NAS Lecture Notes on Membrane Physiology and Neuronal Excitability PDF
Document Details
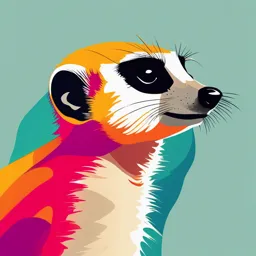
Uploaded by HandsDownStrontium7431
NAS
Tags
Summary
These lecture notes cover membrane physiology, including cell membrane structure, ion channels, electrochemical gradients, equilibrium potential, and the resting membrane potential. The importance of the Na/K pump and action potential initiation is also described.
Full Transcript
**[NAS Lecture 2 -- Membrane Physiology]** **Structure of the cell membrane**Diagram of cell membrane with text Description automatically generated The structure of the plasma membrane is a **bilayer** consisting of phospholipid molecules with the phosphate heads facing outwards (on the surface) a...
**[NAS Lecture 2 -- Membrane Physiology]** **Structure of the cell membrane**Diagram of cell membrane with text Description automatically generated The structure of the plasma membrane is a **bilayer** consisting of phospholipid molecules with the phosphate heads facing outwards (on the surface) and the fatty acid tails facing the inside of the bilayer. - This makes the membrane **impermeable** to polar molecules. - The plasma membrane's structure also contains proteins such as **ion channels** (passive movement of ions) and **ion pumps**. Ion channels can be voltage-gated (generate action potentials), ligand-gated, mechanically-gated, non-gated, or leak (always open). These factors allow the plasma membrane to create an intracellular environment which is different to the external environment. **Functions of the cell membrane** - Defines boundaries of the cell -- cells have varied shapes to enable them to carry-out their functions. - Encloses the cell's organelles -- nucleus, nissl substance, mitochondria etc. - Enables the cell to create an internal environment that promotes the normal function of the cell -- creates an internal environment that is different from the outside of the cell. - The cell membrane is able to influence the composition of the intracellular compartment but not the extracellular compartment. (extracellular compartment aka interstitial space and intraceullular environment are both largely aqueous environments. **Ion channels** - The exposed surface of a protein can be chemically heterogeneous. E.g. the exposed ends of a channel proteins are hydrophilic and their middle surfaces (embedded in the membrane) are hydrophobic. - Ions channels have ion selectivity and a gating mechanism - they are essential for controlling Em (ion movement is passive) **Electrochemical gradients** The accumulation of ions in different concentrations inside and outside cells leads to the establishment of 2 gradients: - **Chemical** gradient -- caused by **concentration** **difference** inside and outside the cell. - **Electrical** gradient -- membrane potential caused by difference in **polarity** (charge) inside and outside the cell. The combined effect is known as the **electrochemical** **gradient**. The ions involved in setting up membrane potential are mainly Na^+^ and K^+^, in addition partly to Cl^-^ and Ca^2+^. Ion Concentration inside (mM) Concentration outside (mM) ------ --------------------------- ----------------------------- K+ **150** 5.5 Na+ 15 **150** Ca2+ 1 x 10^-7^ **1.8** Cl- 9 **125** **Equilibrium potential** Equilibrium potential is the membrane potential where there is no net movement of one particular ion into or out of a cell. Each ion has its own equilibrium potential, as this depends on the charge of the ion and its concentrations inside and outside the cell. The following example covers the equilibrium potential of K^+^: and how it could be set up: 1. The inside of the cell is more -ve than the outside (the inside of a all cells contains a minute excess of anions leading to a negative voltage within the cell).\ \ At first, there are more K^+^ ions on the inside of the cell, so a **chemical force** is pushing the ions outwards through **leak K^+^ channels**.\ There is also a weak **electrical force** attracting some K^+^ ions back into the cell, because it is more -ve inside.\ Chemical force \> electrical force, therefore **K^+^ ions are leaving the cell**. 2. As K^+^ ions leave the cell, the -ve charge inside the cell increases, this causes the electrical force acting inwards to increase. The chemical force does not change much, because only a small number of ions needs to be exchanged to sufficiently change the membrane potential. 3. Eventually, there is a point where chemical force (outwards) = electrical force (inwards). Therefore there is **no net movement** of K^+^. The membrane potential at which this happens is the equilibrium potential for K^+^ (**E~K+~**). If the membrane was only permeable to K+ ions, then the resting membrane potential of the cell would be equal to E~K+~. However this is not the case, because the membrane is also permeable to other ions. The equilibrium potential for an ion can be calculated using the **Nernst** **equation** *(do not need to memorise)*: Nernst Equation : r/Mcat  **Interaction of other ions to create resting membrane potential** Following up from the equilibrium potential of K^+^, now Na^+^ is considered as well: 1. There are more Na^+^ ions outside the cell than inside.\ Therefore, there is a chemical force driving Na+ ions into the cell.\ In addition, the inside of the cell is negative, therefore there is a strong electrical force also acting inwards.\ Overall, this creates a strong driving force for Na^+^ into the cell. 2. As a result of Na+ entering the cell, the **inside becomes less negative**, so the electrical force attracting Na^+^ into the cell becomes weaker. Therefore, **less Na+ ions enter the cell**.\ \ In addition, the electrical force attracting K^+^ back into the cell becomes weaker, so **K^+^ ions begin to leave** the cell. 3. Eventually, there is **equal movement** of **Na+ into** the cell as **K+ out** of the cell. A diagram of a chemical reaction Description automatically generated The membrane potential of the cell is now very close to the **resting membrane potential**. The interaction of Cl- must also be considered in order to achieve a more accurate value. **[Resting Electrical Properties]** - Resting Membrane Potential (Em) = -60 to -70 mV - This means: - There is an excess of negative charge inside the cell - The voltage is stable -- so there is an equal movement of ions inside and outside the cell (explained below) - How is this achieved? 1. **The permeability of the membrane to the ion: how easily can they cross the membrane?** - The plasma membrane contains Non-gated (leak): K+ channels and Na+ channels - Therefore, the membrane has a permeability to each ion (P~K~ , P~Na~) - The number of the leak channels for each different ion is the factor which sets the permeability for that ion. 2. **Factors influencing ion movement (flux) across the membrane ** - **Chemical gradient**: There is an unequal ion distribution so ions will flow down their concentration gradient - K+ concentration is higher inside 🡪 driven to leave cell through ion channels (efflux) - Na+ concentration is higher outside 🡪 driven to enter cell through ion channels (influx) - **Electrical force:** Ions are charged (Na+/K+) thus are attracted by voltage inside cell (Em) - At negative Em, drive for K+ and Na+ to move into the cell (influx) Driving an ion across the membrane electrically requires: - the membrane possesses channels permeable to that ion to provide conductance - There is an electrical potential difference across the membrane The **Goldman** **equation** can be used to estimate resting membrane potential given the concentrations of the 3 ions inside and outside the cell, as well as their relative **permeability**.  Because the membrane is much **more permeable to K^+^ ions** (due to more leak channels), the resting membrane potential of neurones is closer to E~K+~ than E~Na+~. RMP of a neurone is approximately **-70 mV**. Nernst equation can be simplified to **E~ion~ = 61mV x log~10~ (\[ion\] out / \[ion\] in)** **E~ion~** for K+ will be the lowest part of the action potential curve and **E~ion~** for Na+ will be the highest part of the action potential curve. **Role of the Na/K pump** A diagram of a structure Description automatically generated The Na/K pump plays a role in maintaining the resting membrane potential. Because there is a net entry of Na^+^ and net exit of K^+^, the Na/K pump neutralises this movement by pumping **3 Na+ ions out** of the cell, and **2 K+ ions into** the cell. The Na/K pump DOES NOT create the resting membrane potential, it only helps **maintain** it. **Electrically Excitable Biological Tissues** - A defining characteristic of electrically excitable biological tissues is that they are able to let out a momentary discharge of the standing electrical potential between the intra and extracellular compartments. - All living biological tissue have a resting membrane potential. - In nervous tissue the process of discharging the standing electrical potential is also the mechanism of electrical communication between cells. - Upon discharge the cell membrane works to restore the resting membrane potential. - The process of death is characterised by the permeanent discharge of resting membrane potentials of electrically excitable tissues. **Factors that define whether or not particles cross the cell membrane?