MED1019 Lecture 4 — Senses PDF
Document Details
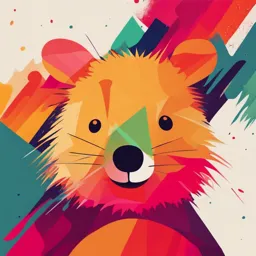
Uploaded by RomanticLaboradite465
Tags
Summary
This document covers the different types of senses and how they work in the human body.
Full Transcript
Because learning changes everything. ® Chapter 10 The Senses HOLE’S ESSENTIALS OF HUMAN ANATOMY & PHYSIOLOGY Fourteenth Edition Charles J. Welsh © 2021 McGraw Hill. All rights reserved. Authorized only for instructor use in the classroom. No reproductio...
Because learning changes everything. ® Chapter 10 The Senses HOLE’S ESSENTIALS OF HUMAN ANATOMY & PHYSIOLOGY Fourteenth Edition Charles J. Welsh © 2021 McGraw Hill. All rights reserved. Authorized only for instructor use in the classroom. No reproduction or further distribution permitted without the prior written consent of McGraw Hill. Outlines Pain Olfaction: Sense of smell Gustation: Sensation of taste Vision: Sensation of sight Sensation of Hearing and Equilibrium The Ageing effect of Nervous system © McGraw Hill 2 Introduction to the Senses Senses are derived from sensory receptors that detect changes in the environment, and stimulate neurons to send nerve impulses to the CNS for processing The body responds with feelings or sensations Senses fall into 2 categories: General senses: Widely distributed and structurally simple Touch, pressure, temperature, pain Special senses: Have complex specialized sensory organs in the head Vision, hearing, smell, taste, balance © McGraw Hill 3 Receptors, Sensations, and Perception All action potentials are the same, yet we are aware of different sensory events because of different receptors Types of sensory receptors, according to their sensitivities: Chemoreceptors: receptors sensitive to changes in chemical concentration Pain receptors: detect tissue damage Thermoreceptors: respond to temperature differences Mechanoreceptors: respond to changes in pressure or movement Photoreceptors: respond to light; found in the eye © McGraw Hill 4 Sensation and Perception 感覺 A sensation occurs when receptors are stimulated and send impulses to the brain 知覺 A perception is conscious awareness of stimuli At the same time the sensation is being formed, the brain uses projection to send the sensation back to its point of origin, so the person can pinpoint the area of stimulation The particular sensation depends on the region of the brain that receives the impulses © McGraw Hill 5 Sensory Adaptation The brain must prioritize all the incoming sensory impulses, to prevent being overwhelmed by the amount of incoming information The brain can become less responsive to a maintained stimulus such as the feel of clothing on the skin, persistent odors, ongoing sounds, etc. Sensory adaptation: ability of nervous system to become less responsive to a maintained stimulus Results from either receptors becoming unresponsive or inhibition along CNS pathway © McGraw Hill 6 General Senses General Senses: Widespread, with receptors associated with the skin, muscles, joints, and viscera Senses of touch, pressure, temperature, and pain Receptors for touch and pressure senses sense deformation or displacement of tissues: Free nerve endings of sensory nerve fibers in the epithelial tissues are associated with itching and other sensations Tactile (Meissner's) corpuscles are flattened connective tissue sheaths surrounding two or more nerve fibers; respond to motion of objects that contact the skin; abundant in hairless areas, such as lips, fingertips, palms, soles, nipples, etc. Lamellated (Pacinian) corpuscles are large structures of connective tissue fibers and cells; they function to detect deep pressure; common in deep dermis and subcutaneous layer © McGraw Hill 7 Touch and Pressure Receptors © McGraw Hill photos: (b): Ed Reschke; (c): Ed Reschke/Getty Images 8 Temperature Senses Temperature receptors include 2 groups of free nerve endings in skin: Warm receptors respond to temperatures between 25°C (77° F) and 45°C (113°F); above this range, pain receptors are stimulated, and a burning sensation is produced Cold receptors respond to temperatures between 10°C (50°F) and 20°C (68°F); below this range, pain receptors are stimulated, and a freezing