MAP 2023 - Chromatographic performance & GC.pdf
Document Details
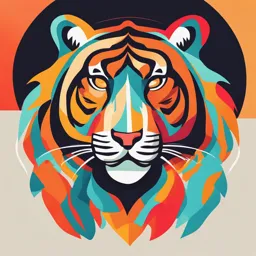
Uploaded by TolerableBliss
Vrije Universiteit Amsterdam
2023
Tags
Full Transcript
Chromatographic performance & Gas chromatography Govert W. Somsen Division of BioAnalytical Chemistry Vrije Universiteit Amsterdam [email protected] MAP 10 November 2023 band broadening in chromatography (1) x l2 Hx σl: standard deviation of peak in length units x: distance peak travelled t...
Chromatographic performance & Gas chromatography Govert W. Somsen Division of BioAnalytical Chemistry Vrije Universiteit Amsterdam [email protected] MAP 10 November 2023 band broadening in chromatography (1) x l2 Hx σl: standard deviation of peak in length units x: distance peak travelled through the column H: proportionality factor plate height H when peak has passed the entire column x = L, so: HL 2 l H l2 L H: plate height σl: standard deviation of peak in length units L: length of column plate height “the capacity of a column to minimize band broadening” abstract term ‘plate’ originates from destillation theory (there are no real plates in chromatography) narrow peak: small H wide peak: large H plate number N (1) ‘number of plates with height H’ per column in chromatographic practice, we measure retention times and peak widths (σ) in time units; transformation of equation to time units (via velocity of mobile phase u0 (in m/s)) yields: N: plate number σt: standard deviation of peak in time units tR: retention time of peak narrow peak: large N wide peak: small N plate number N (2) in chromatographic practice, peak width is measured in a chromatogram as w0.5 or wb w0.5 = 2.354σ wb = 4σ σ = w0.5 / 2.354 σ = wb / 4 resolution in HPLC (1) detector signal ΔtR time , wb,A wb,B tR,A tR,B , if for two closely spaced peaks A and B, we assume that wb,A = wb,B it follows that: RS: resolution NA: plate number of A α: selectivity factor kA: retention factor of A resolution in chromatography resolution in HPLC (4) optimizing Rs by: - increasing N: more narrow peaks - increasing α: larger difference between retention times - increasing k: more retention (up to k~10) sources of band broadening in chromatography there are three main reasons for peak broadening in the column: A different flow paths through column B molecular diffusion C slow mass transfer of analyte through mobile phase and between mobile phase and stationary phase A. flow paths in column - potential flow paths through column have different length - dependent on the followed flow path, molecules of the same compound take somewhat different time to pass the column, resulting in peak broadening for smaller stationary phase particles the difference in length of the flow paths is smaller causing less peak broadening B. molecular diffusion time peak broadening by diffusion is proportional with time : σl: standard deviation of peak in length units D: diffusion coefficient of molecule t: time degree of diffusion increases with time, so contribution to band broadening by diffusion is larger at low flow rates (long analysis time) and smaller at high flow rates (short analysis time) C. slow mass transfer between phases stationary phase mobile phase - partitioning of molecules between moving eluent and stationary phase does not occur infinitely fast (takes some time) - molecules need to diffuse from moving eluent into stationary phase and vice versa - effect is less strong using smaller stationary phase particles as average distances between the phases is smaller partitioning takes time, so contribution to band broadening is smaller at low flow rates (long analysis time) and larger at high flow rates (short analysis time) JJ van Deemter Chemical Engineering Science 5 (1956) 271 KSLA van Deemter equation narrow peak: small H wide peak: large H B H A Cu u H: plate height A,B,C: column constants u: mobile phase velocity or linear flow rate (cm/s) A: flow paths B: diffusion C: mass transfer there is an optimum flow rate for which H is minimal (for HPLC at u ~ 1 mm/s) H-u curve: optimal u0 u0: mobile phase velocity H low u0 - high H, low N - broad peaks - very long analysis times bad and slow separations high u0 - high H, low N - wider peaks - short analysis times not optimal, but fast separation higher u0 - higher H, lower N - bit wider peaks - reasonable analysis times sub-optimal separation u0 optimal u0 - minimum H, maximum