** - Size of particles - Electrical charge of particle - Whether particles are recognised by specialised transport systems that cross the membrane - Solubility of molecules in water - Transport ATPases (ion pumps) - Presence of ion channels (leak channels and selective or non-selective channels). **Channel Ions** - Peripheral or trans-membrane spanning proteins span the full thickness of the cell membrane. - They facilitate passive movement of ions across the membrane. - Some are selective in which ions cross others are less selective in terms of which ions they conduct. - Selectivity filter -- filters structures for size and charge etc - Cavity -- if meets selectivity filter it enters the cavity, in hydration cavity molecules pick up water and reach maximum size. - Gating is last -- only certain ions can exit the gate (eg water oxygen enters but not water potassium ions as their overhydrated). **Ion Pumps** - Maintains RMP -- net entry of Na+ and net exit of K+. - Na/K pump neutralises this movement by pumping 3Na+ out and 2K+ in the cell. - Doesn't create RMP, only maintains it. - They require energy in the form of ATP to maintain the RMP **[NAS Lecture 3 -- Neuronal Excitability]** **Initiating the action potential** In order to initiate an action potential, there needs to be an initial small influx of Na^+^ ions into the cell, caused by a stimulus. This could happen by: - Mechanical pressure (e.g. in mechanoreceptors) - Chemical (e.g. neurotransmitter in synapses) - Electrical (e.g. current flow between heart muscle cells) This influx needs to be sufficient to increase the membrane potential from -70 mV to approximately -55 mV, this is the threshold potential. - If the threshold potential is not reached, an action potential will not be generated. - If the threshold potential is reached, a positive feedback mechanism will lead to the generation of an action potential of the same intensity, regardless of the strength of the initial stimulus. This is the **all-or-nothing principle**. - The level of depolarisation will be proportional to the strength of stimulation applied -- this is known as graded depolarisations which can become an action potential once the threshold is reached. **Equilibrium Potential -- no net movement of one particular ion into or out the cell.** - K+ ions: 150mV inside vs 5.5mV outside chemical force (conc difference) push K+ out via leak channels weak electrical force (charge difference) some K+ goes back into the cell due to minute negative anions inside the cell chemical \> electrical K+ leaves cell. As K+ leave intracellular space becomes negative electrical force increases eventually chemical = electrical no net movement of K+, this is equ potential for K+ - E~k+~. **Stages of the action potential** If the stimulus is capable of bringing the membrane potential to the threshold level, an action potential is initiated: 1. Threshold reached voltage gated Na+ channels open influx of Na+ into cell membrane potential is more positive. 2. Depolarisation membrane potential gets more positive so more voltage gated Na+ ion channels open More Na+ influx & depolarisation positive feedback loop. 3. Peak Potential can overshoot in large neurones (passed +0) otherwise peaks at 0. Voltage gated Na+ channels close, voltage gated K+ channels open efflux of K+ ions out the cell. 4. Repolarisation as K+ leave membrane potential drops to RMP where voltage gated K+ channels close. 5. Hyperpolarisation delay in closure of voltage gated K+ channels excessive efflux of K+ out MP gets more negative than RMP but then restored to normal. 6. Na/K pump reestablishes concentration gradients of Na+ and K+, maintain the normal RMP.  **Refractory period** The refractory period is the length of time following an action potential during which a new action potential cannot be generated. This is due to the **prolonged inhibition of voltage-gated Na+ channels**. There are two refractory periods: - **Absolute refractory period** -- in this period of time, a new action potential CANNOT be generated regardless of stimulus strength. - **Relative refractory period** -- in this period of time, a new action potential CAN be generated, but it requires a stimulus which is stronger than normal. **Conduction of action potentials** Conduction of action potentials is different in myelinated and unmyelinated neurones. In **unmyelinated neurones**, the Na^+^ ions entering the cell diffuse in either direction, causing neighbouring Na^+^ channels to open. This sets up local circuits where the action potential propagates in all directions, this is relatively **slow**. Unmyelination - 0.1 meters/sec In **myelinated neurones**, long circuits are set up where action potentials only occur at the unmyelinated **nodes of Ranvier**. This is known as **saltatory conduction**, where action potentials 'jump' between nodes. This process is much **faster** and **more** **energy efficient**. Myelinated - 100 metres/sec The larger the diameter of the axon, the faster the conduction  **[NAS Lecture 4 -- Somatic Nervous System]** **What is The Somatic Nervous System & What is Its Importance?** - It is the part of the nervous system that is under voluntary control of the human organism - Its sensations give us - awareness of the world around us - It allows us to accurately localise sensations throughout the body - It also gives us awareness of our body position and movement in a three-dimensional space (proprioception) **[Overview of The Nervous System]** A diagram of nervous system Description automatically generated - The CNS & PNS are a continuum and not independent systems (neurones exists on both sides) - **Afferent Neurone:** send sensory impulses from receptors in the PNS to targets in CNS (PNS CNS) - **Efferent Neurons:** Send impulses from the central nervous system to your limbs and organs (effectors) located in the PNS. (CNS PNS) - **Autonomic Nervous system:** Not under voluntary control (unconscious -- fight or flight) - Afferent system is simply just a sensory system (sensory neurones). So they don't divide into somatic and autonomic systems. - Only the efferent system does as it only makes sense that effects are voluntary and involuntary. **[CNS]**  - The brain and spinal cord are encased in Meninges - a layer of connective tissue which acts as a membrane around the brain and the spinal cord. It consists of the dura mater, arachnoid matter and the pia matter. - Both the brain and spinal cord are suspended in Cerebrospinal Fluid - This is present in the subarachnoid space (between arachnoid and pia matter) - Finally the superficial layer of the CNS is the Cranium (brain) & Vertebral Column (spinal cord) - The CNS Responsible for the sophisticated functions of the nervous system. **[PNS]** A diagram of several parts of the body Description automatically generated - The nervous system outside of the CNS - It connects the CNS to its target organs & tissues - It connects sensory organs to the CNS **[The neurone (nerve cell)]** - Is the specialised functional unit of the Nervous System - Its main features (template) are: - The Cell body (perikaryon) (n=1) - The Axon (n=1)  **[General anatomy of a neurone]** Diagram of a diagram of a structure Description automatically generated - Axon hillock -- Region where an action potential is generated. - produces 'kink' in neurones -- visible in micrographs - Axon terminal -- end - Myelin sheath -- insulator 🡪 increases speed of transmission - Myelin sheath has gaps at regular intervals which are called nodes of Ranvier - The segments of the myelinated sheath are produced by individual Glial cells. Schwann cells (PNS) and Oligodendrocytes (CNS) - Nodal membrane: Membrane at the node of ranvier - Internodal membrane: Membrane at the regions that are myelinated **[The somatic nervous system]** A screenshot of a computer screen Description automatically generated - Efferent system conducts impulses away from the somatic nervous system - Afferent system conducts impulses towards the somatic nervous system. - **Somatic Efferents** convey commands from the brain to skeletal muscles only (voluntary movements). It manifests its actions as muscle contraction. - **Afferents** carry sensory information from the surface of the body to the sensory brain. Its sensations give awareness to the sensory experience **[Typifying Characteristics of The Somatic Nervous System]** - It can be said to exist in a bi-stable state - Thus, it is either - Active (awake) or - Inactive (sleep) - It is largely paralysed during \[rapid eye movement sleep\] - Its effector organ is the skeletal muscle only - It is responsible for movement (via muscles) and motor tone of the body **[Characteristic Anatomical Properties of Neurones of The Somatic Nervous System]**  **[Somatic Efferent/Motor neurone]** - Dendrites -- very important as they increase the surface area of the cell's membrane. synaptic impulse are directed through these membranes. - Dendrites with **direct** **connection** to the cell body are known as **proximal** dendrites - Dendrites with **no** **connection** to the cell body are called **distal** dendrites - The diameter of the dendrites the lower the cross-sectional resistance to electrical impulses, so the faster the impulse travel - The Cell body has a **multipolar cellular morphology** -- the cell body has a lot of processes radiating in multiple directions - Cell body is located in the central nervous system (Ventral horn of the spinal cord or Cranial nerve motor nuclei of the brainstem) - They are largely myelinated: - Alpha motor neurons (fastest) (main) innervate the highly contracting fibres that supply the muscle with its power. - Gamma motor neurons (slow) innervate slightly contract fibres -- allows muscle to be sensitive to stretch, over a wide range of muscle lengths. **[Somatic Afferents/ sensory neurone]** - The axon splits into two sub axons. - This is a sensory neurone so its electrical impulses are generated at the periphery end which converts electromechanical energy into electrical impulses - The impulses are then propagated along the periphery axon towards the cell body - The cell body receives a copy of the electrical impulse alerting the cell allowing it to respond metabolically to support the axon -- e.g. makes sure nutrition is made available to the axon. The impulse then moves along the axon towards the brain. - Bold cell body -- Axonal terminals on the cell body would interfere with signal generated in the peripheral corrupting the signal. - This morphology is called **pseudo unipolar morphology** (characteristic primary sensory neurones) - one process radiating in multiple directions - Primary sensory neurones: neurones responsible for converting electromechanical energy into electrical impulses. - Cell body is located outside of the CNS they are located in ganglia. - Levels of myelination vary between functional sub-types - Some are myelinated: - Heavily myelinated (alpha) and (Beta) - Lightly myelinated (gamma) - The rest are unmyelinated: - Knows are C-fibres or free nerve endings (largely responsible for pain sensation) A diagram of several neurones Description automatically generated Bipolar cells: This is characteristic of sensory neurones (retina) **[What is A Motor Neurone]** - It is an efferent neurone that supplies - Skeletal muscle - effectors of the somatic nervous system - Smooth muscle - effectors of the autonomic nervous system - Cardiac muscle are myogenic - Role of skeletal muscles: - Bring about displacement of limb (movement) - Set muscle Tone - Taken together motoneurons make up the motor system of the body  **[Anatomy of The Neuro-muscular Junction]** - It is confined to a specific area of the muscle known as the 'nerve entry point' or neurovascular hilum - The nerve entry point is the: - Geographical centre of any given muscle (allows contraction to be centralised) - Site of entry of a motoneuron into the substance of the muscle - Site of entry of arterial supply to a muscle - Site of exit of venous drainage of the muscle - Site of collection of nicotinic receptors of healthy muscles -- that allow for electrical impulses of the nerve to produce contraction of the muscle done with production of neurotransmitter -- Acetyl choline. **[MYELINATION IN THE SOMATIC NERVOUS SYSTEM]** **[Axons of the PNS]** - Are variable in thickness & length - Some are not myelinated - Thin axons - Others are heavily myelinated -Thick axons - The level of myelination varies between neuronal types - Unmyelinated axons are only found on C fibres (responsible for pain sensation) - somatic sensory neurones - All motor neurones are myelinated - Conduction speed of electrical impulses is correlated to the level of axonal myelination - Expressed mathematically as Conduction velocity (CV) =5.8 x fibre diameter (FD) **[Myelinated Axon in X-Section]** - Thickness of myelin varies between neuronal types - Myelin of thin axons can be 0.5 micrometres thick - Myelin of thick axons can be 2.5 micrometres thick - On average, a Schwann Cell wraps itself 100x around an axon - Certain diseases demyelinate axons - Multiple Sclerosis - Diabetes - Guillain Barre - Polyneuropathies - They provide insulation increases the speed of conduction of nerve impulses - Reduces the capacitance of neurones -- makes it easier for the neurone to be activated - If a nerve axon is cut and it dies away, the myelin sheath left behind maintains the tunnel where the old axon ran. This creates a path taken by a new/regenerated axon. **[Neuroglia and PNS]** - Neuroglia of the PNS are: - **[The Schwann Cell ]** - Main Function is myelination of Peripheral Nerve Axons - **1 Schwann Cell myelinates only 1 axon segment ** - **[The Satellite Cells ]** - Physical Support of Neurones in PNS - **[Microglia ]** - (they are macrophages) Immune and Inflammatory functions **[Neuroglia in CNS]** - A Single **Oligodendrocyte** myelinates many axons at many myelination segments in CNS. A diagram of a structure Description automatically generated **[Membranous Envelopes of Cranial & Spinal Nerves]** **[From out to within]** - **Epineurium ** - sheaths the entire nerve - interfascicular bands attach adjacent nerve fascicle - **Perineurium ** - Axons with common origins and common destinations will travel together forming a nerve fascicle. - Perineurium ensheaths a nerve fascicle - **Endoneurium ** - ensheaths a single cell's axon - Gives it structure and isolates it A diagram of the structure of the spinal cord Description automatically generated  **[NAS Lecture 5 -- Synapses and Neurotransmitters]** **[Synapses and the role of Neurotransmitters]** **[NB: Concept]**: A single action potential results in the production of a single EPSP (excitatory post synaptic potential). Sometimes a single EPSP is strong enough to induce an action potential in the postsynaptic neuron, but often multiple presynaptic inputs must create EPSPs around the same time for the postsynaptic neuron to be sufficiently depolarised. This occur via spatial or temporal summation. **EPSP:** the voltage produced solely by neurotransmitters binding to receptors on postsynaptic membrane. **[Neuronal specialisations for communication]** - Dendrites - form the dendritic tree here lots of information from other neurones is fed into which controls the excitability of a neurone - Axon hillock - Region where an action potential is generated and then propagated along the axon - Axon terminal - release of neurotransmitter at the synapse **[Synapses]** Site of communication between two neurons, two cells or a cell (muscle cell) and a neuron **[The electrical synapse (gap junction)]** - Fastest and most primitive form of communication - The gap junction is a physical point of contact between two adjacent cells via their cell membrane. - Important in glia-neuron and glia-glia communication - Also, cardiac myocytes are connected via gap junctions - allows cardiac muscle to depolarise to cause contraction - Mechanism: - Connexons (protein) line up physically connecting the two cells by having half of each connexon in either cell. - There is a pore in the connexon allowing the passage of ions - Information (ions) can travel in **both directions** - allows synchronous activity between cells - They are relatively rare between neurons in the CNS, although important in the developing CNS A diagram of a cell structure Description automatically generated **[The chemical synapse]** - Uni-directional transfer of information from pre-synaptic post synaptic cell - Plenty of vesicles to allow for the transport of the neurotransmitter. - **Synaptic** **Cleft**: Space that separates two neurones  **CRITICAL PROCESSES OF NEUROTRANSMISSION** +-----------------------------------+-----------------------------------+ | A diagram of a nerve cell |  | | | | | - NB: No physical connection | 1. Action potential (initiated | | | in the cell body) propagates | | | down the axon, invading the | | | nerve terminal | | | | | | (The AP is dependent on membrane | | | depolarisation which is dependent | | | on the influx of Na+ through | | | voltage-gated Na+ channels) | +===================================+===================================+ | A screenshot of a computer |  | | | | | 2. Depolarisation at the nerve | 4. Vesicle will move and fuse | | terminal triggers a | with the membrane of the | | conformation change in Ca^2+^ | presynaptic terminal -this | | voltage gated ion channels | portion is called the active | | causing them to open | zone | | | | | | | | | | | 2. Ca2+ influx into the nerve | 4. Docks with the membrane and | | terminal triggers the release | the vesicle contents | | of neurotransmitter | (neurotransmitter) are | | | released into the synaptic | | | cleft via exocytosis | +-----------------------------------+-----------------------------------+ | A screenshot of a computer screen |  | | 6. Neurotransmitter diffuses | | | across the synaptic cleft | 8. A signal is communicated and | | (down its concentration | this causes depolarisation | | gradient) | EPSP in post synaptic neurone | | | lead to AP. | | | | | | | | 6. Neurotransmitter then binds | | | to the receptor on the post | 8. Signal must be quickly | | synaptic membrane. | terminated after the signal | | | is