sensation is produced Both warm and cold receptors adapt quickly; upon continuous stimulation for 1 minute, sensations start to fade © McGraw Hill 9 Body Position, Movement, & Stretch Receptors Proprioception: sense of body position, location in space Proprioceptors are associated with skeletal muscle; they prevent possible injury to muscles and tendons: Muscle Spindles: Bundles of special skeletal muscle fibers within skeletal muscle, with fibers of sensory neurons wrapped around muscle fibers Monitor state of muscle contraction Golgi tendon Organs: Found in tendon, near point of attachment with muscle Detect amount a tendon stretches during muscle contraction © McGraw Hill 10 Proprioceptors Access the text alternative for slide images. © McGraw Hill 11 Sense of Pain Pain receptors (nociceptors): Some consist of free nerve endings that are stimulated when tissues are damaged Overstimulation of other types of receptors, such as cold receptors, sends signals interpreted as pain Nociceptors communicate with pain sensory neurons via the neurotransmitters Substance P (spinal cord) and Glutamate (brain) Tissue damage causes prostaglandin release, increasing nociceptor sensitivity and pain intensity Aspirin and ibuprofen inhibit prostaglandin synthesis Morphine, heroin, and natural painkillers (endorphins and enkephalins) inhibit release of Substance P © McGraw Hill 12 Visceral Pain Visceral pain receptors are the only receptors in the viscera that produce sensations Visceral pain receptors respond differently than those of surface tissues; in some cases, damage does not produce pain sensations, but stretch or spasms produce strong pain sensations Pain seems to come from mechanoreceptors, decreased blood flow (oxygen deprivation), or chemicals stimulating chemoreceptors Referred pain, visceral pain that feels as if it is coming from another body area, occurs because of the common nerve pathways leading from skin and internal organs Example of referred pain: pain from the heart is often felt as if it is coming from the left shoulder or left arm © McGraw Hill 13 Referred Pain 1 GERD pain is felt as heartburn © McGraw Hill 14 Referred Pain 2 Access the text alternative for slide images. © McGraw Hill 15 Pain Nerve Fibers There are 2 types of fibers (axons) conducting pain impulses away from pain receptors: Fast (acute) pain fibers are myelinated fibers that carry impulses rapidly, are associated with sharp pain, and cease when the stimulus stops; usually sensed as coming from the skin Slow (chronic) pain fibers are unmyelinated fibers that conduct impulses slowly, produce a dull, achy sensation that is difficult to localize, and continue sending impulses after the stimulus stops; usually sensed as coming from deep tissues Often pain stimuli trigger both fast and slow pain fibers, so they produce a sharp pain followed by a slow, aching pain © McGraw Hill 16 Pain Pathways Pain impulses from the head reach brain via sensory fibers of cranial nerves; all others travel on spinal nerves Pain impulses entering the spinal cord are processed in the gray matter of the posterior horn, and then sent to the brain Most of the pain fibers terminate in the reticular formation, thalamus, or limbic system From these locations, other neurons conduct information to the hypothalamus and cerebral cortex The limbic system provides the emotional response to pain The cerebral cortex finds pain source, determines intensity of pain, and formulates motor responses to the pain © McGraw Hill 17 Special Senses The special senses contain sensory receptors in large, complex sensory organs in the head Special senses include the senses of: Smell (olfactory organs) Taste (taste buds) Hearing (ears) Equilibrium (ears) Sight (eyes) © McGraw Hill 18 Sense of Smell Olfactory Organs: Masses of epithelium in roof of the nasal cavity Contain olfactory receptors Olfactory Receptors: Receptor cells are bipolar neurons containing cilia Neurons are supported by columnar epithelial cells Each neuron contains only 1 type of an olfactory receptor membrane protein Smell (olfactory) receptors are chemoreceptors; inhaled odorants stimulate particular groups of olfactory receptors, to provide discrimination