between pathways, Biotechnology and Genetic Engineering Biotechnology and Genetic Engineering A-Introduction A-Investigating metabolism through the examination of its component pathways is a convenient method. Each pathway is comprised of multienzyme sequences, and each enzyme may possess significant catalytic or regulatory characteristics. This map is beneficial for the purpose of visualizing the purposeful "movement" of metabolic intermediates, delineating connections between pathways. Biotechnology and Genetic Engineering A-Catabolic pathways Catabolic reactions are responsible for the extraction of chemical energy in the form of adenosine triphosphate (ATP) from the degradation of energy-rich fuel molecules. Catabolism also enables the conversion of molecules in the diet (or nutrient molecules stored in cells) into the building blocks necessary for the synthesis of complex molecules. Figure 8.3 illustrates the three phases in which energy is generated through the degradation of complex molecules. [Note: Coenzymes, such as NAD+, are typically required for catabolic pathways, which are typically oxidative.] A-Catabolic pathways Biotechnology and Genetic Engineering Biotechnology and Genetic Engineering 1.Hydrolysis of complex molecules: In the first stage, complex molecules are broken down into their component building blocks. For example, proteins are degraded to amino acids, poly - saccharides to monosaccharides, and fats (triacyl glycerols) to free fatty acids and glycerol. Biotechnology and Genetic Engineering 2. Conversion of building blocks to simple intermediates: In the second stage, these diverse building blocks are further degraded to acetyl coenzyme A (CoA) and a few other, simple molecules. Some energy is captured as ATP, but the amount is small compared with the energy produced during the third stage of catabolism. Biotechnology and Genetic Engineering 3. Oxidation of acetyl CoA: The tricarboxylic acid (TCA) cycle is the final common pathway in the oxidation of fuel molecules that produce acetyl CoA. Oxidation of acetyl CoA generates large amounts of ATP via oxidative phosphorylation as electrons flow from NADH and FADH2 to oxygen. Biotechnology and Genetic Engineering c. Anabolic pathways Anabolic reactions involve the combination of small molecules, such as amino acids, to produce complex molecules, such as proteins (Figure 8.3). The decomposition of ATP to adenosine diphosphate (ADP) and inorganic phosphate (Pi) is the primary source of energy required for anabolic reactions, which are endergonic. The electron donor NADPH is the most common source of reducing power in anabolic reactions, which frequently involve chemical reductions. It is important to recognize that catabolism is a convergent process, meaning that a diverse array of molecules is converted into a limited number of common end products. In contrast, anabolism is a divergent process in which a limited number of biosynthetic precursors generate a diverse array of polymeric or complex products Biotechnology and Genetic Engineering c. Anabolic pathways Biotechnology and Genetic Engineering OVERVIEW OF GLYCOLYSIS All tissues utilize the glycolytic pathway to break down glucose, which is used to generate energy (ATP) and intermediates for other metabolic pathways. Glycolysis is the central process in carbohydrate metabolism, as nearly all sugars, regardless of whether they are derived from the diet or catabolic reactions in the body, can be converted to glucose (Figure 8.4A). In cells with mitochondria and an adequate supply of oxygen, pyruvate is the end product of glycolysis. This sequence of ten reactions is referred to as aerobic glycolysis, as oxygen is necessary to reoxidize the NADH that is produced during the oxidation of glyceraldehyde 3-phosphate Biotechnology and Genetic Engineering OVERVIEW OF GLYCOLYSIS Aerobic glycolysis establishes the foundation for the oxidative decarboxylation of pyruvate to acetyl CoA, a significant fuel source in the TCA (or citric acid) cycle. In contrast, pyruvate is converted to lactate through the oxidation of NADH to NAD+ (Figure 8.4C). Anaerobic glycolysis is the term used to describe the process of converting glucose to lactate in the absence of oxygen. The production of ATP is facilitated by anaerobic glycolysis in tissues that are devoid of mitochondria, such as red blood cells, or in cells that are insufficiently oxygenated. Biotechnology and Genetic OVERVIEW OF GLYCOLYSIS Engineering Biotechnology and Genetic Engineering TRANSPORT OF GLUCOSE INTO CELLS Glucose cannot diffuse directly into cells, but enters by one of two transport mechanisms: a Na+-independent, facilitated diffusion transport system or a Na+- monosaccharide cotransporter system. A. Na+-independent facilitated diffusion transport A family of 14 glucose transporters in cell membranes mediates this system. They are referred to as GLUT-1 to GLUT-14 (glucose transporter isoforms 1–14). Two conformational states of these transporters are present in the membrane (Figure 8.10). Extracellular glucose binds to the transporter, which subsequently modifies its conformation