processed. The mechanism | | The receptor is very specific so | to do this, depends upon: | | only one type of neurotransmitter | | | will bind to it. | - the synapse itself and what | | | neuron is involved | | | | | | - the neurotransmitter released | +-----------------------------------+-----------------------------------+ | A diagram of a neurotransmitter |  | | | | | Mechanism 1: The neurotransmitter | Mechanism 2: | | is taken back up into the nerve | | | terminal by a specific | Here mitochondria are in the | | re-uptake | extracellular fluid. | | mechanism. This is done rapidly: | | | | 1. Enzymatic activity in the | | 1. Protein on the cell surface | extracellular fluid breaks | | membrane (re-uptake sites) | down the neurotransmitter | | transport neurotransmitter | released in the synaptic | | back in pre-synaptic | cleft without reuptake | | terminal. | | | | 2. This mechanism is used to | | 2. Once the neurotransmitter is | terminate signalling at | | taken up, it can be broken | certain synapses e.g. those | | down by enzymes present in | that use **acetylcholine** | | the presynaptic membrane. | | | Many (not all) of the enzymes | | | which break down the | | | neurotransmitter are | | | expressed via the | | | mitochondria | | | | | | 3. However, it can also be | | | re-packaged into vesicles | | +-----------------------------------+-----------------------------------+ **Neurotransmitters:** chemical messengers at the synapse **[Major neurotransmitters]** - **Acetylcholine (ACh) (at NMJ)** - Amino acids: - glutamate (most abundant excitatory NT in the CNS) - does most of the brain activity involving stimulation - GABA: (gamma aminobutyric acid) most abundant inhibitory NT - Glycine - Monoamines: - noradrenaline - dopamine - Serotonin (5-hydroxytryptamine 🡪 5-HT) - Neuroactive peptides: - More than 100 different kinds e.g. opioid peptides (endorphin, enkephalin) tachykinins (Substance P, neurokinin A) **[Receptors]** - Recognition site for a neurotransmitter - It a membrane spanning protein expressed by the cell membrane on the post synaptic neuron - It binding site stick out into extracellular compartment whilst the intracellular domain is related to signalling cascades - It initiates an intracellular signal (depolarisation) via changing its conformation - Receptors are specific for a single neurotransmitter - a neurotransmitter can bind to many different receptors whereas one receptor can only have one specific neurotransmitter binding to it i.e. one neurotransmitter - several receptor subtypes - Man-made material and plant based material (exogenous substances) can also mimic molecules and bind to receptor sites **[Receptor signalling mechanisms -- 2 types at a synapse]** [1 -- Ionotropic - (Receptor operated gated ion channels)] [1a) Excitatory Synapse] 1. An excitatory Neurotransmitter binds to the receptor 2. This causes a conformational change in the Na+ ion channels causing it to open 3. This allows influx of Na+ (due to ionic driving force) causing depolarisation 4. This causes small excitatory post synaptic event (see in the graph) (EPSP) (Doesn't last long as the Na+ is pumped out after). 5. So **excitatory neurotransmitters** cause excitation in the post synaptic neuronA black background with white text Description automatically generatedA diagram of a person\'s hand Description automatically generated [1b) Inhibitory Synapse] 1. An inhibitory neurotransmitter is released and interacts with a specific receptor 2. This causes conformation change in Cl^-^ ion channel 🡪 opens 3. This causes an influx of Cl-ions into the cell 4. The movement of Cl-is due to its concentration gradient (against electrical attraction) 5. This causes membrane hyperpolarisation 6. The membrane potential becomes more negative than the resting membrane potential = small inhibitory post synaptic potential (IPSP) \ \ A diagram of a person\'s body Description automatically generated **[2 -- Metabotropic - (G -protein coupled Receptor)]** When neurotransmitter binds: 1. Conformational change in the receptor protein itself 2. This activates a G-protein -- this is tethered on the intracellular side of the membrane 3. The G protein then activates 'effector systems' 🡪 this can be excitatory or inhibitory depending on the particular receptor and G-protein 4. Slower transmission but longer lasting effects on the excitability of the post synaptic cell An activated G protein can affect different potential effector systems: - Open or close G-protein gated ion channels which allows for less/more ion movement changing the excitability of the cell - stimulate or inhibit enzymes/ secondary messenger systems which can switch on/off an enzyme cascade E.g. ACh muscarinic receptors; GABA~B~ receptors; monoamine receptors (except 5-HT~3~) One neurotransmitter can bind to multiple subtypes of receptors which can be either Ionotropic/Metabotropic 🡪 so a neurotransmitter can cause different effects on the same cell at the same synapse if multiple types of receptors are expressed at that synapse. \*can have fast and slow transmission by same neurotransmitter **[Synaptic integration of information]** - Each neuron has thousands of synaptic contacts on it, which all control the excitability of this one neurone. - Therefore, a single cell must integrate all of this information and decide what to do. - NB: A neurone has inputs coming in along its entire surface area (red dots = synapse): A close-up of a nerve cell Description automatically generated **[Spatial Summation]** [Excitatory Synapse ] 1. **One small EPSP is not enough for the threshold value to be reached.** 2. **Spatial Summation:** summing of post synaptic potentials generated at separate synapses 3. Enough simultaneous inputs will cause the membrane potential to reach the threshold value (causing VG Na+ ion channels to open 🡪 Na+ influx) resulting in the generation of an action potential. 4. An input closer to the axon hillock will have a much larger effect on the excitability of the cell compared an input on the dendritic tree. A diagram of a nerve cell Description automatically generated\ \  [Mixed Synapse ] 1. At a mixed synapse you can have both inhibitory and excitatory sypatic imputs 2. An excitatory and inhibitory input, coming in at the same time can lead to no change at all for the excitability of the cell 🡪 and membrane level of polarisation remains the same. 3. If the level of the excitatory input = level of the inhibitory input, then they cancel each other out. A diagram of a nerve cell Description automatically generated **Temporal summation** 1. Summation over time 2. Single synapse repeatedly stimulating the cell (with a small gap in between) 3. In this situation one EPSP is quickly followed by another, so they can sum up because each one does not have time to decay away before another adds on top 4. This can result in the threshold value being reached and an action potential being generated 5. If EPSP are too slow, the first one will decay away!  A white line drawing of a green and black cell Description automatically generated  A white line drawing of a green and black cell Description automatically generated  **[NB:]** Inhibitors do not cause the opposite effect but stop the effect. **[Neuronal Circuits ]** You have met the basic principles of synaptic neurotransmission, including the nature of the different neurotransmitters and how they are released and bring about their effects on the postsynaptic cells. This activity builds on these principles using some simple theoretical circuits to illustrate the points. The following diagrams are highly stylised; you should assume that all inputs are equally weighted. [Part A.] With reference to the diagram: 1. At time zero, all neurones are inactive, A is excitatory, B is excitatory. What happens to the activity of C if A becomes active? Increases 2. At time zero all neurones are inactive, A is excitatory, B is inhibitory. What happens to the activity of C if A becomes active? Decreases 3. Decreases [Part B] 1. At time zero, A, B and C are inactive but D is firing. A is excitatory, B is inhibitory, C is inhibitory. What happens to the activity of D if A becomes active? Stays the same 2. Increases 3. Increases [Part C] 1. At time zero, A and B and D are inactive, C is active. A, B and C are excitatory, D is inhibitory. What will happen to D if A becomes active? What then happens to B? Increases Decreases **[NAS Lecture 6 -- Properties of Motor Units]** **[The Motor System of The Body]** - It is built from: - Motoneurons (Neuronal tissue) - Skeletal Muscles (Muscular tissue) - The Neuromuscular Junction (where the neurone and muscle connect -- specialised synapse). - Connective tissue - They work together to: - Set muscle tone of the body -- a small amount of sustained muscle contraction responsible for body posture - Bring about voluntary movement via antagonistic muscle pairs **[Definition of a motor unit]** - **Motor unit:** The minimal functional unit of the motor system. It consists of a somatic efferent (alpha-motoneuron) and all the extrafusal muscle fibres it supplies e.g. - extra-ocular muscles (1 neurone :10 fibres) - quadriceps (1 neurone:1000 fibres) - **Innervation Ratio:** The