Basic Biomechanics PDF
Document Details
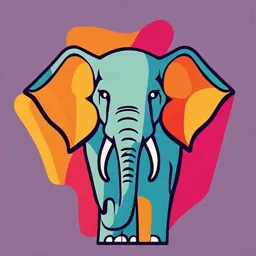
Uploaded by AttentiveSalmon
Tags
Summary
This book chapter introduces basic biomechanics. It discusses the principles of mechanics applied to the human body, including laws of motion, torque, stability, simple machines, levers, and pulleys.
Full Transcript
2363_Ch08-093-112.qxd 12/9/10 5:35 PM Page 93 8 C H A P T E R Basic Biomechanics Laws of Motion The human body, in many respects, can be referred to as...
2363_Ch08-093-112.qxd 12/9/10 5:35 PM Page 93 8 C H A P T E R Basic Biomechanics Laws of Motion The human body, in many respects, can be referred to as a living machine. It is important when learning about Force how the body moves (kinesiology) to also learn about the Torque forces placed on the body that cause the movement. As illustrated in Figure 8-1, mechanics is the branch of Stability physics dealing with the study of forces and the motion Simple Machines produced by their actions. Biomechanics involves tak- ing the principles and methods of mechanics and apply- Levers ing them to the structure and function of the human Pulleys body. As mentioned in Chapter 1, mechanics can be divid- ed into two main areas: statics and dynamics. Statics Wheel and Axle deals with factors associated with nonmoving or nearly Inclined Plane nonmoving systems. Dynamics involves factors associat- ed with moving systems and can be divided into kinetics Points to Remember and kinematics. Kinetics deals with forces causing move- Review Questions ment in a system, whereas kinematics involves the time, space, and mass aspects of a moving system. Kinematics can be divided into osteokinematics and arthrokinemat- ics. Osteokinematics focuses on the manner in which bones move in space without regard to the movement of joint surfaces, such as shoulder flexion/extension. Arthrokinematics deals with the manner in which Mechanics/ Biomechanics Statics Dynamics Kinematics Kinetics Osteokinematics Arthrokinematics Figure 8-1. Mechanics/biomechanics relationship flowchart. 93 2363_Ch08-093-112.qxd 12/9/10 5:35 PM Page 94 94 PART I Basic Clinical Kinesiology and Anatomy adjoining joint surfaces move in relation to each other— stay in motion when the car stopped. Unfortunately, that is, in the same or opposite direction. many of the people with neck injuries from automobile Various mechanical terms must be defined before accidents have demonstrated this law. beginning to discuss these topics. Force is a push or A force is needed to overcome the inertia of an object pull action that can be represented as a vector. A vector and cause the object to move, stop, or change direction. is a quantity having both magnitude and direction. For The object’s acceleration depends on the strength of the example, if you were to push a wheelchair, you would force applied and the object’s mass. For example, kick a push it with a certain speed and in a certain direction. soccer ball and it will roll along the grass. If no forces Velocity is a vector that describes speed and is meas- act on it, the ball will roll forever. However, the force of ured in units such as feet per second or miles per hour. friction acting on the ball causes the ball to eventually A scalar quantity describes only magnitude. Common stop. There is friction between any two surfaces. In this scalar terms are length, area, volume, and mass. Everyday case, it is the friction of the grass on the surface of the examples would be units such as 5 feet, 2 acres, 12 fluid ball that causes the ball to stop rolling. ounces, and 150 pounds. Mass refers to the amount of A soccer ball can also be used to demonstrate matter that a body contains. In this example, the amount Newton’s second law. First, mildly kick the ball and of matter within and making up the body is the mass. notice how far it travels. Next, kick the ball about twice Inertia is the property of matter that causes it to resist as hard as the first kick. Notice that the ball will travel any change of its motion in either speed or direction. approximately twice as far. Acceleration is any change Mass is a measure of inertia—its resistance to a change in in the velocity of an object. The soccer ball is accelerat- motion. ing when it starts moving. If you were to kick the ball Kinetics is a description of motion with regard to again even harder, it would travel proportionately far- what causes motion. Torque is the tendency of force to ther. This is Newton’s second law of motion, the law of produce rotation around an axis. Muscles within the acceleration: The amount of acceleration depends on body produce motion around joint axes. Friction is a the strength of the force applied to an object. force developed by two surfaces, which tends to prevent Acceleration can also deal with a change in direction. motion of one surface across another. For example, if Force is needed to change direction; according to the you slide across a carpeted floor in your