N - most narrow peaks - long analysis times optimal separation optimal u0 • 0.5 - 2 mm/s for LC • 100 - 500 mm/s for cGC size of stationary phase particles in HPLC B H A Cu u dp: diameter of stationary phase particles A dp B is independent of dp C d p2 use of small stationary phase particles gives lower H, and therefore more narrow peaks and thus better separations, or same separation in less time effect of dp in HPLC H (µm) in HPLC there is a trend towards smaller and smaller stationary phase particles (10 µm, 5 µm, 3 µm, 1.7 µm, .....) state-of-the-art effect of dp dp H = 0.0229 mm H = 0.0161 mm H = 0.0115 mm H = 0.0078 mm L N = 6500 N = 6200 N = 6500 N = 6400 smaller dp same resolution with shorter column in shorter time HPLC injector tubing pump mobile phase reservoir column UV absorbance detector data system waste HPLC instrument flow rate pump delivers an adjustable constant flow rate F (mL/min) V0: column void volume F = V0/t0 V0 = ε0 · 0.25π ε0: fraction of column volume filled with mobile phase · dc2 · L dc: column inner diameter u0 = L/t0 F = ε0 · 0.25π type of LC dc (mm) flow rate F conventional LC 4.6 1 mL/min microbore LC 2.0 0.2 mL/min capillary LC 0.3 5 μL/min nano LC 0.075 300 nL/min · dc2 · u0 typical ε0: 0.7 for porous particles typical u0: 1.5 mm/s column pressure pressure needed to pump mobile phase through the column: P B0 L d 2 p u0 ΔP: pressure u0: mobile phase velocity (cm/s) η: viscosity of mobile phase L: length of column B0: permeability factor typical B0: 1000 for porous particles dp: diameter of stationary phase particles 500 for non-porous particles small stationary phase particles lead to (very) high column pressures use of columns with particles of 1.7 µm requires special equipment and pumps that can provide ultra high pressures (>1000 bar): UPLC of UHPLC sample injection in HPLC absorbance detection in HPLC UV light detector Next: gas chromatography gas chromatography differential partitioning of gaseous analytes between two phases non-volatile liquid or solid stationary phase stands still, does not move A stat gaseous mobile phase moves along the stationary phase A mob in mobile phase: analyte is in the gas phase (high temperature needed) retention is basically a function of boiling point of analyte: more retention for analyte with higher boiling point mobile phase acts as carrier gas: is inert and has no interaction with analyte and virtually no effect on retention basic GC set-up GC process - volatile liquid or gaseous sample is injected through septum in heated port where it rapidly evaporates - vapor is swept through column by carrier gas - column is hot enough to provide sufficient vapor pressure for analytes - separated analytes flow through hot detector GC of beer vapor gaseous mobile phase inert mobile phase: nitrogen (N2), hydrogen (H2) or helium (He) gas is non-viscous: low pressure needed for gas flow analytes have high diffusion coefficients in gas phase gas allows the use of open-tubular columns with relatively large column internal diameters gas allows use of (very) long columns or high flow rates results in high plate numbers and/or short analysis times however: limited application only applicable to volatile and thermostable analytes non-volatile/thermolabile analytes only after chemical derivatization non-volatile sample matrices cause problems aqueous samples are notoriously difficult analyte derivatization example trimethylsilylation trimethylsilylated products are more amenable to GC analysis - analyte is first extracted to solvent suitable for derivatization reaction - derivative should be in GC-compatible solvent prior to injection GC: wall-coated columns wall-coated open tubular (WCOT): standard format in GC stationary phase film fused-silica capillary thin film of stationary phase (0.05 - 5 µm) on inner column wall gas flow GC column in oven GC columns - fused-silica capillaries - internal diameter: 50 - 500 µm - length: 10 - 100 m fused-silica flexible unbreakable due to polyimide outer coating (stable up to 375-400 °C) very inert low metal content (no adsorptive places and catalytic sites) with thin film of stationary phase on inner wall: low number of active silanol groups (adsorptive places) stationary phase mostly based on polysiloxane R is e.g. methyl, phenyl or cyanopropyl nature of R-groups determines: - the polarity - the selective interactions between analyte and stationary