Metabolism - 1 PDF
Document Details
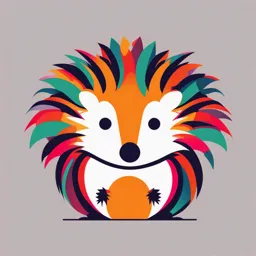
Uploaded by AmusingBambooFlute
The University of Hong Kong
Tags
Summary
This document provides an overview of carbohydrate metabolism, including the digestion of dietary carbohydrates, glucose transport, glycolysis, and aerobic respiration. It discusses the different types of glucose transporters and their roles in various tissues, along with the fates of glucose after entering the cells.
Full Transcript
4. METABOLISM 4.1. GLUCOSE METABOLISM 4.1.1. COMMON STRUCTURES OF DIETARY CARBOHYDRATES - Carbohydrates are organic molecules - The empirical formula for many of the simpler carbohydrates is (CH2O)n, where n ≥3, hence the name “hydrate of carbon” - Dietary carb...
4. METABOLISM 4.1. GLUCOSE METABOLISM 4.1.1. COMMON STRUCTURES OF DIETARY CARBOHYDRATES - Carbohydrates are organic molecules - The empirical formula for many of the simpler carbohydrates is (CH2O)n, where n ≥3, hence the name “hydrate of carbon” - Dietary carbohydrates: - Major intake of carbohydrates in humans is starch - Humans also take in some cellulose, which stimulates healthy peristalsis - Glycogen is stored in the body but not often ingested, since it degrades rapidly - Structure of glycogen is similar to amylopectin in starch - Stereoisomers of glucose: - Depends on orientation of OH group on C-1 - Leads to differences in 3D structure: - Determines whether or not they can be recognised by specific enzymes - Also have L- and D-forms 4.1.2. DIGESTION OF DIETARY CARBOHYDRATES 73 - Glucose in diets: - Starch is the major carbohydrate in our diet - Glycogen is one of the fuel molecules our body stores - Glucose is the common monomer in both - Hence, the single unit of glucose structure must be cleaved off from its polymers or dimers during digestion in the GI tract, before absorption and transportation in liver and muscle cells for assembly - Mainly makes use of 𝛼-D-glucose - Degradation of amylose and amylopectin by α-amylases: - Amylose: linear chain of 𝛼-glucose, with 1,4-glycosidic bonds - Amylopectin: branched chains of 𝛼-glucose, with 1,6-glycosidic bonds - Spatially, amylose has more efficient packaging of monomers - Others: - Lactose – 𝛽-1,4 linkage between galactose and glucose Sucrose – 𝛼-1,2 linkage between fructose and glucose [Maltotriose – 3 glucose molecules; Maltose – 𝛼-1,4 linkage between 2 glucose molecules; Glucose] [Alpha limit dextrins – a short chained branched amylopectin remnant, maintains 𝛼-1,4 and 𝛼-1,6 bonds; Isomaltose – 𝛼-1,6 linkage between 2 glucose molecules] - The different glycosidase activities are found in four glycoproteins: 1. Glucoamylase – release glucose from 𝛼-1,4 linked glycosyl residues 2. Sucrase–isomaltase complex – cleave sucrose and other 𝛼-1,4 linked glycosyl residues, and cleaving some 𝛼-1,6 bonds such as isomaltose 3. Trehalase – cleave trehalose, an 𝛼-1,1 glucose-glucose disaccharide 4. Lactase-glucosylceramidase – 𝛽-glycosidase complexes, cleave 𝛽-1-4 bonds in lactose [Glycosidases: - Enzymes, e.g. lactase, attached to the brush border, produced by the enterocytes - They are collectively called the small intestinal disaccharidases, although glucoamylase is really an oligosaccharide] [Trehalose: 74 - Trehalose, which is found in insects, algae, mushrooms, and other fungi, is not currently known to be a major dietary component - Trehalase deficiency was discovered when a woman became very sick after eating mushrooms and was initially thought to have α-amanitin poisoning - Amanitin – RNA polymerase inhibitor present in some mushrooms - Trehalase deficiency is rare except among Greenland Eskimos] - Pathway of digestion: - The salivary alpha amylase can quickly cleave some 𝛼-1,4 bonds on the amylose chain of starch - Amylase is inactivated in acidic environment of his stomach - Rapid ingestion indicates a reliance on efficient enzymatic digestion in small intestine (no time for salivary amylase to act on starch) - Digestion by pancreatic 𝛼-amylase in lumen - Other hydrolase enzymes on mucosal cell – which cleave the dietary carbohydrates into free monosaccharides for uptake across mucosal cells into the portal circulation (to the liver) - Undigested carbohydrates go straight to ileum and may be fermented by bacteria and eventually secreted - In the duodenal lumen, further 𝛼-1,4 bonds in amylose are cleaved by pancreatic 𝛼-amylase to release free glucose - Branched forms such as 𝛼-dextrin, maltotriose and maltoses, and lactose/ sucrose are hydrolysed to form monosaccharides - Only monosaccharides are absorbed by the gut - Structure of intestinal villi: - Blood and lymph branches are abundantly connected to basal side of villi - Enterocytes and globular cells near the surface of villi - Highly folded to maximise surface area - Digestive enzymes, such as disaccharidase complexes and glycosidases are attached to the membrane of the brush border of absorptive cells (enterocytes) - Brush border faces the lumen of intestine, contains digestive enzymes - The dietary disaccharides, lactose and sucrose, are converted to monosaccharides by glycosidases (small intestinal disaccharidases) 75 - Free monosaccharides are soluble, polar, and repelled by phospholipid bilayer’s internal non- polar layer - Once the carbohydrates have been split into monosaccharides, the sugars are transported across the intestinal epithelial cells and into the blood for distribution to all tissues 4.1.3. GLUCOSE TRANSPORTERS - Glucose transporters in the small intestine: - Located on the luminal side across cytosol of enterocytes to capillary - SGLT: - Sodium-glucose linked transporters (sodium-coupled co-transporter) - Uses active transport - Located on the brush border membrane - Co-transports glucose/ galactose with sodium ion down a sodium concentration gradient generated by Na-K ATPase pump in the basolateral membrane - GLUT: - Glucose transporter - Uses facilitated transport - GLUT5: a. Located on brush border membrane, takes fructose from lumen to cytosol b. Located on basolateral side – transport cytosolic fructose out to capillaries - GLUT2: - Located on basolateral membrane - Transport of fructose, galactose, glucose into the enterohepatic vein [Liver → Peripheral circulation → Absorption by peripheral cells for metabolic need; Subjected