MLS 111L Human Anatomy and Physiology With Pathophysiology PDF
Document Details
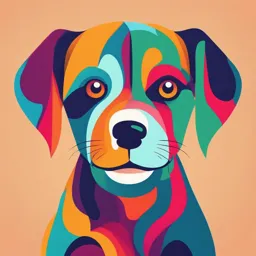
Uploaded by CourteousDwarf1185
Jarell Mae O. Alfonso
Tags
Summary
These notes cover the special senses, including smell, taste, vision, hearing, and balance. It examines anatomy and physiology of these body systems. The text also looks at how the eye works, focusing on the different structures involved in forming images on the retina.
Full Transcript
Sense is the ability to perceive stimuli Sensation is the process initiated by stimulating sensory receptors and perception is the conscious awareness of those stimuli Brain constantly receives a wide variety of stimuli from both inside and outside the body, but stimulation of sensor...
Sense is the ability to perceive stimuli Sensation is the process initiated by stimulating sensory receptors and perception is the conscious awareness of those stimuli Brain constantly receives a wide variety of stimuli from both inside and outside the body, but stimulation of sensory receptors does not immediately result in perception Perception results when action potentials reach the cerebral cortex Senses are divided into 2 basic groups: General and Special senses A. GENERAL SENSES have receptors distributed over a large part of the body. Divided into 2 groups: the somatic senses and the visceral senses a. SOMATIC SENSEs provide sensory information about the body and the environment b. VISCERAL SENSES provide information about various internal organs, primarily involving pain and pressure B. SPECIAL SENSES are more specialized in structure and are localized to specific parts of the body. The special senses are smell, taste, sight, hearing, and balance. And these special types of senses are what we will tackle for our Adult eyeball measures about 2.5 cm (1 in.) in diameter Of its total surface area, only the anterior one-sixth is exposed; the remainder is recessed and protected by the orbit, into which it fits Anatomically, the wall of the eyeball consists of three layers: (I) fibrous tunic, (II) vascular tunic, and (III) retina I. FIBROUS TUNIC The fibrous tunic is the superficial layer of the eyeball and consists of the anterior cornea and posterior sclera. A. CORNEA - Transparent coat that covers the colored iris Because it is curved, the cornea helps focus light onto the retina Its outer surface consists of nonkeratinized stratified squamous epithelium The middle coat of the cornea consists of collagen fibers and fibroblasts, and the inner surface is simple squamous epithelium. I. FIBROUS TUNIC B. SCLERA - “White” of the eye A layer of dense connective tissue made up mostly of collagen fibers and fibroblasts Covers the entire eyeball except the cornea; it gives shape to the eyeball, makes it more rigid, protects its inner parts, and serves as a site of attachment for the extrinsic eye muscles At the junction of the sclera and cornea is an opening known as the scleral venous sinus(canal of Schlemm). A fluid called aqueous humor, drains into this sinus. II. VASCULAR TUNIC The vascular tunic or uvea is the middle layer of the eyeball. It is composed of 3 parts: choroid, ciliary body, and iris. A. CHOROID Highly vascularized located at the posterior portion of the vascular tunic - blood vessels provide nutrients to the posterior surface of the retina Contains melanocytes which causes this layer to appear dark brown in color Melanin in the choroid absorbs stray light rays which prevents reflection and scattering of light within the eyeball II. VASCULAR TUNIC B. CILIARY BODY - Anterior portion of the vascular tunic Dark brown in color because it contains melanocytes Consists of ciliary muscles (circular band of smooth muscle) and ciliary processes (protrusions or folds on the internal surface) Contain blood capillaries that secrete aqueous humor Extending from the ciliary process are zonular fibers (suspensory ligaments) that attach to the lens Contraction or relaxation of the ciliary muscle changes the tightness of the zonular fibers, which alters the shape of the lens, adapting it for near or far vision. II. VASCULAR TUNIC C. IRIS Colored portion of the eyeball and shaped like a flattened donut It is suspended between the cornea and the lens Consists of melanocytes, and circular and radial smooth muscle fibers. The amount of melanin in the iris determines the eye color A principal function of the iris is to regulate the amount of light entering the eyeball through the pupil II. VASCULAR TUNIC D. PUPIL - Hole in the center of the iris Appears black because, as you look through the lens, you see the heavily pigmented back of the eye (choroid and retina) If bright light is directed into the pupil, the reflected light is red because of the blood vessels on the surface of the retina Autonomic reflexes regulate