stocking feet, law, the change in an object’s direction depends on the there will be so much friction between the two surfaces force applied to it. that you won’t slide very far. However, if you slide across Another part of Newton’s second law deals with the a highly polished hardwood floor in your stocking feet, mass of an object. Mass is the amount of matter in an there will be very little friction between these two sur- object. Acceleration is inversely proportional to the mass faces and you will have a good slide. of an object. If you apply the same amount of force to two objects of differing mass, the object with greater mass will accelerate less than the object with less mass. You can Laws of Motion demonstrate this by first rolling a soccer ball, then rolling a bowling ball with the same amount of force. The heav- Motion is happening all around you—people walking, ier bowling ball will not travel nearly as far. cars traveling on highways, airplanes flying in the air, Newton’s third law of motion, the law of action- water flowing in rivers, balls being thrown, and so on. reaction, states that for every action there is an equal Isaac Newton’s three laws explain all types of motion. and opposite reaction. The strength of the reaction is Newton’s first law of motion states that an object at rest always equal to the strength of the action, and it occurs tends to stay at rest, and an object in motion tends to in the opposite direction. This can be demonstrated by stay in motion. This is sometimes referred to as the law jumping on a trampoline. The action is you jumping of inertia, because inertia is the tendency of an object down on the trampoline. The reaction is the trampoline to stay at rest or in motion. To demonstrate this law, pushing back with the same amount of force. This causes consider riding in a car. If the car moves forward quickly you to rebound up in the opposite direction that you from a starting position, your body pushes against the jumped. The harder you jump, the higher you rebound. back of the seat and your neck probably hyperextends. As stated, no motion can occur without a force. There Your body was at rest before the car moved, and it tend- are basically two types of force that will cause the body ed to stay at rest as the car started to move. If the car is to move. Forces can be internal, such as muscular con- moving and then stops suddenly, your body traction, ligamentous restraint, or bony support. Forces is thrown forward and your neck goes into extreme can also be external, such as gravity or any externally flexion, because your body was in motion and tended to applied resistance such as weight, friction, and so on. 2363_Ch08-093-112.qxd 12/9/10 5:35 PM Page 95 CHAPTER 8 Basic Biomechanics 95 Force Point of application Force is one of those concepts that everyone under- stands but is difficult to define. To create a force, one object must act on another. Force can be either a push, which creates compression, or a pull, which creates ten- sion. Movement occurs if one side pushes (or pulls) Force A harder than the other. Forces are vector quantities. A vector quantity describes both magnitude and direction. A person pulling a heavy load with a rope is an example of a vec- tor. The tension in the rope represents the vector’s mag- nitude, and the direction of the pull on the rope repre- sents the vector’s direction. A vector force can be shown graphically by a straight Force B line of appropriate length and direction. Figure 8-2 Figure 8-2. Concurrent force system. Two people pushing shows two people (representing forces) pushing on the at different angles to each other through a common point of chest of drawers, but at right angles to each other. The application. characteristics of force include the following: example of parallel forces would be the three-point 1. Magnitude (each person is pushing equally in pressures of bracing (Fig. 8-4). Two forces—in this case, this case) X and Y—are parallel to each other and pushing in the 2. Direction (shown by the arrow) same direction, while a third parallel force (Z), the back 3. Point of application (the same for both people) brace, is pushing against them. This middle force must Forces can be described by the effect they produce. A always be located between the two parallel forces. To be linear force results when two or more forces are acting effective, the middle force must be of sufficient along the same line. Figure 8-3A shows two people strength to resist the other two forces. You could also pulling a boat with the same rope in the same direction. say that the two forces must be of sufficient strength to Figure 8-3B shows two people pulling on the same rope resist the middle force. but in opposite directions. Parallel forces occur in the To produce concurrent forces, two or more forces same plane and in the same or opposite direction. An must act on a common point but must pull or push in A B Figure 8-3. Linear forces. (A) Two people pulling in same direction. (B) Two people pulling in opposite directions. 