to modulation of glucose’s presence in blood – excess amounts are removed for storage] 76 - Glucose transport in other body tissues: - GLUT1: Blood cells, Blood Brain Barrier, Foetal Cells - GLUT2: Liver, Kidney, Pancreas, Intestine - GLUT3: Neurons, Placenta - GLUT4: Muscles, Adipocytes - GLUT1, GLUT2, and GLUT3 are insulin insensitive - GLUT4 is insulin sensitive - Cellular preferences of fuel molecule: - Almost all cells utilise glucose as fuel molecules, via GLUT1 and GLUT3 - 3 storage spaces for glucose: 1. Liver – as glycogen, or as fatty acid and cholesterol in triacylglycerol 2. Muscle – as glycogen 3. Adipocytes – as triacylglycerol 1. Liver: - Uses GLUT2, a bidirectional transporter of glucose - GLUT2 is also present on renal tubular cells, pancreatic 𝛽 cells, and enterocytes - Produces and stores glycogen used for entire body 2. Muscles: - Use GLUT4, which is insulin sensitive - Glycogen mainly for use of muscles only 77 3. Adipocytes: - Uses GLUT4 - When blood glucose is high, insulin released from pancreas to blood will induce GLUT4 expression on adipocytes → facilitate the uptake of glucose - Glucose has 2 fates after entering adipocytes: 1. Acetyl-CoA for de novo fatty acid biosynthesis 2. Conversion to glycerol-3-phosphate to form backbone for triacylglycerol, storage form of lipids [LPL, ACC, DGAT are enzymes] [Pathway on the left shows lipid uptake by adipocytes] - Uptake and fate of other monosaccharides: - Galactose: - Converted into glucose and stored as glycogen - Fructose: - Taken up by liver and converted to glucose, glycogen and lactate - A fraction may be converted into fatty acid - Blood fructose concentrations always low 78 4.1.4. ANAEROBIC GLYCOLYSIS - Glucose phosphorylation: - Entry of glucose into the cell is mediated by the glucose transporter, e.g. GLUT1 - Upon entry, it is phosphorylated to glucose-6-phosphate, which is a committed step for metabolism, as it is irreversible reaction - Glycolysis: 1. Energy investment phase (investment): - ATP is hydrolysed to donate a phosphate group, facilitated by an enzyme hexokinase - Glucose converted to glucose-6-phosphate - Reaction is irreversible Intermediate: - Isomeric rearrangement to form fructose-6-phosphate - Enzyme used can catalyse both forward and backwards reaction - Hydrolysed to form second phosphate group in fructose-1,6- bisphosphate 2. Energy generation phase (productive): - F-1,6-P splits to form 2 triose biphosphates - Release of inorganic phosphate groups produces 2 ATP and 1 NADH per triose phosphate molecule - Net generation of 2 ATP and 2 NADH in glycolysis - Pyruvate/ Pyruvic acid is the end product - Anaerobic glycolysis: - In absence of oxygen, pyruvate can be reduced by LDH-A (lactic dehydrogenase-A) to lactate - Lactate is exported from the cell by a transporter called MCT (monocarboxylate transporter) - There is no net generation of NADH, there is no need for O2 79 - Fate of lactate: - Lactate mainly released from RBCs [RBCs carry oxygen but not mitochondria, so can only carry out anaerobic glycolysis] - Lactate in blood can go to heart muscles, resting skeletal muscles etc., and be converted to pyruvate for entering TCA cycle - Lactate from anaerobic glycolysis in muscles, red blood cells, and many other cells, can return to the liver to reconvert to glucose by gluconeogenesis via pyruvate, and be taken up by RBCs again (Cori cycle) 4.1.5. AEROBIC RESPIRATION 80 - Oxidative fate of pyruvate: - Pyruvate can be converted to acetyl-CoA for use in the TCA cycle - Last carbon bond from remains of original glucose is cleaved, releasing CO2 - Gets electrons in the form of hydride ions, reduces NAD+ → NADH (electron carriers for electron transport chain) - Compare to anaerobic oxidation, 30 to 32 ATP can be generated - Overview of tricarboxylic acid (TCA) cycle: 1. Pentose phosphate pathway (PPP): - Provides cellular NADPH, which functions as biochemical reductants [Regeneration of NADPH from NADP which is part of vital scheme to keeping the oxygen free radical under control in RBCs] - Produce ribose-5-phosphate required for nucleotide biosynthesis 2. UDP-glucose pathway: - Uridine-diphosphate glucose - Functions: a. Intermediate compound in glycogen production b. Channelled for use in molecular constructions, e.g. add sugars to proteins in post- translational modification 4.1.5. CLINICAL CONNECTIONS OF GLUCOSE METABOLISM Clinical connections: - All cells require glucose for metabolic functions, BUT neither glucose nor other sugars are specifically required in the diet - Glucose can be synthesised from many amino acids found in dietary protein, also from triacylglycerol - Fructose, galactose, xylulose, and all the other sugars required for metabolic processes in the human body can be synthesised from glucose (gluconeogenesis) Lactose intolerance: - Refers to a condition of pain, nausea, and flatulence after the ingestion of foods containing lactose, most notably dairy products - Although lactose intolerance is often caused by low levels of lactase, it also can be caused by intestinal injury - Lactase is usually the first one to be affected among glycosidases 81 Positron emission tomography (PET) scan: - Using radioactive tracer fluorine-18 fluorodeoxyglucose (18F-FDG) to certain cancer diagnosis - Glucose analogue as radioactive tracer - Hydroxyl group on C2 position on glucose is substituted by F-18 (18F-FDG is the analogue) - 18F-FDG is taken up by glucose using cells, phosphorylated once inside by hexokinase - Phosphorylated sugars (due to their ionic charge) cannot exit from the cell - Sugars get trapped in cells until they decay, allowing intense radioactive labelling of tissues with high glucose uptake – characteristic to many types of cancer - PDG-PET scans are used for diagnoses, staging and monitoring treatment of cancers - Case 1: John, a healthy 15-year old student, drank a huge bottle of freshly pressed tropical fruit juice on his way home from school. Later, he had diarrhoea caused by malabsorption of fruit juice. - Although disaccharides in fruit juice can be efficiently cleaved into 3 monosaccharides, only glucose in fruit can still be dealt with with a normal efficiency by getting absorbed by the active transport SGLT1 on the lumen side of enterocytes, and GLUT2 on the basolateral