pupil diameter in response to light levels II. VASCULAR TUNIC D. PUPIL - Hole in the center of the iris When bright light stimulates the eye, parasympathetic fibers of the oculomotor (III) nerve stimulate the circular muscles or sphincter papilla of the iris to contract, causing a decrease in the size of the pupil (constriction) In dim light, sympathetic neurons stimulate the radial muscles or dilator pupillae of the iris to contract, causing an increase in the pupil’s size (dilation) III. RETINA Third and inner layer that lines the posterior three-quarters of the eyeball and the beginning of the visual pathway. A. OPTIC DISC Site where the optic (II) nerve exits the eyeball Bundled together with the optic nerve are the central retinal artery, a branch of the ophthalmic artery, and the central retinal vein Branches of the central retinal artery fan out to nourish the anterior surface of the retina; the central retinal vein drains blood from the retina through the optic disc III. RETINA B. PIGMENTED LAYER A sheet of melanin-containing epithelial cells located between the choroid and the neural part of the retina The melanin in the pigmented layer of the retina, as in the choroid, helps to absorb stray light rays C. NEURAL (SENSORY) LAYER A multilayered outgrowth of the brain that processes visual data extensively before sending nerve impulses into axons that form the optic nerve LENS Located behind the pupil and iris Within the cells of the lens, proteins called crystallins, arranged like the layers of an onion, make up the refractive media of the lens, which normally is perfectly transparent and lacks blood vessels Enclosed by a clear connective tissue capsule and held in position by encircling zonular fibers, which attach to the ciliary processes Helps focus images on the retina to facilitate clear vision INTERIOR OF THE EYEBALL The lens divides the interior of the eyeball into two cavities: the Anterior cavity and Vitreous chamber. I. ANTERIOR CAVITY The space anterior to the lens—consists of two chambers Anterior Chamber lies between the cornea and the iris Posterior chamber lies behind the iris and in front of the zonular fibers and lens INTERIOR OF THE EYEBALL I. ANTERIOR CAVITY Both chambers are filled with aqueous humor, a transparent watery fluid that nourishes the lens and cornea Aqueous humor continually filters out of blood capillaries in the ciliary processes of the ciliary body and enters the posterior chamber It then flows forward between the iris and the lens, through the pupil, and into the anterior chamber Normally, aqueous humor is completely replaced about every 90 minutes INTERIOR OF THE EYEBALL II. VITREOUS CHAMBER Larger posterior cavity of the eyeball which lies between the lens and the retina Within the vitreous chamber is the vitreous body, a transparent jellylike substance that holds the retina flush against the choroid, giving the retina an even surface for the reception of clear images INTERIOR OF THE EYEBALL II. VITREOUS CHAMBER Unlike the aqueous humor, the vitreous body does not undergo constant replacement It is formed during embryonic life and consists of mostly water plus collagen fibers and hyaluronic acid Also contains phagocytic cells that remove debris, keeping this part of the eye clear for unobstructed vision INTERIOR OF THE EYEBALL II. VITREOUS CHAMBER The pressure in the eye, called intraocular pressure, is produced mainly by the aqueous humor and partly by the vitreous body Normally it is about 16 mmHg (millimeters of mercury) Intraocular pressure maintains the shape of the eyeball and prevents it from collapsing In some ways the eye is like a camera: Its optical elements focus an image of some object on a light-sensitive “film”— the retina—while ensuring the correct amount of light to make the proper “exposure.” To understand how the eye forms clear images of objects on the retina, we must examine 3 processes: (1) Refraction: Bending of light by the lens and cornea (2) Accommodation: Change in shape of the lens (3) Constriction: Narrowing of the pupil I. REFRACTION OF LIGHT RAYS When light rays traveling through a transparent substance (such as air) pass into a second transparent substance with a different density (such as water), they bend at the junction between the two substances --- REFRACTION As light rays enter the eye, they are refracted at the anterior and posterior surfaces of the cornea Both surfaces of the lens of the eye further refract the light rays so they come into exact focus on the retina. Images focused on the retina are inverted (upside down). I. REFRACTION OF LIGHT RAYS They also undergo right-to-left reversal; that is, light from the right side of an object strikes the left side of the retina, and vice versa. The reason the world does not look inverted and reversed is that the brain “learns” early