phase highest retention (and selectivity) is obtained when analyte and stationary phase have similar properties: - apolar compounds on apolar phases - polar compounds on polar phases GC retention stationary phase dimethylpolysiloxane (non-polar) column oven temperature 70 ˚C Amob Astat boiling point Tb (˚C) hexane 68.7 heptane 98.4 octane 125.7 GC retention and boiling point GC of n-alkanes (C10-C16) stationary phase dimethylpolysiloxane C16 column oven temperature 373 K (100 °C) C10 boiling point Tb (K) ln k ~ Tb Tb : boiling point analyte (K) k : retention factor analyte retention and column oven temperature k Toven (K) k as function of column oven temperature k Toven (K) retention factor of analyte A (kA) with boiling point Tb,A at different column oven temperatures Toven: ln 𝑘 𝑘 , , , , = 85𝑇 𝑅 1 , 𝑇 − , 1 𝑇 R: gas constant = 8.314 J/molK , T: absolute temperature (K) separation as function of difference in boiling point k Toven (K) selectivity factor α for two analytes (A and B) with different boiling ln 𝑘 85 = ln 𝛼 = 𝑘 𝑅𝑇 𝑇 , point Tb at column oven temperature Toven: −𝑇 , plate height H for open tubular columns Van Deemter equation: no particles (no Eddy diffusion; no A term): H A H B C u0 u0 B C u0 u0 u0: velocity of mobile phase H = HB + HC HB 2 D m u0 HC Dm: diffusion coefficient of analyte in mobile phase d 2f d c2 u0 g( k ) u0 f ( k ) Dm Ds dc: Ds: df: f(k),g(k): column inner diameter diffusion coefficient of analyte in stationary phase film thickness stationary phase constants depending on analyte retention factor k Golay equation for open tubular GC columns d 2f 2 Dm d c2 H f ( k ) u0 g( k ) u0 u0 Dm Ds u 0: linear velocity of mobile phase Dm: diffusion coefficient of analyte in mobile phase dc: column inner diameter Ds: diffusion coefficient of analyte in stationary phase df: film thickness stationary phase f(k): constant depending on analyte retention factor k g(k): constant depending on analyte retention factor k effect of column diameter dc on H d 2f 2Dm d c2 H f ( k ) u0 g( k ) u0 u0 Dm Ds lowest H for small dc at higher flow rates: narrow peaks in short analysis times! effect of carrier gas on H diffusion constant Dm of analyte is carrier gas dependent d 2f 2Dm d c2 H f ( k ) u0 g( k ) u0 u0 Dm Ds optimum H for He and H2 at higher flow rates: shorter analysis times! Dm,N2 < Dm,He < Dm,H2 He and H2 are preferred carrier gases column length L H depends on k 1 6 k 11 k 2 f(k ) 96 ( 1 k ) 2 g( k ) 2k 3( 1 k ) 2 d 2f 2Dm d c2 H f ( k ) u0 g( k ) u0 u0 Dm Ds H (cm) k = 5.0 k = 1.0 k = 0.5 u0 (cm/s) low retention factor k is favorable for low H (more narrow peaks) decrease k by using higher oven temperature: use temperature gradient ! column oven temperature programming final T T T-gradient cooling down start T time • separation of analytes with wide range of boiling points • sharp peaks (high N) for all analytes • high peak capacity (room for many peaks) temperature programming column: 15 m x 0.32 mm; 0.25 µm carrier gas: helium, 30 cm/s column oven temperature 100 °C 1. 2. 3. 4. 5. 6. 7. n-decane (Tb, 174 ˚C) n-undecane n-dodecane n-tridecane n-tetradecane n-pentadecane n-hexadecane (Tb, 287 ˚C) column oven temperature 50 °C for 1 min 50-120 °C at 20 °C/min sharp peaks for all compounds in much shorter analysis times! temperature programmed GC high resolution and fast GC long columns for high peak capacity column: 30 m x 250 µm T-gradient: 50-290 oC, 23 oC/min 93 peaks in 16 min short columns for fast GC column: 30 cm x 50 µm T: constant 9 peaks in 0.64 s flame ionization detector (FID) most used GC detector • carrier gas mixed with hydrogen • mixed with air and ignited: flame • combustion of organic compounds produces free electrons • signal proportional to flux of C-atoms • almost universal response (anything that contains C) • uniform sensitivity for all organics (98 – 102%) • quantification without calibration possible • sensitive: low LOD’s • wide linear range (> 105) GC vs. HPLC GC HPLC • for volatile analytes (or made volatile by derivatization) • non-volatile and thermo-labile analytes • very high peak capacity (capillary GC, T-programmed) • high detector sensitivity • main application areas: petrochemichal industry environmental analysis • better selectivity (more options) • larger sample volumes possible • main application areas: pharmaceutical industry biomedical and clinical analysis food analysis polymers