to facilitate the transportation of glucose across the cell membrane Biotechnology and Genetic Engineering 1- Tissue-specificity of GLUT gene expression: The glucose transporters exhibit a pattern of expression that is specific to each tissue. For instance, neurons are equipped with the primary glucose transporter, GLUT-3. Biotechnology and Genetic Engineering 1- Tissue-specificity of GLUT gene expression: The glucose transporters exhibit a pattern of expression that is specific to each tissue. For instance, neurons are equipped with the primary glucose transporter, GLUT-3. The abundance of GLUT-1 is high in erythrocytes and the blood-brain barrier, but it is minimal in adult muscle. Conversely, GLUT-4 is abundant in skeletal muscle and adipose tissue. [Note: Insulin increases the number of GLUT-4 transporters that are active in these tissues. Biotechnology and Genetic Engineering 2. Specialized functions of GLUT isoforms: Glucose movement in facilitated diffusion is characterized by a concentration gradient, which is a progression from a high glucose concentration to a lower one. For instance, glucose uptake from the blood is primarily regulated by GLUT-1, GLUT-3, and GLUT-4. By contrast, GLUT-2, which is located in the liver and kidney, has the ability to either transport glucose into these cells when blood glucose levels are high or transport glucose from these cells when blood glucose levels are low (e.g., during fasting). [Note: Pancreatic β cells also contain GLUT-2.] GLUT-5 is unique in that it serves as the primary transporter of fructose in the small intestine and the testes, rather than glucose. Biotechnology and Genetic Engineering Na+-monosaccharide cotransporter system This is an energy-intensive process that transports glucose "against" a concentration gradient, meaning that it moves from low glucose concentrations outside the cell to higher concentrations within the cell. This system is a carrier-mediated process in which the concentration gradient of Na+ is coupled to the movement of glucose, which is simultaneously transported into the cell. A sodium-dependent glucose transporter (SGLT) serves as the carrier. The epithelial cells of the intestine, renal tubules, and choroid plexus are the sites of this type of transport. [Note: GLUT-1 is also present in the choroid plexus, which is a component of the blood-brain barrier.] Biotechnology and Genetic Two phases of aerobic glycolysis Engineering Two phases of aerobic glycolysis The conversion of glucose to pyruvate is a two-step process (Figure 8.6). The initial five reactions of glycolysis are classified as an energy investment phase, during which the phosphorylated forms of intermediates are synthesized at the expense of ATP. The subsequent reactions of glycolysis are an energy generation phase in which a net of two molecules of ATP are formed by substrate-level phosphorylation per glucose molecule metabolized. Glucose-phosphorylation A. 1- Because there are no specific transmembrane carriers for phosphorylated sugar molecules, they are unable to readily permeate cell membranes. A 2- dditionally, they are too polar to diffuse through the lipid core of membranes. As a result of the irreversible phosphorylation of glucose the sugar is effectively trapped as cytosolic glucose 6- phosphate, thereby committing it to further metabolism within the cell. The phosphorylation of glucose to glucose 6-phosphate is catalyzed by multiple isozymes of the enzyme hexokinase in mammals. Hexokinase Hexokinase is one of three regulatory enzymes of glycolysis that catalyze the phosphorylation of glucose in the majority of tissues (see also phosphofructokinase and pyruvate kinase). Hexokinase is capable of phosphorylating a variety of hexoses in addition to glucose and has a broad substrate specificity. The reaction product, glucose 6-phosphate, inhibits hexokinase and accumulates when the metabolism of this hexose phosphate is reduced. Hexokinase exhibits a high affinity for glucose and a low Km (refer to p. 59). This enables the efficient phosphorylation and subsequent metabolism of glucose, even in the presence of modest glucose concentrations in the tissue (Figure 8.7). However, hexokinase has a low Vmax for glucose and, as a result, is unable to sequester (trap) cellular phosphate in the form of phosphorylated hexoses or phosphorylate more carbohydrates than the cell can effectively utilize. 1. Glucokinase: The primary enzyme responsible for the phosphorylation of glucose is glucokinase, which is also known as hexokinase D or type IV. It is found in liver parenchymal cells and β cells of the pancreas. Glucokinase serves as the glucose sensor in β cells, thereby establishing the insulin secretion threshold. During hyperglycemia, the enzyme in the liver promotes the phosphorylation of glucose. [Note: Hexokinase also functions as a glucose sensor in neurons of the hypothalamus, where it is essential for the adrenergic response to hypoglycemia.] The enzyme's sugar specificity is comparable to that of other hexokinase isozymes, despite its popular but misleading appellation, glucokinase. THANK YOU