number of muscle fibres innervated by a single motor neurone. - Generally, the smaller the innervation ratio the more precise the movement. A blue background with yellow text Description automatically generated **[Where the motoneurons originate from in the nervous system ]** A diagram of nervous system Description automatically generated (Follow the arrows) **[Muscles]**  - Striated and non-striated muscles are both made of muscle tubulars. - In Striated however the tubulars are arranged in a parallel formation. - In non-striated they run in every direction. - Both cardiac and smooth muscle are myogenic -- generate their own electrical activity 🡪 ANS only controls rate & strength of contraction **[The Importance of Skeletal Muscle]** - Set muscle tone of the body -- a small amount of sustained muscle contraction responsible for body posture - Allow for voluntary movement via antagonistic muscle pairs - Stabilises joints - Generate heat -- mechanical efficiency of skeletal muscle is 20% so 80% is lost by heat. **[GENERAL ANATOMY OF SKELETAL MUSCLES]** **[The Myofibril, The Fascicle & Muscle]** - The single cell of muscle is known as a myocyte 🡪 muscle fibre - Myofibrils are the most abundant organelle within them - Myocytes collect together form a fascicle - The neurovascular bundle (blood vessels) of any muscle runs in between and in parallel with muscle fascicles - Fascicles collect to form a muscle Image result for myocyte myofibril muscle \| Anatomy and physiology, Biology class, Physiology **[Tissue envelopes of skeletal fibres]** - **Epimysium** -- CT enveloping all fascicles - **Perimysium** -- CT enveloping a fascicle - **Endomysium** -- CT enveloping each muscle fibre **[Importance of tendons]** - A tendon is a tough band of fibrous connective tissue - Attaches muscle to bone - It forms a point of confluence, bringing together the individual contractions of the myocytes to produce a combined action at a usually a joint. **[Types of skeletal muscles]** Skeletal muscle can be classified according the assemblies of their fascicles: - **[convergent/triangular]** -- muscle fibres converge to a small point on a tendon 🡪 allows for very strong contraction (i.e. pectoralis major) - **[circular/sphincteric]** - surround openings at orifices (i.e. orbicularis oris) - **[parallel/strap]** -- fibres run parallel to each other 🡪 these are long muscles that cause large movements 🡪 not strong but good endurance (i.e. sternocleidomastoid) - **[fusiform]** -- spindle-shaped 🡪 belly wider than points of origin & insertion (i.e. biceps brachii) - **[Pennated Muscles]** - **[unipennate]** -- fibres diagonal to tendon 🡪 allows great strength (i.e. lumbricals) - **[bipennate]** -- bilateral fibres run in opposing diagonals to central tendon 🡪 more power but less motion range (i.e. rectus femoris) - **[multipennate]** - multiple rows of diagonal fibres, with a central tendon which branches into two or more tendons 🡪 produce powerful contractions (i.e. deltoid muscle w/ anterior, posterior & middle sections) A diagram of a person\'s body Description automatically generated **[How Muscles Contract:]** - **Actin** --- the thinner filament. They are connected to the Z line (see below). Actin filaments have binding sites for myosin heads. These are called actin---myosin binding sites. When a muscle is relaxed the actin---myosin sites are blocked by tropomyosin. - **Myosin** --- the thicker filament. Myosin filaments have globular heads that bind to the actin binding sites. - **(Sarcoplasm:** the shared cytoplasm within a muscle fibre.) Myofibrils have alternating light and dark bands due to the varied overlapping of actin and myosin A close-up of a graph Description automatically generated  **[How muscle contraction occurs (sliding filament model)]** 1. There are many neuromuscular junctions along the length of a muscle to ensure that all the muscle fibres contract simultaneously ensuring a powerful muscle movement. 2. Muscle contraction is triggered when an action potential arrives at a **neuromuscular junction** --- the point where a motor neurone and a skeletal muscle fibre meet. 3. When an action potential reaches the neuromuscular junction, it stimulates the release of Acetylcholine. 4. Acetylcholine binds to receptors on the postsynaptic membrane (the sarcolemma), opening sodium ion channels, and resulting in depolarisation. 5. Acetylcholine is then broken down by enzymes preventing the muscle being overstimulated. 6. When the action potential reaches the sarcoplasmic reticulum in the sarcoplasm it stimulates calcium ion channels to open. 7. The calcium ions diffuse down their concentration gradient flooding the sarcoplasm with calcium ions. 8. The calcium ions bind to troponin causing it to change shape. 9. This pulls on the tropomyosin moving it away from the actin---myosin binding sites on the actin filament. 10. Now that the binding sites have been exposed the myosin head binds to the actin filament forming an actin---myosin cross-bridge. 11. Once attached to the actin filament the myosin head flexes, pulling the actin filament along. The molecule of ADP bound to the myosin head is released. 12. An ATP molecule can now bind to the myosin head. 13. This causes the head to detach from the actin filament. 14. The calcium ions present in the sarcoplasm also activate the ATPase activity of the myosin. This hydrolyses the ATP to ADP and phosphate, releasing energy which the myosin head uses to return to its original position. 15. The myosin head can now attach itself to another actin---myosin binding site further along the actin filament and the cycle is repeated. 16. This is repeated up to 100 times per second. 17. The cycle continues as long as the muscle remains stimulated. 18. During the period of stimulation many actin---myosin bridges form and break rapidly, pulling the actin filament along. 19. This shortens all the sarcomere in the myofibril and causes the muscle to contract. Diagram of a diagram showing how the structure is attached to the wire Description automatically generated with medium confidence  **[PROPERTIES & FEATURES OF MOTONEURONES]** - 2 motoneurone in a series arrangement are hypothesised - Upper motoneurone - Lower motoneurone - (you will be expected to understand differences between upper & lower motoneurone) A diagram of a motor cycle Description automatically generated **[Lower motoneurons]** - Lower motor neurones are the same thing as alpha motor neurones - It's cell body is in either: - Lamina IX of the grey matter of the spinal cord - Cranial nerve motor nucleus 🡪 grey matter of the brain stem - Its axon supplies skeletal muscles of the body directly - Upper motor neurones command lower motor neurones to bring about contraction. They do not have access to skeletal muscle.  **[Characteristics of The Motor Unit]** - The muscle fibres of a single motor unit are randomly distributed throughout the thickness of a muscle. This allows for: - Muscle contraction to be distributed uniformly throughout the muscle which allows for smoother movement (all parts are contracting not just a small section concentrated with muscle fibres if the structure was not random) - The nervous system to regulate the rate of contraction. **[Characteristics of muscle fibres of a motor unit]** - Each muscle fibre is innervated by only 1 motoneurone - All the muscle fibres of a single motor unit have the same physiological profile - same contraction speeds - same susceptibility to fatigue - They have the same histochemical profile - Same myosin fibre types - Same enzyme expression profile 🡪 same Metabolic profile A screenshot of a cellphone Description automatically generated [ ] All black myocytes are innervated by same motor neurone etc. **[Twitch characteristics of muscle fibres]** - A twitch is a brief contraction of a muscle in due to electrical stimulation - Muscle fibres can be classified into 3 categories according to: - How quickly they develop force - How long they take to relax Profile of a twitch:  (Y axis is Force measured with strain gauge) - 3 Broad classes of twitch fibres (myocytes): - NB: All twitch fibres belonging to a single motor unit at the same type - Fast twitch (Type FF): Muscle develops force at a high speed but fatigues very quickly (shown by width of curve) (e.g. in eye) - Intermediate Twitch (Type FR): Muscle develops force at a medium speed and fatigues at a moderate rate (shown by width of curve) (Gastrocnemius) - Slow Twitch (Type S): Muscle develops force at a slow speed but fatigues very slowly (shown by width of curve) (soleus) A diagram of a normal distribution Description automatically generated **[Summation of twitches ]**  1. When you increase the frequency of twitches summation occurs and you get a tetanus. 2. The Tetanus contraction is sustained and smooth. 