membrane on the small intestine - Fructose absorption is much less efficient in large amounts, so it is flushed down to the colon with other potential diarrhoea inducing agents e.g. sorbitol - Bacteria ferments them and produces excess gases, and excess water is drawn in, leading to discomfort and diarrhoea - Case 2: Ivan, a 56-year-old gentleman, has a sedentary lifestyle. He is suspected to have developed type II diabetes mellitus. Being severely overweight, he has a large waist with central obesity. He says that he cannot understand how he‘s got fat, as he never eats any fatty or deep-fried food, although he does enjoy a variety of pasta and rice dishes on a daily basis and at every meal. 82 4.1.6. ABNORMAL GLUCOSE METABOLISM - 3 main types of glucose regulation: 1. Blood glucose levels - Normal: 4 to 5.9 mmol/L - Balance – certain amount of glucose is available in the blood at all times - In the case of sudden tissue need or influx from food intake, backup resources from storage spaces can be used to release or uptake glucose through pathways of metabolism 2. Hormone levels - Natural in-built, orchestrated capacity to maintain the glucose homeostasis in blood, utilising the coordination of different hormones 3. Nerve impulses - Rapid response [No insulin induction → GLUT4 go back inside cells] - Detailed diagram: [Exercise alone can lead to increased GLUT4 on the cell surface independent of GLUT4 induction] 83 - Graph showing glucose homeostasis: - Importance of blood glucose measurement: - In the postprandial phase, insulin facilitates the transportation of glucose from the bloodstream into cells - Further, insulin enables the liver to inhibit gluconeogenesis, and facilitates the storage of glucose in the form of glycogen (glycogenesis) and fats (de novo lipogenesis) - Check and maintain crucial metabolic homeostasis and glucose balance - Method of monitoring blood glucose: - The level of glucose in the blood can be measured by applying a drop of blood to a chemically treated, disposable ‘test-strip’, which is then inserted into an electronic blood glucose metre - The reaction between the test strip and the blood is detected by the metre and displayed in units of mg/dL or mmol/L - Other principles underlying the assay of glucose: - Modern chemical methods invariably rely upon stages involving enzymes (e.g. glucose oxidase, glucose dehydrogenase, hexokinase) linked to chromogenic reactions - Others are linked to reactions featuring changes in electron flow that can be measured by suitable electronic metres - There are also less common techniques that employ physical methods for glucose detection, such as differences in infrared spectra 84 - Graph showing plasma glucose of normal vs abnormal patients: In diabetic patient, the plasma glucose level sustains high over a prolonged period of time (IV) glucose tolerance test: - Used to determine a person's ability to handle a glucose load - The test can show whether a person can metabolise a standardised measured amount of glucose - The results can be classified as normal, impaired, or abnormal [Myxoedema = hypothyroidism] - Summary: - Tight control in normal homeostasis in blood governs to keep a regular pattern of glucose tolerance upon dietary intake, or sustains a fine balance of glucose availability to meet the tissue demands - Deviance glucose tolerance pattern suggests abnormal glucose metabolism but the underlying pathophysiology can be varied 85 4.2. LIPID METABOLISM 4.2.1. LIPIDS - Insoluble in water or only slightly miscible in water - E.g. Bile salts, Eicosanoids, Steroid hormones, Triacylglycerol, Phospholipids/ sphingolipids, Vitamins (fat-soluble), Cholesterol - With various structural similarities and differences - E.g. Cholesterol: - Distinct four fused hydrocarbon ring structure - Bulky sterol structure is precursors to bile salts, steroid hormones and vitamin D - E.g. Fatty acids: - Long hydrocarbon chain with a terminal carboxyl group - Common structural feature in eicosanoids, phospholipids, sphingolipids - Basic component of triacylglycerol - Classes of lipids: [Arachidonic acids: 20-carbon long polyunsaturated fatty acids] - Structure of fatty acids: - Hydrocarbon chain predominantly hydrophobic - Terminal carboxyl group (pKa 4.8); when pH > pKa, the deprotonated form predominates - At physiological pH, COOH group ionises to form COO- 86 - COO- has a high affinity for water given the fatty acids’ amphipathic nature - Free fatty acids are highly insoluble and must be transported in the circulation in the blood associated with albumin - More than 90% of the fatty acids found in the plasma are in the form of fatty acid esters, primarily triacylglycerol, cholesterol esters and phospholipids, contained in circulating lipoprotein particles - Saturated fatty acids: - Have no double bonds - Cis double bonds cause a fatty acid to kink - Unsaturated fatty acids: - Have double bonds - Monounsaturated fatty acids: have 1 double bond - Polyunsaturated fatty acids: have >1 double bond [In humans, fatty acids are generally saturated or monounsaturated] - Nomenclature: - Short fatty acid: 2-4 carbons - Medium fatty acid: 6-12 carbons - Long fatty acid: 14-20 carbons - Very long fatty acid: ≥22 carbons - Short-hand: - Carbon is counted from the carboxylic acid end (𝛼); carbon at the other end is 𝜛 - Number in front of colon: number of carbons - Number after colon: number of double bonds - Number in brackets: position of double bonds - Omega nomenclature: - Count the position of the last double bond starting from the omega/ mesyl end - E.g. Arachidonic acid is an omega-6 fatty acid - Precursor of prostaglandins - E.g. Linoleic acid is an omega-6, essential fatty acid - Maintain membrane fluidity (of skin) - E.g. 