of smells Chemicals must dissolve in liquids to stimulate the receptors © McGraw Hill 19 Olfactory Nerve (Cranial Nerve 1) Smell and taste are chemical senses. The human nose contains ~ 10- 100 million receptors for smell (olfaction) in the olfactory epithelium at the superior part of the nasal cavity. Sensory signals are transducted to the primary olfactory cortex at the temporal lobe. Note that there is no relay to the thalamus. © McGraw Hill 20 The Olfactory Area and Olfactory Receptors © McGraw Hill 21 Olfactory Pathways When olfactory receptors are stimulated, their fibers synapse with neurons in the olfactory bulbs lying on either side of the crista galli of the ethmoid bone The axons of olfactory receptor neurons form Cranial Nerve I, the Olfactory Nerve Sensory impulses are first analyzed in the olfactory bulbs, and then travel to their major interpreting area within the temporal and frontal lobes of the cerebrum Some impulses proceed from the olfactory tracts into the limbic system, which provides emotional responses to certain odors © McGraw Hill 22 Olfactory Stimulation Each odor stimulates a set of specific receptors in cell membranes of olfactory receptor cells This causes an influx of sodium ions and depolarization; if threshold is reached, an action potential is generated The brain interprets different receptor combinations as an olfactory code Olfactory receptors adapt quickly, so smells fade quickly © McGraw Hill 23 Gustation: Sense of Taste Taste is also a chemical sense. There are only 5 primary tastes: sour, sweet, bitter, salt and umami (MonoSodium Glutamate; or the meaty and savory taste). Flavour other than umami is a combination of the other 4 primary tastes. Approximately 5000 (adult ) – 10,000 (children) taste bunds ae found on the mucosa of the tongue and on the soft dpalate, pharynx and epiglottis. Spicy food stimulates thermoreceptors and pain receptors at the tongue rather than the taste buds. © McGraw Hill 24 Sense of Taste Taste buds: The spherical organs of taste; each contains 50-100 taste cells Located mainly along the papillae of the tongue Some are scattered throughout the mouth and pharynx Taste Receptors: Taste cells (gustatory cells) are modified epithelial cells that function as chemoreceptors; replaced every 10 days Taste cells contain the taste hairs, which protrude from openings called taste pores; they are the sensitive portions of the cells Chemicals must be dissolved in water (saliva) in order to be tasted The sense of taste involves specific membrane protein receptors that bind with specific chemicals in food Taste cells are rapidly-adapting © McGraw Hill 25 Taste Receptors Taste buds are located in the papillae (small elevations) on the dorsum of tongue. Vallate papillae (about 12( each contains ~100 -300 taste buds) Fungiform papillae (scattered over the tongue with about 5 taste buds each) Foliate papillae (located in lateral trenches of the tongue. Most of the taste buds degenerate in early childhood). © McGraw Hill 26 Taste Sensations There are at least 5 types of taste cells Each type of taste cell is most sensitive to a certain type of chemical stimulus, resulting in 5 primary taste sensations: sweet, sour, salty, bitter, and umami (delicious) Others that may be recognized are alkaline and metallic Taste buds are responsive to one taste sensation with distinct receptors All types of taste buds are found on all parts of the tongue © McGraw Hill 27 Taste Buds © McGraw Hill 2828 Taste Receptors © McGraw Hill 29 Taste Pathways Taste impulses travel on the facial, glossopharyngeal, and vagus nerves to the medulla oblongata Impulses continue through the thalamus Impulses are interpreted in the gustatory cortex in the parietal lobe of the cerebrum © McGraw Hill 30 Sense of Hearing The ear provides the senses of hearing and equilibrium The ear has outer, middle, and inner portions Outer (External) Ear: The external ear consists of 3 portions: The auricle (pinna), which collects the sound The external acoustic meatus (external auditory canal), an S-shaped tube that transports sound toward the eardrum The tympanic membrane (eardrum), that lies at the end of the external acoustic meatus, and vibrates