in life to coordinate visual images with the orientations of objects. The brain stores the inverted and reversed images we acquired when we first reached for and touched objects and interprets those visual images as being correctly oriented in space. I. REFRACTION OF LIGHT RAYS About 75% of the total refraction of light occurs at the cornea. The lens provides the remaining 25% of focusing power and also changes the focus to view near or distant objects. When an object is 6 m (20 ft) or more away from the viewer, the light rays reflected from the object are nearly parallel to one another. The lens must bend these parallel rays just enough so that they fall exactly focused on the central fovea, where vision is sharpest. I. REFRACTION OF LIGHT RAYS Because light rays that are reflected from objects closer than 6m are divergent rather than parallel, the rays must be refracted more if they are to be focused on the retina. This additional refraction is accomplished through a process called accommodation. II. ACCOMMODATION AND THE NEAR POINT OF VISION A surface that curves outward, like the surface of a ball, is said to be convex. When the surface of a lens is convex, that lens will refract incoming light rays toward each other, so that they eventually intersect. If the surface of a lens curves inward, like the inside of a hollow ball, the lens is said to be concave and causes light rays to refract away from each other. The lens of the eye is convex on both its anterior and posterior surfaces, and its focusing power increases as its curvature becomes greater. II. ACCOMMODATION AND THE NEAR POINT OF VISION When the eye is focusing on a close object, the lens becomes more curved, causing greater refraction of the light rays. This increase in the curvature of the lens for near vision is called accommodation. The near point of vision is the minimum distance from the eye that an object can be clearly focused with maximum accommodation. This distance is about 10 cm (4 in.) in a young adult. II. ACCOMMODATION AND THE NEAR POINT OF VISION When viewing distant objects, the ciliary muscle is relaxed and the lens is flatten because it is stretched in all directions by taut zonular fibers When viewing a close object, the ciliary muscle contracts, which pulls the ciliary process and choroid forward toward the lens. This action releases tension on the lens and zonular fibers. Because it is elastic, the lens becomes more spherical (more convex), which increases its focusing power and causes greater BRAIN PATHWAY AND VISUAL FIELDS The axons within the optic (II) nerve pass through the optic chiasm (a crossover, as in the letter X), a crossing point of the optic nerves and some axons cross to the opposite side, but others remain uncrossed. After passing through the optic chiasm, the axons, now part of the optic tract, enter the brain and most of them terminate in the lateral geniculate nucleus of the BRAIN PATHWAY AND VISUAL FIELDS Here they synapse with neurons (passing of nerve impulse) whose axons form the optic radiations, which project to the primary visual areas in the occipital lobes of the cerebral cortex, and visual perception begins. Some of the fibers in the optic tracts terminate in the superior colliculi, which control the extrinsic eye muscles, and the pretectal nuclei, which control pupillary and accommodation reflexes. Everything that can be seen by one eye is that eye’s visual field. As noted earlier, because our eyes are located anteriorly in our heads, the visual fields overlap considerably. BRAIN PATHWAY AND VISUAL FIELDS We have binocular vision due to the large region where the visual fields of the two eyes overlap— binocular visual field. The visual field of each eye is divided into 2 regions: the nasal or central half and the temporal or peripheral half. For each eye, light rays from an object in the nasal half of the visual field fall on the temporal half of the retina, and light rays from an object in the temporal half of the visual BRAIN PATHWAY AND VISUAL FIELDS Visual information from the right half of each visual field is conveyed to the left side of the brain, and visual information from the left half of each visual field is conveyed to the right side of the brain, as follows: 1. The axons of all retinal ganglion cells in one eye exit the eyeball at the optic disc and form the optic nerve on that side. 2. At the optic chiasm, axons from the temporal half of each retina do not cross but continue directly to the lateral geniculate nucleus of the thalamus on the same side. BRAIN PATHWAY AND VISUAL FIELDS 3. In contrast, axons from the nasal half of each retina cross the optic chiasm and continue to the opposite thalamus. 