𝛼-linolenic acid is an omega-3 essential fatty acid - For growth and development [Essential: humans lack the enzymes needed to synthesise the fatty acids, must be obtained via diet] - Others: - Fatty acids with chain lengths of 4 to 10 carbons are found in significant quantities in milk - Structural lipids and triacylglycerols contain primarily fatty acids of at least 16 carbons 87 4.2.2. TRIACYLGLYCEROLS AND TRANSPORTED FATS - Structure of triacylglycerols: - Triglycerols are only slightly soluble in water, cannot form micelles by themselves - They coalesce within white adipose tissue to form large oil droplets that are nearly anhydrous - Cytosolic lipid droplets in adipose tissue are the major energy reserve of body - Three fatty acids are esterified at their carboxyl ends to a glycerol backbone to form triacylglycerol - Micelles: - Aggregates (or supramolecular assembly) of surfactant phospholipid molecules dispersed in a liquid, forming a colloidal suspension - A single droplet of hydrophobic lipid droplets, enclosing with a shell of bile salts - For bile salt the hydrophilic side is facing the outside of the sphere - Chylomicrons: - Lipoprotein particles that consist of triacylglycerol, phospholipids, cholesterol, and proteins - From exogenous dietary lipids - Very Low Density Lipoproteins (VLDL): - Produced in the liver - Carries triacylglycerols and dietary carbohydrates from lipogenesis from liver to blood circulation - From endogenously synthesised lipids 88 4.2.3. TRIACYLGLYCEROL DIGESTION AND ABSORPTION - Triacylglycerol is the major lipid in human diet - Major sites in the GI tract: - Small intestine, lymph nodes, blood circulation, capillary walls - Peripheral tissues (liver, muscles, adipose tissues) - Limited digestion of triacylglycerols in the mouth (lingual lipase) and stomach (gastric lipase), due to low solubility of substrate - Lingual lipase – produced by cells in the back of tongue - Gastric lipase – produced by cells in the stomach - Preferentially hydrolyse short/ medium chains (≤12 carbons) - Most active in young children for the digestion of milk - Triacylglycerol is mostly digested in intestinal lumen - Lipolysed into two free fatty acids and 2-monoacylglycerol (a glycerol with a fatty acid esterified at position 2’) - Reconverted into triacylglycerols again in enterocytes and packaged in chylomicrons - Chylomicrons secreted into lymph circulation from enterocytes - Fatty acids released are taken up by muscles and adipose tissues and oxidised to CO2 and H2O 89 - Steps of triacylglycerol digestion in intestine: 1. Hydrolysis - Triacylglycerols are digested by lipoprotein lipase (LPL), enzymes found attached to capillary walls at endothelial cells - Hormone cholecystokinin is secreted by small intestine, which signals the gallbladder to release bile acids and the pancreas to release digestive enzymes [Acid-stable lipases, emulsification of other pancreatic enzymes facilitate action of lipase] - Peptide hormone secretin in small intestine secretes bicarbonate in response to the acidic materials in duodenum (pH raised to 6) - Together with colipases, pancreatic lipases is the major enzyme to carry out hydrolysis - Digest lipids of all chain lengths from carbon position 1’, 2’, or 3’ - Lipolysed into two free fatty acids and 2-monoacylglycerol (a glycerol with a fatty acid esterified at position 2’) [Hydrolysis from positions 1 and 2 of the glycerol moiety] 2. Micelle formation - Solubilisation of triacylglycerols into micelles - The lipid digestion product from enzymatic digestion partitions into the mixed micelles with bile salt in the small intestine lumen - Facilitate triacylglycerols to get across the water layer to reach the enterocytes on the intestinal lumen 3. Absorption by enterocytes - The micelles interact with the enterocytes’ membrane and allow diffusion of the lipid-soluble components to diffuse across the enterocytes’ membrane into the cells of enterocytes - Bile acids do not enter the enterocytes at this point – they remain in the intestinal lumen and travel further down before being sent back to the liver via enterohepatic circulation - Allows bile salts to be used multiple times for fat digestion - Short chain free fatty acids go directly into the blood circulation (short/ medium chain) and into the portal blood (travel bound to albumins) 90 4. Formation of chylomicrons - Triacylglycerols are insoluble in water - If allowed directly into blood, they will coalesce and impair blood flow - Lipoprotein particles like phospholipids and proteins do not easily or readily coalesce in aqueous solution - Proteins in the lipoprotein are called apolipoprotein - Other components include cholesterols and fat-soluble vitamins - The two free fatty acids and 2-monoacylglycerol are reassembled into triacylglycerols - Hydrophilic region interacts with water near the surface of the lipoprotein - Hydrophobic molecules are in the interior of the lipoprotein - Positioning of cholesterol: - Hydroxyl group of cholesterol is near the surface of the lipoprotein - In cholesterol ester, the hydroxyl group is esterified to a fatty acid - Cholesterol esters are in the core as they are synthesised by the reaction of cholesterol and activated fatty acids 5. Exocytosis of chylomicrons from enterocytes to blood circulation 4.2.4. FATE OF TRIACYLGLYCEROL AND FATTY ACIDS A. Sites of triacylglycerol storage - Adipocytes play an important role in the storage of triacylglycerols - Adipose cells specialised in synthesis and storage of triacylglycerols and for mobilisation into fuel molecules that are transported into blood - Categorised into brown and white adipose tissues - White adipose tissues stores triacylglycerols - Important in regulating energy homeostasis because it is capable of releasing triacylglycerol-derived fatty acids into bloodstream - Can be used by other tissues as energy substrate or packaged in triacylglycerols-rich lipoproteins in the liver - Brown adipose tissues dissipates energy into heat - In cytoplasm of a adipose cell, droplets of triacylglycerols coalesce to form a large globule - The globule may occupy most of the cell volume - There is also a minor accumulation of triacylglycerol in liver and muscles B. Mobilisation of triacylglycerol and fatty acids - Primary metabolic role of adipose tissue: - In adipose tissues: - Insulin stimulation is activated to both transport glucose into adipocytes (by GLUT4), and for the synthesis and secretion of LPL from the cells (LPL activated by protein C2) - LPL digests the triacylglycerols of both chylomicron and VLDL - Glycolysis occurs producing G3P, which is the substrate required for lipogenesis - G3P forms the backbone required for triacylglycerol synthesis 91 - Joining G3P, fatty acids from lipids carried by VLDL and chylomicron from intestine are esterified