with the sound waves © McGraw Hill 31 Major Parts of the Ear © McGraw Hill 32 Middle Ear The middle ear (tympanic cavity) is an air-filled space in the temporal bone It houses 3 tiny bones called the auditory ossicles: malleus, incus, and stapes: The tympanic membrane vibrates the malleus, which vibrates the incus, then the stapes The stapes vibrates the fluid inside the oval window of the inner ear The vibrations in the fluid stimulate the hearing receptors in the inner ear Auditory ossicles both transmit and amplify sound waves © McGraw Hill 33 The Auditory Ossicles © McGraw Hill J and J Photography 34 Auditory Tube The auditory (eustachian) tube connects the middle ear to the nasopharynx It helps to maintain equal air pressure on both sides of the eardrum Mucous membrane infections of the throat can travel up the auditory tube to the middle ear and cause middle ear infections © McGraw Hill 35 Inner (Internal) Ear 1 Inner ear is a labyrinth: a communicating network of chambers and tubes The inner ear consists of a membranous labyrinth inside an bony (osseous) labyrinth within the temporal bone Between the two labyrinths is a fluid called perilymph Endolymph is the fluid inside the membranous labyrinth The labyrinths contain the cochlea, which functions in hearing, and the semicircular canals, which function in equilibrium © McGraw Hill 36 Inner (Internal) Ear 2 Three semicircular canals are responsible for dynamic equilibrium that has to do with maintenance of the body’s position in response to linear sudden movements. The three canals (ducts) lie at right angles to each other which allows for rotational acceleration or deceleration. © McGraw Hill 37 Inner (Internal) Ear 3 The cochlea contains 3 chambers that spiral around its entire length: Scala vestibuli, Cochlear duct, and Scala tympani The oval window leads to the upper compartment, the scala vestibuli, which runs to the tip of the cochlea The lower compartment, the scala tympani, runs from the tip of the cochlea to the round window The middle compartment, the cochlear duct, lies between these two compartments The cochlear duct is separated from the scala vestibuli by the vestibular membrane, and from the scala tympani by the basilar membrane © McGraw Hill 38 Cross Section of the Cochlea The semicircular canals, utricle and saccule work with the coordination functions of the cerebellum to maintain balance and equilibrium during movements of the human body. The cochlea translates vibrations into neural impulses that the brain can interpret as sound. The cochlea duct is 30- 35 mm in length and contains endolymph. © McGraw Hill 39 Inner (Internal) Ear 4 Vibrations of the basilar membrane are transmitted into the hearing receptor organ, the spiral organ (organ of Corti), which rests on it: Spiral organ runs the entire spiral length of the cochlea Hair cells, the hearing receptor cells, possess hairs that extend into the endolymph of the cochlear duct Above hair cells lies the tectorial membrane, which touches tips of the hairs Sound vibrations pass from the basilar membrane into the hair cells, which bend against the tectorial membrane; mechanical deformation leads to generation of action potentials in hair cells Vibrations in the fluid of the inner ear cause the hair cells in different parts of the spiral organ to bend, resulting in ability to hear sounds with different frequencies (pitch) Action potentials are conducted along the cochlear branch of the vestibulocochlear nerve, to the auditory cortex (in temporal lobe of cerebrum) © McGraw Hill 40 The Spiral Organ & Tectorial Membrane © McGraw Hill 41 The Hair Cells of the Spiral Organ © McGraw Hill Clouds Hill Imaging Ltd./Science Source 42 Auditory Pathways Nerve fibers carry impulses to the auditory cortices of the temporal lobes, where they are interpreted Some fibers cross over, so both sides of the brain can interpret impulses from both ears Hearing loss can be either conductive or sensorineural © McGraw Hill 43 Steps in Generation of Sensory Impulses in the Ear TABLE 10.1 Steps in the Generation of Sensory Impulses from the Ear 1. Sound waves enter external acoustic meatus. 2. Sound waves cause eardrum to reproduce vibrations coming from sound source. 