2363_Ch08-093-112.qxd 12/9/10 5:35 PM Page 96 96 PART I Basic Clinical Kinesiology and Anatomy e t rc n Point of fo ulta application es R X Force A Z Y Force B Figure 8-5. A parallelogram shows graphically the resultant force of two concurrent forces pushing on a chest of drawers. Figure 8-4. Parallel forces of body brace. Forces X and Y are parallel in the same direction, while force Z is parallel but in the opposite direction. Force Z must be between forces X and Y to provide stability. If force Z was at either end, instead of in the middle, motion would occur. Resultant different directions, such as the two people pushing on force the cabinet in Figure 8-5. The overall effect of these two different forces is called the resultant force and lies somewhere in between. Because forces can be represented as vectors, they can be shown graphically using what is called the parallelo- Anterior Posterior gram method. Using Figure 8-5 as an example, first draw deltoid deltoid in vectors for the two forces (solid lines). Secondly, com- plete the parallelogram using dotted lines. Next, draw in the diagonal of the parallelogram (middle line and arrow). This diagonal line represents the resultant force. An example of resultant force in the body is the ante- rior and posterior parts of the deltoid muscle (Fig. 8-6). Although both parts have a common attachment (the insertion), they pull in different directions. When both parallel forces are equal, the resultant force causes the shoulder to abduct. If the pull of the two forces were not equal (i.e., if the pull of the anterior deltoid were stronger than that of the posterior), the resultant force would produce motion more in the direction of the ante- rior deltoid (Fig. 8-7). The shoulder would flex and Figure 8-6. Resultant force of equal forces of anterior abduct diagonally in a forward and outward direction. and posterior deltoid muscles. 2363_Ch08-093-112.qxd 12/9/10 5:35 PM Page 97 CHAPTER 8 Basic Biomechanics 97 Resultant force Anterior Trapezius deltoid Posterior (upper) deltoid Figure 8-7. Resultant force of unequal forces moves toward the stronger force. Serratus anterior A force couple occurs when two or more forces act in different directions, resulting in a turning effect. In Figure 8-8, notice that the upper trapezius pulls up and in, the lower trapezius pulls down, and the serratus ante- rior pulls out. The combined effect is that the scapula Trapezius rotates. (lower) Figure 8-8. Force couple of muscles rotating the scapula. Torque Torque, also known as moment of force, is the ability of force to produce rotation around an axis. It can be Line of pull thought of as rotary force. The amount of torque a lever has depends on the amount of force exerted and the dis- tance it is from the axis. Use of a wrench demonstrates torque. The twisting force (torque) exerted by the wrench can be increased either by 1. increasing the force applied to the handle, or 2. increasing the length of the handle. Center of joint Moment arm Torque is also the amount of force needed by a mus- Figure 8-9. Moment arm of biceps is the perpendicular cle contraction to cause rotary joint motion. distance between the muscle’s line of pull and the center of How much torque can be produced depends upon the the joint. strength of the force (magnitude) and its perpendicular distance from the force’s line of pull to the axis of rota- tion. That perpendicular distance is called the moment Fig. 8-10B). This is because the perpendicular distance arm, or torque arm (Fig. 8-9). Therefore, the moment arm between the joint axis and the line of pull is very small. of a muscle is the perpendicular distance between the Therefore, the force generated by the muscle is primarily muscle’s line of pull and the center of the joint (axis of a stabilizing force, in that nearly all of the force gener- rotation). Torque is greatest when the angle of pull is at ated by the muscle is directed back into the joint, 90 degrees (Fig. 8-10A), and it decreases as the angle of pulling the two bones together. pull either decreases (Fig. 8-10B) or increases (Fig. 8-10C) Contrary to that, when the angle of pull is at 90 degrees from that perpendicular position. (see Fig. 8-10A), the perpendicular distance between the No torque is produced if the force is directed exactly joint axis and the line of pull is much larger. Therefore, through the axis of rotation. Although this is not quite the force generated by the muscle is primarily an angular possible for a muscle, it comes very close. For example, force, or movement force, in that most of the force gener- if the biceps contracts when the elbow is nearly or com- ated by the muscle is directed at rotating, not stabilizing, pletely extended, there is very little torque produced (see the joint. 