4. Each optic tract consists of crossed and uncrossed axons that project from the optic chiasm to the thalamus on one side 5. Axon collaterals (branches) of the retinal ganglion cells project to the midbrain, where they participate in neural circuits that govern constriction of the pupils in response to light and coordination of head and eye movements. BRAIN PATHWAY AND VISUAL FIELDS * (#5 cont.) Collaterals also extend to the suprachiasmatic nucleus of the hypothalamus, which establishes patterns of sleep and other activities that occur on a circadian or daily schedule in response to intervals of light and darkness. 6. The axons of thalamic neurons form the optic radiations as they project from the thalamus to the primary visual area of the cortex on the same side. BRAIN PATHWAY AND VISUAL FIELDS Although we have just described the visual pathway as a single pathway, visual signals are thought to be processed by at least three separate systems in the cerebral cortex, each with its own function. (1) One system processes information related to the shape of objects, (2) another system processes information regarding color of objects, and a (3) third system processes information about movement, location, and spatial organization. Our sense of smell reacts with the molecules in the air called odorants, or scent as you call it. As odorants enter the nasal cavity (space inside your nose), they are trapped in the epithelial cells lining your nose, thus stimulating your scent receptors. Our nose is lined with 3 types of epithelial cells namely: olfactory receptor cells, supporting cells, and basal cells. 1. OLFACTORY RECEPTOR CELLS These are bipolar neurons (one axon, one dendrite) that contain the olfactory cilia, hair like projections that have the receptors that are stimulated by the odorants. 2. SUPPORTING CELLS These are columnar epithelial cells that provide physical support, nourishment, insulation to the olfactory receptor cells. They also provide protection by detoxifying chemicals that come in contact with the olfactory epithelium. 3. BASAL CELLS These are stem cells that continually divide to produce new olfactory receptor cells. OLFACTORY GLAND OR BOWMAN’S GLAND An epithelium lining the nasal cavity, although they do not participate directly in odor determination, they are important to note since they are the ones producing mucus that keeps the nasal cavity moist. Moreover, the mucus helps to dissolve the odorant to better interact with the olfactory cilia. Once an electrical impulse is generated, the signal travels from the dendrite of the olfactory receptor cell to its axon, wherein collection of these axons from different cells is termed Olfactory Nerve I. These nerves pass a bone (cribriform plate) that divides the brain and the nasal cavity, ending in an area of gray matter just below the frontal lobe of the cerebrum, termed as olfactory bulb. Neurons in the olfactory bulb have long axons that reach to the temporal (side) of the brain, the signal received will travel through this and will reach the primary olfactory area on the temporal part of the cerebral cortex where consciousness of smell begins. Note that olfaction cannot always be available to smell the same scent continuously. Our ability to perceive the same scent decreases gradually, this is called olfactory adaptation. This is why the scent we smell cannot be as strong as it was minutes after smelling it. The sense of taste or gustation, like the olfaction, responds to chemical stimuli. However, it is much simpler than olfaction for only 5 primary tastes can be distinguished: sour, sweet, bitter, salty, and umami. The sensory structures that detect taste stimuli are the taste buds. Most of the nearly 10,000 taste buds of a young adult are on the tongue, but some are found on the soft palate (posterior portion of the roof of the mouth), pharynx (throat), and epiglottis (cartilage lid over voice box). The taste bud is an oval shaped body found in elevations on the tongue called papillae (singular is papilla) consisting of 3 types of epithelial cells: 1. GUSTATORY RECEPTOR CELLS: These have microvilli (hair like projections) that picks up the chemical stimuli from anything ingested 2. SUPPORTING CELLS: These cells bring physical support to the gustatory receptor cells. They can also become new receptor cells when needed. 3. BASAL CELLS: Stem cells located the base which produce new supporting cells. Tastants will stimulate the gustatory receptors located at different parts of the oral (buccal) cavity and that which will generate nerve impulse that will go straight to gustatory nucleus in the medulla oblongata. From here, some impulse will project to the thalamus and to the primary gustatory area of the brain where conscious perception of taste arises. Note that odorants from food can rise upward from the mouth to the nasal cavity where olfaction can be stimulated. 