3. Auditory ossicles amplify and transfer vibrations to end of stapes. 4. Movement of stapes at oval window transfer vibrations to perilymph in scala vestibuli. 5. Vibrations pass through vestibular membrane and enter endolymph of cochlear duct, where they move the basilar membrane. 6. Different frequencies of vibration of basilar membrane stimulate different sets of receptor cells. 7. As a receptor cell depolarizes, its membrane becomes more permeable to calcium ions. 8. Inward diffusion of calcium ions causes vesicles at base of the receptor cell to release neurotransmitter. 9. Neurotransmitter stimulates dendrites of nearby sensory neurons. 10. Sensory impulses are triggered on fibers of the cochlear branch of vestibulocochlear nerve. 11. Auditory cortices of temporal lobes interpret sensory impulses. © McGraw Hill 44 Sense of Equilibrium The sense of equilibrium consists of two parts: static and dynamic equilibrium: The organs of static equilibrium help to maintain the position of the head, posture, and balance when the head and body are still The organs of dynamic equilibrium help to maintain balance when the head and body suddenly move or rotate © McGraw Hill 45 Static Equilibrium Organs of static equilibrium are located within the vestibule of the inner ear, between the cochlea and semicircular canals Membranous labyrinth in the vestibule contains 2 chambers: utricle and saccule Each chamber contains an organ of static equilibrium, called a macula, which consists of: Hair cells, which are the sensory receptors Gelatinous material, which the hairs of hair cells project into Otoliths, grains of calcium carbonate, which are embedded in the gelatinous mass Gravity or head movement causes the gelatin and otoliths to shift, bending hairs of hair cells and generating action potentials Impulses travel to the brain via the vestibular branch of the vestibulocochlear nerve, indicating the position of the head © McGraw Hill 46 Maculae and the Sense of Static Equilibrium © McGraw Hill 47 Dynamic Equilibrium 1 Organs of dynamic equilibrium are inside the semicircular canals of the inner ear The 3 semicircular canals detect motion of the head, and they aid in balancing the head and body during sudden movement The organs of dynamic equilibrium are called cristae ampullaris, and are located in the ampulla of each semicircular canal of the inner ear The canals and cristae are situated at right angles to each other Hair cells, the sensory receptor cells, extend into a dome-shaped gelatinous mass, called a cupula © McGraw Hill 48 Dynamic Equilibrium 2 Rapid turning or movement of the head or body causes the semicircular canals to move; the endolymph remains stationary This leads to bending of the cupula and the hairs of the hair cells embedded within it Bending of the hairs generates action potentials, and impulses are conducted to the brain Impulses travel to the brain via the vestibular branch of the vestibulocochlear nerve, indicating the position of the head Mechanoreceptors (called proprioceptors) associated with the joints, and the changes detected by the eyes, also help maintain equilibrium © McGraw Hill 49 A Crista Ampullaris © McGraw Hill 50 Head Rotation & Dynamic Equilibrium © McGraw Hill 51 Anatomy of the Eye © McGraw Hill 5252 Sense of Sight The eye is the organ that contains visual receptors, which provide sense of vision. Accessory organs of sight: the lacrimal apparatus, eyelids, and extrinsic muscles; provide assistance through protection and movement: Eyelid: Protects the eye from foreign objects 4 layers: skin (thinnest in body), muscle, connective tissue, conjunctiva Muscles: orbicularis oris & levator palpebrae superioris Conjunctiva: a mucous membrane that lines the inner surface of the eyelids and folds back to cover the anterior surface of the eyeball (except over the cornea) © McGraw Hill 53 Closed Eyelids & Anterior Eye (Sagittal Section) The eyeball is composed of a fibrous layer (Corner and Scler), a vascular layer (Choroid, Ciliary body and Iris) and an inner layer with the Retina (neural part, non-neural part). © McGraw Hill 54 Visual Accessory Organs Lacrimal Apparatus: Lacrimal gland produces tears that