3. Our body stimulates muscles at a very high frequency meaning summation occurs much more quickly removing the ups and downs resulting in a smoother contraction. **[Motor Unit Properties]** - The motor neuron determines the characteristics of the muscle it innervates - This was worked out in an experiment where you took two motor units. One which had a type S motor neurone and a type S muscle and another with a Type FF motor neurone and a type FF muscle. - The motor neurones were switched (i.e. S motor neurone connected to FF muscle). - The Muscles changed their twitch properties and biochemistry and conformed to the same muscle type of the neurone connected. A diagram of different types of motorene Description automatically generated - lower motor neuron = involuntary - upper motor neuron = voluntary **[NAS Lecture 7 -- The Neuromuscular Junction]** **[The Neuromuscular Junction -- a specialised synapse]** - The junction between a motor neuron and a myocyte (muscle fibre) - There are many neuromuscular junctions along the length of a muscle (curved shape) to ensure that all the muscle fibres contract simultaneously ensuring a powerful muscle movement. - It allows for rapid and reliable neurotransmission - The strength of synaptic transmission at each NMJ virtually guarantees that all muscle fibres contract rapidly in response to every single action potential conducted. Diagram of a cell structure Description automatically generated  (Ventral horn is where the cell bodies are located) **[Role of Ca2+ in excitation-contraction coupling]** - Ca2+ conc greater intraceullularly compared to outside - Action Potential in presynaptic terminal - Depolarisation opens voltage gated Ca2+ channels - Ca2+ influx into presynaptic terminal (down concentration gradient) - Ca2+ entry into presynaptic terminal triggers vesicle fusion with presynaptic membrane - Ca2+ sensor is a synaptotagmin -- it changes its conformation on Ca^2+^ binding to it which triggers vesicle fusion - Neurotransmitter released (e.g. Ach) by exocytosis Diagram of a cell membrane Description automatically generated **[Acetylcholine: neurotransmitter at end plate]** - The only receptor at the post synaptic membrane of the neuromuscular junction is the Nicotinic Acetylcholine receptor - ACh binds to nicotinic ACh receptors (ligand-gated ion channel) on postsynaptic membrane - Ligand gated ion channel: When ACh binds a conformational change occurs which opens the pore to allow ion movement - 5 Subunits of proteins - Two binding sites for ACh: So two molecules of ACh bind to confirmational change \ A circular chart with numbers and letters Description automatically generated **[Generic synapse]** 1. **An excitatory neurotransmitter is released** and will bind to an excitatory ionotropic receptor This could be an ACh nicotinic receptor 2. 2 molecules of ACh binding to the receptor causing Na+ influx and membrane depolarisation 3. Small excitatory post synaptic potential **[NMJ -- SPECIALISED SYNAPSE]** **Pre-synaptic neurone** - Lots of mitochondria - More vesicles containing neurotransmitter than any other synapse in the CNS - Multiple active zones for release of large amounts of neurotransmitter simultaneously - One action potential causes the release of 200-300 quanta, all releasing their 4000 molecules of ACh each (much more than a generic synapse) **Postsynaptic neurone** - Junctional folds - Very high density of nACh receptors - high density of voltage gated Na+ channels  NB: Motor end plate is equivalent of post synaptic neurone in a generic synapse. Depoliarisation in NMJ greater than in generic post synaptic receptors **[Generating an action potential at NMJ]** - **End plate potentials:** the voltage produced solely by ACh binding to nAChR on motor end plate. They result in an action potential being generated. - **Note:** EPP and an Action potential are two separate events. AP produce by opening of VG Na^+^ ion channels - The resting membrane potential at the motor end plate is -90mV compared to that of a neuron which is -65mV. - A lot of ACh released 🡪 ACh binds to nAChRs on postsynaptic end-plate membrane (2 ACh molecules required to activate receptor) - Thousands of nAChR receptors are activated 🡪 channel opens -- permeable to both Na^+^ & K^+^ - Na^+^ influx is much greater than K^+^ efflux - This results in a 70mV worth of depolarisation, and the end plate potential changes from -90 to -20mV (peak). - This massively exceeds the threshold value so VG Na+ ion channels are able to open (more Na+ influx) 🡪 generate an action potential. - This makes the synapse highly reliable i.e. will cause an action potential **[Fate of Ach]** - ACh binds to nAChR for \~ 1 ms - Released back into synaptic cleft - hydrolysed by Acetylcholinesterase (AChE) into choline and acetate. - Choline can then be taken up by the presynaptic neuron and used to make more ACh. A diagram of a cell membrane Description automatically generated **[EPP (end plate potential) vs AP (action potential)]**  - EPP initiates an action potential in muscle (\>threshold) so 2 different electrical events. - EPP decays as you move further away from the motor end plate. Smaller EPPs are being generated as density of nAChRs receptors decreases as you move from the synpase. - However VG Na+ ion channels are present all across the membrane of the muscle fibre - Therefore the size of action potentials remain constant as you move away from the motor end plate. Red -- EPP Blue -- action potential **[EPP -- Final notes]** - Timing: Pre-synaptic AP to EPP generation in post-synaptic membrane \~ 1 msec - EPP is very large (compared to most synaptic potentials e.g. EPSP) -- Many ACh vesicles released; high density of nAChRs -- threshold easily reached. - EPP generated by ligand-gated channels -- opening voltage-gated channels (i.e. action potential). **[The postsynaptic action potential]** - Action potential invades T (transverse) -tubule system. - The T- tubules allows the transmission of the AP to move away from the motor end plate and to separate myofibrils to cause contraction - Sarcoplasmic reticulum is where the calcium needed for contraction is provided from in response to depolarisation **[Ca2+ release from the SR]** - AP moves down into T-tubules from the motor end plate - The DHP receptor is tethered to the ryanodine receptor (located Ca2+ release channel). - Depolarisation of the membrane causes activation of this DHP receptor - The DHP receptor undergoes a conformation change which activates the ryanodine receptor and causes it to be removed from the sarcoplasmic reticulum. This causes opening of the calcium release channel. - This allows calcium out of the SR which then causes contraction -- by the sliding filament mechanism. - Repolarisation of the membrane, the Ca2+ releasing channel becomes closed - Preventing more release of calcium - And calcium which has been released is pumped back into the SR Diagram of a diagram showing a method of reciprocating reaction Description automatically generated  **[Motoneuron activity leads to muscle contraction]** - A single AP down an axon will cause a twitch. - This can be measured by measuring muscle tension and motor neuron activity. - Action potentials need to be fired at a frequency of 40 hertz (allow enough summation) to produce a sustained contraction. **Summation of Twitches** A diagram of a physical activity Description automatically generated **[Summation of twitches ]**  1. When you increase the frequency of twitches summation occurs and you get a tetanus. 2. The Tetanus contraction is sustained and smooth. 3. Our body stimulates muscles at a very high frequency meaning summation occurs much more quickly removing the ups and downs resulting in a smoother contraction. **[Disease which affects NMJ: Myasthenia Gravis]** - affects 1:200,000 - autoimmune disease of nAChR - reduced number of nAChR at at NMJ 🡪 the synapse fails, becoming unreliable - Causes muscle weakness during a sustained activity - Affects (fatigues) those muscles which are most used e.g. skeletal eye muscles used in oculomotion 🡪 early sign is eyelid dropping and vision dropping. - Improves with rest and is better in the morning - Treatment is AChE inhibitors - blocks the enzyme breaking down ACh, so ACh lasts for longer time stimulating, amplifying activity at the synapse which prolongs signal - Medication: neostigmine (contains inhibitor) **[Muscle firing and Tension in response to nerve stimulation (Red = AP) (Muscle tension Blue)]** A diagram of a diagram of a muscle firing Description automatically generated with medium confidence(don't need to remember image) **[NAS Lecture 10 -- Degeneration & Regeneration of Peripheral Nerves Following Trauma]** The nervous system expresses its actions through one of three permutations: **Neurones supply:** - Skeletal muscles -- via somatic nervous system - Smooth muscles -- via the autonomic nervous system - Glands -- via the autonomic nervous system - Sensory brain -- via afferents that originate in the periphery and special sense organs. In all of these cases, the brain communicates with effectors of the somatic or autonomic divisions through relay chains of neurones that we call descending pathways. Causes for degeneration of nerves are multiple: Genetics of the body like motor neurone disease Natural process of aging Acquired causes through injury Acquired toxic infection affecting nerves (Also diseases like multiple sclearosis) A diagram of neurology Description automatically generated Defnitions: If a neurone or its immediate enviromentmt are disturbed or interfered with, function