to form lipid droplets of triacylglycerol - Fatty acids activated, forming fatty acyl-CoA to react with G3P to form triacylglycerols [Because adipose tissue lacks glycerol kinase and cannot use the glycerol produced by LPL, the glycerol travels through the blood to the liver for the synthesis of triacylglycerols there] - Fasted state and stressed conditions: - Hormone-sensitive lipases will be activated to mobilise triacylglycerols - Insulin levels low; glucagon levels high - Intracellular cAMP: increases and activates protein kinase A which phosphorylates hormone-sensitive lipase (HSL) - Phosphorylated HSL is activated and initiates the breakdown of adipose triacylglycerols - HSL (adipose triacylglycerol lipases) cleave fatty acids from triacylglycerols - Other lipases complete the process of lipolysis - The glycerol part travels to liver and fatty acids bind to albumin to liver, muscles and other tissues to be further oxidised for energy, CO2 and H2O C. De novo fatty acid synthesis - 𝛽-oxidation of fatty acids has a high energy yield - E.g. 2 carbon from the hydrocarbon chain of palmitoyl chain is used for the 𝛽-oxidation to feed an acetyl- CoA into the TCA cycle - Each TCA cycle from the fat acetyl-CoA generate 12 ATP - 16 carbon chain can generate 129 ATP: 92 - During prolonged fasting, acetyl-CoA produced by 𝛽-oxidation of fatty acid in the liver are converted to ketone bodies which are released into the blood - Although liver constantly synthesises low level of ketone bodies, their production becomes more significant during fasting – ketone bodies needed to generate energy at peripheral tissues - E.g. ketone bodies to muscles: - 3-hydroxybutyrate is oxidised to acetoacetate, producing NADH - Acetoacetate is then provided with CoA molecule – acetoacetyl-CoA is actively removed to form two acetyl-CoA - Acetyl-CoA is used in TCA cycle to produce ATP [3-hydroxybutyrate is a secondary ketone body from acetoacetate; acetoacetate metabolises into acetone and CO2 in blood] - Extrahepatic tissues, including the brain but excluding cells without mitochondria (e.g. RBCs), can efficiently oxidise acetoacetate and 3-hydroxybutyrate - Although the liver actively producing ketone bodies, it lacks thiophorase and therefore is unable to use ketone bodies as a fuel themselves 93 - More on triacylglycerol synthesis: - The major source of carbon for fatty acid synthesis is dietary carbohydrates - When an excess of dietary carbohydrates is consumed, glucose is converted to acetyl- CoA - This provides the 2 carbon units that condense in a series of reactions on the fatty acid synthesis complex producing palmitate, then converts into other fatty acids - Fatty acid synthesis is located in the cytosol - An excess of dietary protein can also result in the increase in fatty acid synthesis - Carbon source from amino acids enter as oxaloacetate (OAA), which can be converted to acetyl-CoA or TCA cycle intermediates - Metabolic fate of lipid fuel molecules: - Dietary glucose converted through glycolysis to pyruvate: enters mitochondria, forms acetyl- CoA and OAA, condenses to form citrate - Citrate is then transported to cytosol, cleaved to form cytosol acetyl-CoA for fatty acid synthesis - Growing fatty acid chains attached to the fatty acid synthase complex in the cytosol is elongated by the sequential addition of 2 carbon units provided by malonyl-CoA - Once produced they are transported and used for various tissues for synthesis of triacylglycerol, the main storage form of fuel - Triacylglycerol is used to produce glycerol, phospholipids, and sphingolipids, which are the major components of cell membranes 4.2.5. CLINICAL CONNECTIONS OF LIPID METABOLISM - Case 1 – Mica: - M, 6 m/o - Seizure, stomach virus, not eating well - Blood glucose level 1.5 mmol/L (reference range 3.3-6.0 mmol/L) - Urine ketone bodies level negative - Indicates metabolic disease: - Medium-chain fatty acyl-CoA dehydrogenase (MCAD) deficiency - Hypoglycemic at fasting - Mechanism of MCAD deficiency: - A lack of intake of normal amount of food - The body goes to fasting conditions - Fatty acid utilisation becomes important to maintain plasma glucose level and provide ketone bodies to peripheral tissues - Mica is unable to utilise medium chain fatty acids for 𝛽-oxidation due to MCAD deficiency - Decreased acetyl-CoA production – triggering factor for activation of enzymes to kick off gluconeogenesis normally in liver to make glucose – to maintain plasma glucose levels - Impaired fatty acid 𝛽-oxidation leads to reduced ATP and NADH production – that are vital components in gluconeogenesis as well - Ketogenesis is also decreased as a result from the lack of acetyl-CoA 94 - Case 2 – Alan Marshall: - M, 44 y/o - History of alcohol abuse – alcohol-induced acute pancreatitis - Typical symptoms: abdominal pain, nausea, vomiting - Prevalence in US: ⅓ caused by alcohol insults - Mechanism: - Ethanol is shown to induce pancreatic necrosis - The damaged pancreatic acini affects the normal secretion of pancreatic amylase into the small intestine - The lack of enzymes affect normal rate of hydrolysis, such as triacylglycerol hydrolysis - In turn affects normal rate of lipid absorption at the small intestine - Induce steatorrhea (fat-rich stools) - Management: - To take commercially available pancreatic enzymes - To eat a low fat diet - To take in short-chain rather than long-chain fatty acids - Case 3 – Des Todd: - M, 18 y/o - Long sedentary summer – BMI: 27 - He is out of shape due to overeating and lack of exercise during the summer - He decides to lose weight by taking a low fat diet and doing more exercise to burn fat - Efficiency of a low fat diet: - Fat storage not entirely from dietary intake – excess amount of carbohydrates provide carbon for de novo lipogenesis - Excess amount of protein carbon skeleton from aa provide carbon for de novo lipogenesis - Efficiency of exercise: - Burn fat is a common way to mean catabolizing stored body fat for energy consumption - The stored body fat is triacylglycerol - Lipolysis mobilises triacylglycerol into free fatty acids and glycerol - 𝛽-oxidation converts fatty acids into acetyl CoA, which enters TCA cycle and ETC for ATP production - Ketone bodies can provide a quick alternative fuel source to body cells 95 