lubricate & cleanse the eye Tiny tubules release tears over the surface of the eye 2 small ducts, the canaliculi, drain tears into the lacrimal sac; the tears proceed into the nasolacrimal duct and then into the nasal cavity Tears also contain an antibacterial enzyme called lysozyme Extrinsic eye muscles: Attach to the sclera 6 extrinsic eye muscles move the eye in all directions © McGraw Hill 55 The Lacrimal Apparatus © McGraw Hill 56 The Extrinsic Eye Muscles © McGraw Hill 57 Muscles of the Eyelids and Eyes TABLE 10.2 Muscles Associated with the Eyelids and Eyes Name Innervation Function Muscles of the Eyelids Orbicularis oculi Facial nerve (VII) Closes eye Levator palpebrae Oculomotor nerve (III) Opens eye superioris Extrinsic Muscles of the Eyes Superior rectus Oculomotor nerve (III) Rotates eye upward and toward midline Inferior rectus Oculomotor nerve (III) Rotates eye downward and toward midline Medial rectus Oculomotor nerve (III) Rotates eye toward midline Lateral rectus Abducens nerve (VI) Rotates eye away from midline Rotates eye downward and away from Superior oblique Trochlear nerve (IV) midline Inferior oblique Oculomotor nerve (III) Rotates eye upward and away from midline © McGraw Hill 58 Structure of the Eye The eye is a fluid-filled hollow sphere The eye has 3 distinct layers: Outer (fibrous) layer Middle (vascular) layer Inner (nervous) layer Spaces of the eye contain fluids to maintain shape © McGraw Hill 59 Transverse Section of the Eye © McGraw Hill 60 Outer Layer Outer (fibrous) layer: Consists of the cornea and the sclera The cornea is transparent, and makes up the anterior 1/6 of the outer layer; helps focus light rays The sclera is white, and makes up the posterior 5/6 of the outer layer The sclera is made of mostly collagen; it protects the eye and is an attachment for the extrinsic eye muscles The optic nerve and blood vessels pierce the sclera in the posterior of the eye © McGraw Hill 61 Middle Layer 1 Middle (vascular) layer: Consists of the choroid coat, ciliary body, and iris The choroid coat is vascular and darkly pigmented; it performs 2 functions: to nourish other tissues of the eye and to keep the inside of the eye dark The ciliary body forms a ring around the front of the eye; it contains ciliary processes, ciliary muscles and suspensory ligaments, that hold the lens in position and change its shape to focus images at different distances © McGraw Hill 62 The Lens and Ciliary Body, Posterior View © McGraw Hill 63 Middle Layer 2 The ability of the lens to adjust shape to facilitate focusing on objects at different distances is called accommodation When the ciliary muscle relaxes, the suspensory ligaments pull outward, causing the lens to flatten to focus on distant objects When the ciliary muscle contracts, the suspensory ligaments relax, allowing the lens to take on a more convex shape to view closer objects © McGraw Hill 64 Accommodation © McGraw Hill 65 Middle Layer 3 The iris: A thin, pigmented diaphragm of smooth muscle The colorful portion of the eye Lies between cornea and lens Adjusts the amount of light entering the pupil, an opening in center of iris: Dim light stimulates the radial muscles of the iris, and the pupil dilates Bright light stimulates the circular muscles, and the pupil constricts Aqueous humor is secreted by the ciliary body; it fills the space between the cornea and lens (the anterior cavity); nourishes cells and maintains shape © McGraw Hill 66 Dilation and Constriction of the Pupil © McGraw Hill 67 Inner Layer 1 The inner layer: Consists of the retina, which contains photoreceptors Almost transparent, and consists of several layers of cells Continuous with optic nerve in the back of the eye Forms inner lining of eye, extending forward almost to the ciliary body In the center of the retina is the macula lutea, which has a central depression called the fovea centralis; this is the point of sharpest vision in the retina © McGraw Hill 68 The Layers of Cells in the Retina Access the text alternative for slide images. © McGraw Hill 69 Retinal Structure © McGraw Hill Ed Reschke/Getty Images 70 Inner Layer 2 Medial to the fovea centralis is the optic disc, where nerve fibers leave the eye