Ion Channels and Resting Membrane Potential PDF
Document Details
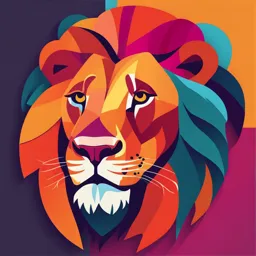
Uploaded by SuperbDidactic
Unipa
Tags
Summary
This document discusses ion channels and resting membrane potential. It explains different types of ion channels and their roles in generating electrical signals in neurons. The document also describes the concept of membrane potential and its importance in nerve function.
Full Transcript
Ion Channels and Resting membrane potential The nervous system function are based on neurons activity that generate, trasmit and elaborate nervous information. Nervous information depends on the changes of the membrane potential provoked by the opening or closure of ionic channels....
Ion Channels and Resting membrane potential The nervous system function are based on neurons activity that generate, trasmit and elaborate nervous information. Nervous information depends on the changes of the membrane potential provoked by the opening or closure of ionic channels. Neuron Fessura sinaptica in Terminazione sinaptica Dendriti Nucleo dendrite Soma Segmento iniziale Sinapsi Segmento Assone inibitoria mielinico Nodo Sinapsi Ranvier eccitatorie Ion Channels Intrinsic membrane proteins. Made by different subunits forming a pore to make a specific ion pass the membrane (Na+, K+, Ca2+ etc.). Across the membrane ionic fluxes produce rapid changes of membrane potential. Heteromeric channels Homomeric channels Ion channel selectivity Ion channels can have either high selectivity for an ion or allow the passage of different types of ions (Na+, K+ and Ca2+). Selectivity depends on chemical interactions with the P domain (polar aminoacid residues that bind loosely with the ion) and on the pore size. The ions are surrounded by H2O molecules (solvation) with dimensions proportional to the concentration of the electric charge. ↓atomic radius ➔ ↑charge concentration ➔ ↑solvation Charge: Na+ = K+ Atomic radius: Na+ (0.095 nm) < K+ (0.133 nm) solvation radius: Na+ > K+ The ion leaves a relevant part of H2O molecules, binds for a very short time ( a a’ High conductance a g= tan(a) (g) i (pA) Low conductance (g) The slope indicates the value of the conductance Vm (mV) Ohmic Channels: have a constant conductance. Linear relationship between DV (at the two sides of the membrane) and current (i) flowing through the channel. Rectifying Channnels: with variable conductance. They conduct better for certain values of Vm. Voltage-gated ion channels Closed at resting membrane potential, they open after changes in the membrane potential. Highly selective for a specific ion species, they are characterized by an activation threshold (i.e. the minimum value that the membrane potential must reach for the channel to open). The opening depends on the presence of a voltage sensor (constituted by positively or negatively charged aminoacid sequences) which opens the channel gate Na+ Channel Inactivation gate S4 Selectivity filter ▪ Segment S4 = voltage sensor (positively charged aminoacids) triggers channel opening. ▪ P Region = selectivity filter. ▪ domain III-IV loop = inactivation gate ▪A change in the membrane potential (depolarization) determines an outward displacement of the S4 segment, which is transmitted to the P region (selectivity filter). ▪ Channel inactivation occurs through a folding of the loop between domains III and IV (inactivation gate), which occludes the pore from the internal side. Main voltage-gated channels Na+ channels: (Nav1.1-Nav1.9). Composed by a subunits (sufficient to generate a functional pore with voltage-dependence) associated with one or more β subunits (β1 or β3 and β2), which modulate the kinetics and voltage-dependence of the channel. Involved in the genesis of the action potential. Blocked by TTX. Functional properties Low activation threshold, Intense ion flow, Rapid inactivation kinetics (responsible for the absolute refractoriness of the action potential) K+ channels: activated by voltage (Kv, 12 families and numerous subtypes) and by Ca2+ (KCa). Formed by 4 equal a subunits, with a single domain of 6 segments. Functional properties Slow inactivation kinetics, responsible for the repolarization phase of the action potential of which they determine the duration. Ca2+ channels: 3 families (Cav1, Cav2, Cav3) with different isoforms. Formed by different subunits (a1, which forms the pore of the channel, β, g and a2) Functional properties High threshold activation (HVA) channels: Cav1 and Cav2: type L, N, P / Q and R. They activate at potentials around -20mV, and slowly inactivate. Low threshold activation (LVA) channels: Cav3: type T. Activate at potentials close to the resting potential (-65mV, -50mV) and quickly inactivate. Ion channels and membrane potential Membrane potential: difference in electric potential across the cell membrane (negative inside), determined by the different ionic distribution at the two sides of the membrane. In excitable cells (nerve and muscle cells) it is referred to as resting potential since it is the voltage across the membrane of cells at the resting state. Membrane potential Potential difference across the membrane In mammal cells it ranges between -65mV and -70 mV. In nerve and muscle cells (excitable cells) it changes in response to specific stimuli, which cause ion fluxes across the membrane. The most important modification of the membrane potential is the action potential, which is responsible for the transmission of nerve information and muscle contraction. The membrane potential depends on the different distribution of electric charges (ions) between the inside and outside of the cell. The cell membrane separates two solutions: the intracellular and extracellular or interstitial fluid with different ionic composition. Cell membrane Intracellular solution: High conentration of K+ and A- (protein anions) Extracellular solution: High concentration of Na+ e Cl- Intracellular fluid Interstitial fluid How is the membrane potential generated? Model to explain its existence Semi-permeable membrane (permeable only to the K+ ion) to separate the intra- and extracellular compartments that have different ionic content. Membrane permeable only to K+ _ + Excess of negative charges Excess of positive charges K+ Cl- Na+ Extracellular Intracellular K+ _ Cl- + K+ Na+ K+ Cl- K+ K+ Na+ Cl- K+ is pushed out according to its concentration gradient. The flow of + charges is not followed by an equivalent flow of - charges When the electronegativity inside the cell is enough to create an electric force (electric gradient), which opposes the chemical one (concentration gradient), the tendency of the K+ to go out is equal to its tendency to enter the cell. The potential difference at which this equilibrium is reached is the equilibrium potential for the K+. The membrane potential is generated by: Presence of ion concentration gradient Selective permeability of the membrane If the membrane is permeable to K+ only, the membrane potential is the equilibrium potential of K+ (Ek) The equilibrium potential of an ion Defined by Nerst’s equation Ex = Equilibrium potential for a given ione R = universal gas constant RT [X]out T = absolute temperature (in Kelvin, -273°C) Ex = ln F = Faraday’s constant (96,485 Coulomb/mol) zF [X]in z = valence of the ionic species Xin = intracellular concentration of the ionic species X Xout= extracellular concentration of the ionic species X At the temperature of 25 ° C, RT / F = 25.2 mV. By transforming the natural logarithm (ln) into decimal logarithm (log) (log x = 2.303 ln) we have: [X]out monovalent Ex = 58 mV log [X]in cation If [X+]out/[X+]in > 1 ➔ Ex>0 If [X+]out/[X+]in < 1 ➔ Ex