4.3. AMINO ACID METABOLISM 4.3.1. METABOLISM OF EXOGENOUS AMINO ACIDS - Overview: - Amino acids are the building blocks of proteins - Obtained from two sources: - Exogenous supply from diet - Endogenous supply of constant protein turnovers - General structure of amino acids: - Exogenous supply of amino acids: - Main source of amino acid intake - Proteins of foods are broken down into free amino acids before entering the blood via the enterocytes (intestinal absorptive cells) - Digestion of proteins begins in the stomach - Activation of pepsin from pepsinogen in the stomach by the chief cells - Self-cleavage process when pH drops due to secretion of HCl - Process continues to completion in the small intestine - The pancreas (exocrine) secretes a number of zymogens which eventually become activated enzymes, e.g. trypsin, chymotrypsin, elastase, and carboxypeptidases - Trypsin is cleaved from trypsinogen by the enteropeptidase secreted by the brush border cells of the small intestine - Once trypsin is activated from trypsinogen, it cleaves the other zymogens as they enter the gastrointestinal lumen from the pancreas [“pro-” or “-ogen”: inactive forms of enzymes (zymogens)] - As gastric content of the food empties into the intestine – pH rises again by the action of bicarbonate, allowing endopeptidases to cleave the proteins into free amino acids [Exopeptidases are secreted by enterocytes. They are present inside enterocytes and on the brush borders and act on small peptides] 96 - Absorption of amino acids: - Amino acid are absorbed at the intestinal lumen - Enterocytes take in free amino acids, dipeptides and tripeptides from the intestinal lumen - Na+-dependent-carrier transports both Na+ and amino acid into enterocytes - Na+ pumped out for K+ by Na-K ATPase pump 97 - On serosal side, amino acids are carried by facilitated transport down its concentration gradient (example of secondary active transport) - Only free amino acids get across into blood circulation - Traces of polypeptides can pass into the blood - May be transported through intestinal epithelial cells by pinocytosis or by slipping between the cells lining the gut wall - May be troublesome for premature infants and lead to allergies caused by proteins in their food 4.3.2. METABOLISM OF ENDOGENOUS AMINO ACIDS - Protein turnovers: - Half-life of proteins within our body ranges from minutes to days - Example of proteins being constantly synthesised and degraded: - Haemoglobin, muscle proteins, digestive enzymes, proteins from cells shredded off from the gastrointestinal tract - Intracellular muscle degradation have 2 essential mechanisms: 1. Lysosome - Autophagy process - Unwanted intracellular components are surrounded by membranes fused with lysosomes - Inside lysosomes, cathepsin cleaves proteins into free amino acids - Free amino can leave and region the intracellular amino acid pool 2. Ubiquitin-proteasome system - Covalently linked to the small protein ubiquitin, the ubiquitin packed protein interacts with the proteasomes - Large complex that degrade proteins to small peptides in an ATP-dependent manner - Ubiquitin is released intact and recycled 98 A. Transamination: - When the amino group of an amino acid is transferred to a carbon skeleton (𝛼-keto acid), forming a new amino acid - A typical reaction coupled with amino acids transaminases and cofactors - Fate of carbon skeleton: - Carbon skeletons of excess amino acids are usually converted to glucose or triacylglycerol - Triacylglycerols usually packaged and secreted from the liver by VLDL - Glucose can be stored in glycogen or released into blood - Amino acids that can pass through the liver are converted into proteins in other tissues - Each 𝛼-keto acid can enter the TCA cycle at its appropriate points - This reaction is reversible: TCA intermediates can also be used to make amino acids - Through the TCA cycle, the liver makes it possible to use proteins as an energy source, when glucose is not available B. Deamination: 99 - Fate of nitrogen component: - Around equal amounts of ingested and excreted nitrogen to each day in adults - Possible waste product of amino acids – sources of ammonia in different parts of the body for the urea cycle - Deamination reaction: - Amino group from glutamate not taken up by 𝛼-keto acid but forms ammonium ion - Ammonia can get across cell membranes, ammonium ions cannot freely diffuse across cell membranes - Hyperammonemia, neurotoxic condition to the brain and CNS - Most tissues transfer nitrogen from amino acids to the liver for disposal as urea - This produces either: 1. Alanine from pyruvate-glucose-alanine cycle in skeletal muscles, kidney, and the intestinal mucosa 2. Glutamine from skeletal muscles, lungs, and neural tissues - Free ammonia can be taken in the form of alanine or glutamine and delivered to the liver to be made into urea 100 Urea Cycle: - Takes place in the liver hepatocytes, so urea is synthesised in the liver - Alanine and glutamine are the major nitrogen carriers of amino acid nitrogen from peripheral tissues to the liver - Helps to maintain nitrogen balance in the body so that we can get rid of excess toxic ammonia/ammonium ion [Efficient work done by the liver: ammonia and ammonium ion in blood is usually very little] - Major nitrogenous excretory product is urea - Urea is a soluble, non-toxic carrier of nitrogen - In the formation of a urea molecule, one nitrogen comes from ammonium ion that is released from deamination from glutamate, and one from aspartate - Urea can travel through the blood to the kidney to be excreted - Disorders of urea cycle leads to hyperammonemia 4.3.3. NITROGEN TRANSPORTATION BY ALANINE AND GLUTAMINE - The glucose/alanine cycle: - From the muscle, the alanine formed travels to the liver - The carbons of alanine are used for gluconeogenesis and the nitrogen is used for urea biosynthesis - This could occur during exercise or in starvation when the muscle uses blood-borne glucose - E.g. in muscles: - Every peripheral cell will have nitrogen disposal (disposal picked up by alanine and glutamine) - Conversion of alanine to glucose and urea: - Coupled reaction of alanine and 𝛼-ketoglutarate through transamination reaction - 𝛼-keto acid formed is pyruvate - Nitrogen on alanine is given to form glutamate 101 - Possible fates of nitrogen from glutamate: a. Proceed to deamination reaction to release ammonium ions (backbone is recycled) b. Proceed through transamination reaction (between glutamate and oxaloacetate) to form aspartate - Aspartate and ammonium can go into urea cycle to form urea - Alanine transfers amino groups from the skeletal muscles, kidney and gut to the liver, converted to urea for excretion - Backbone of alanine (pyruvate) can be used to make glucose 102 - Transportation by glutamine: - Glutamine can be synthesised by 𝛼-ketoglutarate accepting two ammonium ions - Glutamine can be formed in the muscles and peripheral tissue - Glutaminase converts glutamine to glutamate (to 𝛼-ketoglutarate) - This is found in the liver - This inter-organ amino acid exchange takes place when there is fasting/ at post-absorptive state: - Use of amino acid for fuel or synthesis of essential compounds/proteins - Free amino acid pool is supported by largely a net degradation of skeletal muscle proteins - Glutamine and alanine serve as amino group carriers from skeletal muscles to other tissues - Glutamine also brings ammonium to kidneys for excretion of protons and serve as fuel for kidney, gut and cells of the immune system [Alanine: after deamination, the amino group enter urea cycle, the carbon skeleton forms pyruvic acid (𝛼-keto acid)] [Glutamine: forms ammonia and glutamate] [Glutamate forms urea and 𝛼-ketoglutarate] 4.3.4. SUMMARY 103 1. Maintenance of blood amino acid pool 2. Free amino acids from dietary proteins/ endogenous protein turnovers can provide source of essential amino acids 3. Blood amino acids can be used to form new proteins or part of nucleotide synthesis 4. a. Other compounds synthesised from amino acid precursors are essential for physiological functions (nucleotides, neurotransmitters, hormones, etc.) b. Amino acids degraded to nitrogen containing urinary metabolites and do not return to the free amino acid pool 5. Amino acid carbon skeletons are recycled for gluconeogenesis and other processes for energy generation 6. Nitrogen is removed from amino acids; nitrogen in amino acid degradation primarily appears in urine as urea, ammonia or ammonium - Connection to the gut and other cellular metabolic pathways: - Protein turnovers demands a balanced supply of amino acids - Need for peptide-like molecules to get a supply of free amino acids to meet their turnovers - Amino acid metabolism takes place in all tissues 104 - Recap of the fates of amino acid components after degradation: 1. Carbon skeleton: - In the form of 𝛼-keto acids (amino acid without amino group) - Possible pathways: - Enter TCA cycle for generation of ATP directly - Converted into ketone bodies for energy usage - Converted to carbohydrates (glucose) for short-term storage (glycogen) - Converted to fatty acids (triacylglycerol) for long-term fuel storage 2. Nitrogen part: - Ammonia can also be used when urine is formed for the uptake of free hydrogen ions in the kidney - Fate of amino acids in fed state: - Fate of amino acids during fasting: - No uptake from gut - Mainly from net degradation of skeletal muscle proteins - Glutamine brings ammonium to the kidneys for the excretion of protons and serve as a fuel for the kidney that and the cell of the immune system 105 4.4. SYNTHESIS OF OTHER BIOMOLECULES - Overview: - Synthesis of other biomolecules: - Neurotransmitters and signalling molecules - Porphyrin rings (e.g. heme) - Purines and pyrimidines - Phosphocreatine - Glutathione 4.4.1. SYNTHESIS OF NEUROTRANSMITTERS - Neurotransmitters: - Chemical messengers in the body - Transmit signals from nerve cells to target cells in muscles, other nerves, glands, etc. - Play crucial roles in regulating many body functions e.g. heart rate, breathing, mood, digestion, etc. 1. Catecholamines: - Amino acid tyrosine is the precursor of the catecholamines a. Dopamine - A major regulator of the reward motivated behaviour b. Norepinephrine - Both a neurotransmitters and a hormone c. Epinephrine (adrenaline) - “Fight-or-flight” hormone 106 2. GABA: - Glutamate is the precursor of GABA, the inhibitory neurotransmitter of the CNS 3. Histamines: - Histidine is the precursor of histamine, one of the mediators of allergic reactions 4. Serotonin: - Tryptophan is the precursor of serotonin - Serotonin is known as the happy chemical/hormone - Foods like bananas and chocolates are rich in tryptophans → increase synthesis of serotonin 4.4.2. SYNTHESIS OF PORPHYRINS - Porphyrins - Porphyrin makes up heme - Heme is an essential cofactor for proteins involved in key biological processes such as oxidation, oxygen transport and storage and electron transport - E.g. Haemoglobin, cytochromes, myoglobin - Formation of porphyrins: 1. Reaction of glycine with succinyl CoA first forms the intermediate 𝛿-aminolevulinate 2. 2 molecules of 𝛿-aminolevulinate condense to form porphobilinogen 3. 4 molecules of porphobilinogen combine to form protoporphyrin 4. Iron (Fe) ion is inserted into protoporphyrin to form heme 107 - Porphyria/ vampire disease: - Mutations or misregulations of enzymes in the heme biosynthesis pathway - Precursors accumulate in red blood cells, body fluids and liver - Accumulation of precursor uroporphyrinogen I - Urine becomes discoloured (pink to dark purplish depending on light, heat exposure) - Teeth may show red fluorescence under UV light - Skin is sensitive to UV light - There is a craving for heme (insufficient production by body) - Heme is the source of bile pigments: - Heme from degradation of erythrocytes is degraded to bilirubin in two steps: 1. Heme oxygenase linearises heme to create biliverdin, a green compound (seen in a bruise). 