3 cranial nerves contain axons of the first-order gustatory neurons that innervate (supply) the taste buds. The facial (VII) nerve serves taste buds in the anterior two- thirds of the tongue; the glossopharyngeal (IX) nerve serves taste buds in the posterior one-third of the tongue; and the vagus (X) nerve serves taste buds in the throat and epiglottis. From the taste buds, nerve impulses propagate along these cranial nerves to the gustatory nucleus in the medulla oblongata. From the medulla, some axons carrying taste signals project to the limbic system and the hypothalamus; others project to the thalamus. Taste signals that project from the thalamus to the primary gustatory area in the insula of the cerebral cortex give rise to the conscious perception of taste and discrimination of taste sensations. Vibrations create sound waves. Sound waves are collected by the auricle and pass through the external auditory canal toward the ear drum (tympanic membrane). Sound waves strike the tympanic membrane and cause it to vibrate. This vibration causes the vibration of the 3 ossicles of the middle ear, and by this mechanical linkage, the force of vibration is amplified and transferred to the oval window. This now moves the sensory receptor causing it to release an impulse. This impulse will now travel and pass different parts of the brain before it reaches the primary auditory area in the cerebral cortex. This area is where sound recognition happens. Balance (equilibrium) can be divided into 2 kinds: STATIC EQUILIBRIUM: Refers to the position of the body (mainly head) in relation to gravity. The movements that stimulate the receptors of this type of equilibrium is tilting of the head or speeding up or slowing down of the speed, as during riding a moving car. DYNAMIC EQUILIBRIUM: Refers to the maintenance of body position (mainly head) in response to sudden movement such as rotational movement. Collectively, the receptor organs for equilibrium are called the vestibular apparatus - these include the saccule, utricle, and semicircular ducts. The 2 chambers, utricle and the saccule, each contain a specialized epithelium called maculae. The maculae have hair cells contained in a gelatinous mass called otolithic membrane. The weighted gelatinous mass moves in response to gravity, bending the hair cells and causing nerve signals to be generated. The signal from the vestibular apparatus will travel to the vestibular area in the parietal lobe of the cerebral cortex to provide us with the conscious awareness of the position and movements of the head and limbs. OTOLITHIC ORGANS: SACCULE AND UTRICLE The walls of both the utricle and the saccule contain a small, thickened region called a macula. The two maculae, which are perpendicular to one another, are the receptors for static equilibrium. Maculae consist of 2 kinds of cells: hair cells, which are the sensory receptors, and supporting cells. OTOLITHIC ORGANS: SACCULE AND UTRICLE The (1) hair cells are the receptors for the static equilibrium. Together with these are (2) columnar supporting cells that secrete a thick gelatinous, glycoprotein layer called otolithic membrane that rests on the hair cells. A layer of dense calcium carbonate crystals, called otoliths, extends over the entire surface of the otolithic membrane. SEMICIRCULAR DUCTS The 3 semicircular ducts function in dynamic equilibrium. The ducts lie at right angles to one another in 3 planes: The two vertical ducts are the anterior and posterior semicircular ducts, and the horizontal one is the lateral semicircular duct. This positioning permits detection of rotational acceleration or deceleration. SEMICIRCULAR DUCTS In the ampulla, the dilated portion of each duct, is a small elevation called the crista. Each crista contains a group of hair cells and supporting cells. Covering the crista is a mass of gelatinous material called the cupula. When you move your head, the attached semicircular ducts and hair cells move with it. The release of neurotransmitter from hair cells of the spiral organ ultimately generates action potentials in the first- order auditory neurons that innervate the hair cells. The axons of these neurons form the cochlear branch of the vestibulocochlear (VIII) nerve. These axons synapse with neurons in the cochlear nuclei in the medulla oblongata. Some of the axons from the cochlear nuclei decussate (cross over) in the medulla, ascend in a tract called the lateral lemniscus on the opposite side, and terminate in the inferior colliculus of the midbrain. Other axons from the cochlear nuclei end in the superior olivary nucleus of the pons. Slight differences in the timing of action potentials arriving from the two ears at the superior olivary nuclei allow us to locate the source of a sound. Axons from the superior olivary nuclei ascend to the midbrain where they terminate in the inferior colliculi.