of the neurone might be disrupted The neurone might thus dysfunction as a result The extent of dysfunction and its severity often depend on the nature of the unsult to the neurone (or its environment)  **[Seddon's classification of nerve damage]** In terms of depth, the damage to the nerve due to an injury can be classified into 3 categories: - **Superficial** -- Damage to the epineurium layer (ensheaths the entire neurone and bands interfasical bands attach to nerve fascicles) - **Intermediate** -- Damage to the perineurium layer (ensheaths a nerve fascicle -- a fascicle is a collextion od axons wirth common origion and destinations). - **Deep** -- Damage to the endoneurium layer, and possibly its associated axons (ensheaths a single cells axon). Superficial and intermediate injuries are likely to heal naturally relatively quickly. In terms of damage to the axon, injuries can be classified into 3 further categories: - **Neuropraxia** -- Temporary loss of a neurone's function, usually due to damage no deeper than the level of the myelin sheath (e.g. compression of nerve fibre leading to loss of blood supply to axon). Complete recovery of neurone function is **likely**. - **Axonotmesis** -- Damage to axon of the neurone, this could cause axon to be split into two. Reinnervation of effector organ is **likely**, as long as superficial layers (endoneurium, perineurium and epineurium) remain intact. New nerve sprouts would use the ensheathing membranes to resuplly the target effector to restore normal function. - **Neurotmesis** -- Complete transection (cut) of nerve fibre, including axon and the superficial layers. Function of nerve fibre **cannot** be recovered. Its most severe class of nerve damage.  **Reactions to injury in axonotmesis** **[Reaction of neurone]** When the axon gets damaged (axonotmesis), the neurone is divided into two segments: - **Proximal segment** -- The segment that remains associated with the cell body (survives the injury and continues to get support of cell body). - **Distal segment** -- The segment that is not attached to the cell body (often cut off, loses potential for repairs and nutritional support, it becomes vulnerable to phagocytosis by glia). When neurones are injured how does their local envuonment change? The behaviour o 3 classes of glial cells is critical Myelin forming cells -- oligodendeocttes and schwaan cells Astrocytes -- create an environment in which neuroens thrive Microglia -- these are rudimentary immune cells of the nervous system. **[Fate of distal segment]** Immediately after injury, the distal segment stops conducting action potentials, and begins to leak intracellular fluid. The open end begins to seal itself and swell. Minutes after injury... synaptic transmission off, cut ends swell. An hour or so after injury... synaptic terminal degenerates -- accumulation of neurofilaments, vesicles. Astroglia surround terminal normally -- they react by causing terminals to be pulled away from postsynaptic cell. In the hours following the injury, the synaptic terminal of the distal segment is degenerated by the action of astrocyte cells. In the days following the injury, the leftover distal stump undergoes **Wallerian degeneration**, dying due to lack of nutrition and being digested by phagocytes.  **[Wallerian Degeneration]** - Axon and myelin sheath of distal segment are both broken down. - Phagocytic action to clean up the mess.**\ \ **A diagram of a nervous system Description automatically generated **[Fate of proximal segment]** Immediately after injury, the proximal segment stops conducting action potentials, and begins to leak intracellular fluid. The open end begins to seal itself and swell. In the days after the injury, the segment undergoes **chromatolysis**: - Nissl bodies are displaced, triggering the activation of cellular repair - Cell body actively produces proteins to repair cell. - Volume of cell body increases due to new proteins synthesised. - Nucleus moves to peripheral part of cell body. - The axon begins to grow nerve sprouts (some find schwann cell tubes) to try and reinnervate effector organ - **If nerve sprouts reach the effector organ, function soon returns.** **[Regeneration after Axonotmesis]** - Macrophages release mitogens, inducing Schwann Cells to repopulate - Schwann cells make laminin (a substance which helps guide and regenerate axons) - Macrophages also release interleukins which cause Schwann cells to release **Nerve Growth Factor** (making distal segment grow) - Endo-,Peri- and Epineurium also provide guidance for nerve sprouts as they try and find Nerve Growth Factors **[Reaction of skeletal muscle]** In the case of somatic motor units, the damage to lower motor neurones (or a-motoneurons) leads to denervation of the skeletal muscle it innervated. Reinnervation is usually attempted by the proximal segments of the neurones, this may or may not be successful. Deprying the muscle of its nerve supply is denervation Re-innervation is regrowth of the nerve to resupply the muscle **[Switching muscle types]** Slow and fast (FF or FR) muscles are innervated by different types of motoneurons (Type S or Type FF/FS). If one type of motoneuron is damaged, and it regrows to innervate a different type of muscle, this leads to the muscle itself switching to match the type of motoneuron connected. For example, if a Type S motoneuron denervates its S muscle fibre and incorrectly reinnervates a Type FF muscle fibre, the type FF muscle fibre will switch to a type S muscle fibre in response. **[Fate of non-reinnervation ]** As a muscle awaits reinnervation, it enters an acute phase of muscle damage. **Acute phase:** 1. Muscle is paralysed and is **areflexic** (does not display reflexes). 2. Muscle becomes **atonic** (relaxed) 3. Muscle begins to **fasciculate** (involuntarily contract) If the muscle is not reinnervated, the muscle progresses to the chronic phase. **Chronic phase:** 1. Fasciculations (involuntary contractions) cease. 2. **Denervation** **atrophy** occurs (muscle loses bulk and is broken down). 3. Muscle dies. 4. Muscle undergoes **fibrosis**. Muscle is replaced by connective tissue (e.g. adipose) **[Rhabdomyolysis ]** This is a life-threatening complication caused by the breakdown of skeletal muscle and releasing of its intracellular components into the blood. This leads to acute renal failure. This could be caused by trauma to muscle, such as vehicle accidents, over-exercise, and injected harmful substances and certain pathology of muscle damage. **[NAS Lecture 12 -- Simple Reflex]** Reflexes are quick, involuntary actions that occur as a response to a stimulus. Reflexes are found in the somatic (~~voluntary~~) and autonomic (~~involuntary~~) nervous system (somatic can be involuntary too). Neuronal reflexes are **stereotyped,** **involuntary** (without conscious thought) reactions of the CNS to specific **sensory** input. Reflexes occur through reflex arcs, which consist of: 1. **Sensory receptor** -- detects stimulus 2. **Afferent limb** (sensory neuron axons) -- carries information (stimulus) to CNS 3. A **central component** (interneuron ~~relay neurone~~ or synapse). Integration centres are in spinal cord or brainstem nuclei. *Enteric NS in gut.* Modulation: input and output 4. **Efferent limb** -- causes motor response in effector organ (skeletal muscle, smooth muscle or gland). They innervate effectors. 5. **Effector**. Glands, all types of muscles for an appropriate response. **Functions of reflexes** - **Protective --** e.g. limb withdrawal, cough reflex, skeletal muscle reflexes to protect from tears - **Co-ordinated activity** -- e.g. postural control such as walking, typing, writing - **Homeostasis** -- e.g. regulation of blood pressure **Simple stretch reflexes (myotatic)** - Important for posture - Adjust degree of muscle contraction - Sensory receptors = collectively known as proprioceptors (muscle spindles oe golgi tendons) **Muscle spindle** -- no interneurons. If the sensory neurone synapses directly with the motor neurone, the reflex is known as a **monosynaptic** reflex. **[Mechanism 1 of Simple Stretch Reflex mediated via muscle spindle]** 1. Muscle is stretched and elongated (e.g. by a weight) which stretches the muscle spindle 2. This generates an action potential (if the stimulus is large enough to reach the threshold value) which travels along to the axon terminal. 3. At the synapse the sensory neurone will release excitatory neurotransmitter. 4. This excites the motor neurone (increases motor neuron activity) 🡪 action potentials travel along the motor neuron back towards the muscle. 5. When the AP gets to the NMJ the alpha motor neurone releases ACh 6. This leads to the skeletal muscle contracting. 