2. Biliverdin reductase converts biliverdin to bilirubin, a yellow compound that travels bound to serum albumin in the bloodstream - Bilirubin is: - Excreted through urine and bile - Major pigment of urine (degradation to urobilin) - Further degraded by intestinal microbiota to stercobilin [Stercobilin gives the colour of faeces] 108 - Jaundice: - Yellowish pigmentation of skin, white of eyes, etc. - It can result from: - Impaired liver function (in liver cancer, hepatitis) - Blocked bile secretion (gallstones, pancreatic cancer) - Insufficient glucuronyl bilirubin transferase to process bilirubin (occurs in infants) - Treated with UV to cause photochemical breakdown of bilirubin 4.4.2. SYNTHESIS OF NUCLEOTIDES - Nucleotide biosynthesis - ATP and GTP are energy currencies in many metabolic pathways - Nucleotide pools are kept low in cells, they have to be continuously made - The synthesis may limit rates of transcription and replication in cell - Nucleotides can be synthesised in 2 ways: 1. De novo (“from the beginning”) from amino acids, ribose-5-phosphate, CO2, and NH3 2. Nucleotides can be salvaged from RNA, DNA, and cofactor degradation - De novo synthesis: - Glutamine provides most amino groups - Glycine is precursor for purines - Aspartate is precursor for pyrimidines - Sugar moiety: - Ribose-5-phosphate is made in the pentose phosphate pathway - The major catabolic fate of glucose-6-phosphate (G6P) is glycolysis to pyruvate - Pentose phosphate pathway is one of the important alternate fates of G6P: - Ribose-5-phosphate is made for the synthesis of nucleotides - NADPH is produced for providing reducing power for biosynthetic reactions - Synthesis of purine and pyrimidine nucleotides involve different pathways - Both occur mostly in the liver 109 A. Synthesis of purines: 1. De novo synthesis of purines: - Purines are built on the ribose base - An activated form of ribose, 5-phosphoribosyl 1-pyrophosphate (PRPP), is used - Synthesis begins with reaction of 5-phosphoribosyl 1-pyrophosphate (PRPP) with glutamine - Purine ring then builds up following the addition of 3 carbons from glycine - The first intermediate with a full purine ring is inosinate (IMP) - AMP and GMP are synthesised from IMP 110 [Note: - ATP is used in GMP synthesis - GTP is used for AMP synthesis - AMP and GMP can then be phosphorylated to the diphosphate (ADP and GDP) and triphosphate levels (ATP and GTP) - ATP and GTP are precursors for RNA synthesis AND energy currencies in cells] 2. Purine salvage pathways - In liver, nucleotides can be converted into free bases or nucleosides (purine bases linked to just the ribose without the phosphate group) - These can be transported to other tissues via blood - Free bases and nucleosides from diet can be absorbed and enter cells - Most cells can then salvage the bases and nucleosides to generate nucleotides 111 3. Purine degradation - Degradation of purine nucleotides (AMP and GMP) occur mainly in the liver - Enzymes in the salvage pathways are used for most of the reactions - The pathways for AMP and GMP degradation merge with xanthine production - Xanthine are then oxidised into uric acid which is excreted by the kidneys Excess uric acid – Gout: - Painful joints (often in toes) due to deposits of sodium urate crystals - Treated with avoidance of purine-rich foods (seafood, liver) - Also treated with xanthine oxidase inhibitor, allopurinol (inhibits production of uric acid from xanthine) B. Synthesis of pyrimidines: 1. De novo synthesis of pyrimidines - Unlike purine synthesis, pyrimidine synthesis proceeds by first making the pyrimidine ring and then attaching it to ribose 5-phosphate - Aspartate and carbamoyl phosphate (compounds produced in nitrogen disposal) provide the atoms for the pyrimidine ring structure - Reaction then occurs and then the first complete pyrimidine ring intermediate orotate will be made before it is attached onto the ribose-5-phosphate - PRPP, the activated form of ribose is attached to the pyrimidine ring (orotate) - The resulting nucleotide will then be converted to uridylate (UMP), the first possible pyrimidine 112 - UMP is phosphorylated to UTP - After formation of UTP, it can be converted to CTP - UTP and CTP are precursors of the synthesis of RNA - Ribonucleotides are precursors to deoxyribonucleotides - The ribose moiety in ribonucleotides will be reduced to deoxyribose (can only occur at diphosphate level for any of the nucleotides) - The reduction occurs at the diphosphate level (NDP) and is catalysed by ribonucleotide reductase - The deoxyribose nucleoside diphosphates can then be phosphorylated to triphosphate level (dNTP) - dNTPs are then used for DNA synthesis - But we cannot use dUTP for DNA synthesis, dTTP will have to be made (from dUTP) - Formation of dTTP: - dUTP is made - Dephosphorylation from dUTP to dUMP - dUMP is changed into dTMP - dTMP is phosphorylated to dTTP 113 2. Pyrimidine salvage pathways - Like purines, pyrimidine bases and nucleosides are transported to different tissues via blood circulation - Most cells can then salvage the bases and nucleosides to generate nucleotides - The pyrimidine bases are then salvaged by a 2-step route: 1. Add ribose 2. Add phosphate group 3. Pyrimidine degradation - Pyrimidine nucleotides are dephosphorylated into nucleosides - The nucleosides are then cleaved to produce ribose-1-phosphate and the free pyrimidine bases - Cytosine → Uracil → CO2 + NH4 + 𝛽-alanine - Thymine → CO2 + NH4 + 𝛽-aminoisobutyrate - The products are excreted in urine or converted to CO2, NH4 and H2O 114 4.5. GENERATION OF ATP 4.5.1. OVERVIEW OF ATP AND BIOGENETICS - Need for nutrients: - Nutrients are catabolised to feed into the reactions leading to ATP synthesis - Some nutrients form the structural components of proteins driving the ATP synthesis reactions - Need for oxygen: - Oxygen sits at the final stage of electron transport chain - Without oxygen, no ATP produced is produced, and cells cannot carry out normal functioning - Structure of ATP: - Break in phosphoanhydride bonds (phosphate bonds) generates energy - 7.3 kcal/mol is released from the break of ONE phosphoanhydride bond in ATP 115 - Bioenergetics: - The chemistry and molecular physiology of energy metabolism - Cellular energy transformations [Case 1: Mrs. C suffered a heart attack 8 months ago. She had significant loss of functional heart muscle. While walking, she occasionally experiences a crushing pain located at the centre of chest, often radiating to the neck or arms. There is a partial blockage of coronary arteries. Reasons: - Heart actively transforms ATP chemical bond energy into mechanical work (each heartbeat uses approximately 2% of ATP in the heart - However, heart muscle cells (beyond the block) receive an inadequate blood flow (carrying oxygen and nutrients) - If the heart was not able to regenerate ATP, all its ATP would be hydrolysed in