as the optic nerve; also called the blind spot, due to lack of photoreceptors in this area The posterior cavity of the eye is the space bounded by lens, ciliary body and retina; largest compartment of the eye Posterior cavity is filled with vitreous humor, which, together with collagenous fibers, forms the vitreous body Vitreous body supports the internal parts of the eye and helps the eye maintain its shape © McGraw Hill 71 Features of the Retina © McGraw Hill (b): Thalerngsak Mongkolsin/Shutterstock 72 Light Refraction & Corrective Lenses Focusing causes light rays to bend, which is called refraction Refraction causes images to fall on fovea centralis of retina Both the cornea and lens refract light rays that are focused on the retina, and so do the humors to a lesser degree Emmetropia: normal vision, due to normal eye shape; light waves focus sharply on the retina Myopia (nearsightedness): light rays focus in front of retina, and then scatter, causing blurry image; corrected by concave lens Hyperopia (farsightedness): light rays focus in back of retina, but are not yet converged when they reach retina; corrected by convex lens © McGraw Hill 73 Visual Disorders and Corrections Access the text alternative for slide images. © McGraw Hill 74 Photoreceptors Photoreceptors: Modified neurons, which are the visual receptor cells 2 types: elongated rods and blunt-shaped cones There is 1 type of rod, and there are 3 types of cones Rods: More sensitive to light than cones, and function in dim light Provide black and white vision Provide less precise images (general outlines) than cones, because their axon branches converge onto fewer nerve fibers There are many more rods than cones in the retina Cones: Provide sharp images in bright light Provide color vision Fovea centralis contains densely packed cones, but no rods © McGraw Hill 75 Conduction of Nerve Impulses from Rods & Cones to the Brain Electron Micrograph of Rods & Cones © McGraw Hill 76 Photopigments 1 Rods and cones have light-sensitive pigments that break down upon absorption of light energy Rhodopsin (visual purple): the light-sensitive pigment in rods When stimulated by light, rhodopsin decomposes into a protein, opsin, and retinal (produced from vitamin A) Decomposition of rhodopsin activates an enzyme that initiates changes in the rod cell membrane, generating a nerve impulse Nerve impulses leave the retina via the optic nerve, and travel to the visual cortex, where they are interpreted as vision In bright light, almost all of the rhodopsin is broken down In dim light, rhodopsin regeneration is faster than breakdown © McGraw Hill 77 Photopigments 2 The light-sensitive pigments in cones also consist of opsin proteins and retinal There are three sets of cones, each containing a different opsin protein The wavelength of light determines the color perceived from it; each of the three pigments is most sensitive to different wavelengths of light: Erythrolabe: photopigment most sensitive to red light Chlorolabe: photopigment most sensitive to green light Cyanolabe: photopigment most sensitive to blue light If all 3 sets of cones are stimulated, the color is white; if none are stimulated, the color is black Different forms of colorblindness result from lack of different cone pigments © McGraw Hill 78 Visual Pathways 1 The axons of retinal neurons leave the eyes to form the optic nerves Fibers from the medial half of each retina cross over in a point of convergence of the optic nerves, called the optic chiasma Pathway continues as fibers from the optic chiasma form optic tracts, which proceed to the thalamus From the thalamus, nerve pathways called optic radiations end in the visual cortex of the occipital lobes of the cerebrum © McGraw Hill 79 The neural pathway for vision begins when the rods Visual Pathways 2 and cones convert light energy into neural signals that are directed to the optic (II) nerves. The pathway: 1. Optic (II) nerves 2. Optic chiasm 3. Optic tract 4. Lateral geniculate nucleus of Thalamus 5. Optic radiations that send information to the primary visual areas. 6. The Primary visual areas of the occipital lobes receive optic radiations and produce perception. © McGraw Hill 80