7. Contraction prevents damage 🡪 prevents tearing of muscle (by the heavy weight) by resisting the stretch. This mechanism also allows us to pick up heavy objects. **Excitation vs inhibition of motoneurons** The response to a stimulus in the case of a reflex can either be to excite a certain motoneuron (stimulating contraction of skeletal muscle, for example) or to inhibit the motoneuron. This is done by excitatory or inhibitory interneurons. - Excitation is achieved by release of **excitatory** **neurotransmitters**, such as **ACh** into the synaptic cleft, generating an action potential in the motoneuron. - Inhibition is achieved by release of **inhibitory** **neurotransmitter**, such as **GABA** into the synaptic cleft, inhibiting the generation of an action potential in the motoneuron. Synaptic Transmission \| BioNinja **Skeletal muscle reflexes** **Stretch reflex** A stretch reflex is a motor response that occurs as a result of **stretching** of a muscle, which is detected by **muscle** **spindles**. The activation of muscle spindles sends a signal down the reflex arc to initiate the response. The response involves: - Contraction of agonist muscle(s) via excitation of motor neurones by an excitatory interneuron. - Inhibition of contraction of antagonist muscle(s) due to inhibition of motor neurones by an inhibitory interneuron. An example of this is the **knee** **jerk** **reflex**. Striking the patellar tendon with a reflex hammer leads to stretching of the quadricep muscle. This stretching is detected by muscle spindles, which send a signal down the reflex arc, eventually stimulating: - Quadricep contraction (kicking) via motor neurones. - Inhibition of hamstring contraction as they are the antagonistic muscles of this movement. Other examples include the **jaw** **jerk** **reflex**. This reflex aims to prevent muscle damage due to stretching. **[Mechanism 2 of Simple Stretch Reflex mediated via muscle spindle -- Knee jerk reflex]** - Useful in postural control - The muscle spindle is the sensory receptor (in quadricep) - Sensory neurone connects to both a motor neurone and interneuron (which then connects to another motor neurone) - Stimulus: activate this reflex by tapping below the patella -- hits the tendon - **[Mechanism:]** 1. The tendon being hit causes stretching of the quadriceps muscle which causes the stretching and therefore activating of the muscle spindle 2. This causes an action potential to be sent to spinal cord 3. The sensory neurone releases excitatory neurotransmitter at both synapses. 4. Excitatory neurotransmitter (ACh) at the α-motor neurone will cause action potentials to be sent to quadricep causing contraction. 5. Excitatory neurotransmitter at the interneuron causes the interneuron to releases inhibitory neurotransmitter stopping/reducing action potentials being fired by the hamstring α-motor neurone causing the hamstring to relax. 6. This simultaneous contraction and relaxation allow for extension at the knee. 7. This causes myotatic reflex (contraction of quadriceps muscle) Muscles around joints tend to live in groups of opposing muscles (antagonistic muscle pairs) **Golgi tendon reflex**A diagram of the muscles of the human body Description automatically generated Golgi tendon organs detect an increase in **tension** on the muscle (and tendon), and this initiates the reflex action. The response involves: - Inhibition of agonist muscle by inhibition of its motor neurone via an inhibitory interneuron. - Contraction of antagonist muscle by excitation of its motor neurone via an excitatory interneuron. The aim of this reflex is to protect the muscle from tearing (detaching from tendon) under excessive tension. **Mechanism 1 -- Golgi Tendon organ (GTO)** 1. The muscle picks up a heavy weight so the muscle contracts 2. The tendon is pulled due to an increase in muscle contraction 3. This causes the collagen fibres in the tendon straighten and lightly compress the nerve ending of the GTO which activates it. 4. If it reaches the threshold it will fire an action potential up the sensory axon (increase in the activity of sensory neurone) 5. Excitatory transmitter is released via the sensory neuron which causes an increase in the activity of the inhibitory interneuron. 6. This causes the interneuron to releases more inhibitory neurotransmitter 7. This prevents the activation of a motor neurone 8. Therefore, it cannot release ACh 9. This decreases muscle activity which causes it to contracts less/not at all 10. This is known as a reverse myotatic reflex 🡪 more muscle activity initially results in less muscle activity after reflex. Its function involves: - To prevent damage due to overwork of muscles e.g. sudden drop in weightlifting, as Golgi tendon organ is activated resulting in skeletal muscle in arm to stop contraction. - Fine control of muscle tension - tiny adjustments to muscle activity e.g. when writing. Muscle spindles Golgi tendon organs --------------------------------------------- ---------------------------------------------- Detect stretch (change in length) of muscle Detect tension (force) on tendon and muscle Excite stretched (agonist) muscle Inhibit affected (agonist) muscle Inhibit antagonist muscle Excite antagonist muscle **Withdrawal and crossed-extensor reflexes** A **withdrawal reflex** is a reflex which involves the detection of a painful stimulus by receptors such as nociceptors or thermoreceptors, and subsequent withdrawal of the limb for protection. 1. Painful/damaging stimulus is detected by the receptor. 2. Sensory neurone delivers information to the spinal cord and synapses with excitatory interneurone. 3. Excitatory interneurone activates motor neurones by generating an action potential. 4. The motor neurones stimulate contraction of flexor muscles to withdraw limb. A **crossed-extensor reflex** aims to allow the body to compensate for the withdrawal of the limb. For example, in the case of a lower limb withdrawal reflex: 1. The afferent neurone for the damaging stimulus extends to the other side of the spinal cord. 2. Here, it synapses with excitatory interneurones which activate motor neurones for contraction of the contralateral extensor (quadricep). 3. It also synapses with inhibitory interneurones which inhibit motor neurones of the contralateral flexor (hamstring). This allows the contralateral lower limb to bear the weight of the body due to the withdrawal of the other limb.  **[Mechanism: E.g. Someone walking steps on something sharp]** 1. The sharp object will activate nociceptors once it penetrates the skin. Nociceptors are associated with sensory neurones. 2. AP travels along sensory neurone to the dorsal part of spinal cord 3. In the spinal cord the sensory neurone branches multiple times 4. One of the branches interacts with a neurone which carries information to the brain -- allows for the pain experience. 5. Other branches: NB: (black = inhibitor interneurons and red = excitatory) 6. Excitatory transmitter is released via the sensory neuron. This causes an increase in the activity of the excitatory interneuron. This causes the interneuron to release excitatory neurotransmitter. This cause an increase in the activity of the inhibitory interneuron that it is connected to. This causes the inhibitory interneuron to releases more inhibitory neurotransmitter. This prevents the activation of a motor neurone. Therefore, it cannot release ACh. This decreases **right quadricep** muscle activity so it contracts less/not at all. 7. Excitatory transmitter released via the sensory neuron also causes an increase in the activity of the excitatory interneuron. This causes the interneuron to releases more excitatory neurotransmitter. This causes an increase in activity of the second excitatory interneuron. This causes the interneuron to releases more excitatory neurotransmitter. This activates the motor neurone. Excitatory neurotransmitter (ACh) is released by the α-motor neurone at the NMJ which will cause the **right hamstring** to contract. 8. This allows for simultaneous excitation of the flexor muscle and inhibition of extensor muscle. This allows flexion at the knee allowing you to move foot away from source of the pain. 9. If this occurred whilst a person is walking, the lifting of the leg would cause them to fall over due to forward momentum. So, we need to change muscle activity of the left leg -- it needs to be firmly planted on the ground to allow for stabilisation. So, we need to activate motor neurones on the left side of the spinal cord to influence muscles on the left side. 10. Excitatory transmitter released via the sensory neuron causes an increase in the activity of an excitatory interneuron. This causes the interneuron to releases more excitatory neurotransmitter. This result in the activation of another two excitatory interneurons. The final excitatory interneuron releases excitatory neurotransmitter. This then activates the motor neurone. Excitatory neurotransmitter (ACh) is released by the α-motor neurone at the NMJ causing the **left quadricep** to contract. 11. Excitatory transmitter released via the sensory neuron will cause an increase in activity of an excitatory interneuron. This causes it to release excitatory neurotransmitter. This activates an inhibitory interneuron. This causes an increase in the activity of the inhibitor interneuron. This causes the interneuron to releases more inhibitory neurotransmitter. This prevents the activation of a motor neurone. Therefore, it cannot release ACh. This decrease left hamstring muscle activity so it relaxes. 12. This allows for extension of the knee at the left side to provide a stable base. **ALL OF THESE REFLEXES ARE SOMATIC**