2363_Ch08-093-112.qxd 12/9/10 5:35 PM Page 98 98 PART I Basic Clinical Kinesiology and Anatomy throughout the range, and therefore are more effective at stabilizing the joint than moving it. The coraco- brachialis of the shoulder joint is a good example (see Fig. 10-17). Its line of pull is mostly vertical and quite close to the axis of the shoulder joint. Therefore, it has Line of pull a very short moment arm, which makes this muscle more effective at stabilizing the head of the humerus in the shoulder joint than at moving the shoulder joint. The angular force of the quadriceps muscle is increased by the presence of the patella. The patella, a Joint axis sesamoid bone encapsulated in the tendon, increases the Moment moment arm of the quadriceps muscle by holding A arm the tendon out and away from the femur. This changes the angle of pull, allowing the muscle to have a greater angular force (Fig. 8-11A). Without a patella, the moment arm is smaller, making the muscle’s line of pull more vertical, and much of the force of the quadriceps is directed back into the joint (Fig. 8-11B). Although this is good for stability, it is not effective for motion. To have effective knee motion, it is vital that the quadriceps pro- vide a strong angular force. In summary, if the moment arm is greater, then the angular force (torque) is also greater. Moment arm is determined by measuring the perpendicular distance between the joint axis and the muscle’s line of pull. If the joint angle is near 0 degrees (almost straight), the moment arm is small and the force is a stabilizing action that moves the two bones of the joint together. If B C the joint angle is nearer 180 degrees (completely bent), the moment arm is small and the force is dislocating, Figure 8-10. Effect of moment arm on torque. (A) Moment arm and angular force are greatest at 90 degrees. (B) As joint moves toward 0 degrees, moment arm decreases and stabiliz- Line Line ing force increases. (C) As joint moves beyond 90 degrees and of pull of pull toward 180 degrees, moment arm decreases and dislocating force increases. As a muscle contracts through its range of motion (ROM), the amount of angular or stabilizing force changes. As the muscle increases its angular force, it Center Center decreases its stabilizing force and vice versa. At of joint of joint 90 degrees, or halfway through its range, the muscle has its greatest angular force. Past 90 degrees, the stabiliz- ing force becomes a dislocating force, because the force is directed away from the joint (see Fig. 8-10C). In Moment Moment Figures 8-10B and C, when the stabilizing and dislocat- arm arm ing forces are increasing, the angular (rotating) force is decreasing. Stated another way, a muscle is most efficient at moving, or rotating, a joint when the joint is at or near 90 degrees. It becomes less efficient at moving or rotating when the joint angle is at the beginning or A B near the end of the joint range. Some muscles have a Figure 8-11. Moment arm of quadriceps muscle with a much greater stabilizing force than angular force patella (A) and without a patella (B). 2363_Ch08-093-112.qxd 12/9/10 5:35 PM Page 99 CHAPTER 8 Basic Biomechanics 99 pulling the two bones away from each other. If the joint In the human body, the COG is located in the midline angle is in the midrange of motion, the moment arm is at about the level of, though slightly anterior to, the sec- greatest, and the ability to move the joint is strongest. ond sacral vertebra of an adult. Because body proportions Moment arm, size of the muscle, and contractile change with age, the COG of a child is higher than that of strength of the muscle all determine how effective a an adult. To demonstrate this, move your right arm up muscle is in causing joint motion. over your head and touch your left ear (Fig. 8-13A). Now, ask a 3-year-old to do the same. You will notice that while you can easily touch your ear, the child’s hand Stability reaches only to about the top of the head (Fig. 8-13B). The child’s head is much larger in proportion to the When an object is balanced, all torques acting on it are arms and rest of the body. even and it is in a state of equilibrium. How secure or As a point of interest, height-arm span is a body pro- precarious this state of equilibrium is depends prima- portion made famous by the illustration of Leonardo rily on the relationship between the object’s center of da Vinci. The length of an adult’s outstretched arms is gravity and its base of support. To understand the equal to his or her height (Fig. 8-14). principles of stability, certain terms must be defined. Base of support (BOS) is that part of a body that is in Gravity is the mutual attraction between the earth and contact with the supporting surface. If you outlined the an object. Gravitational force is always directed verti- surface of the body in contact with the ground, you would cally downward, toward the center of the earth. have identified the BOS. Line of gravity (LOG) is an Practically speaking, gravitational force is always imaginary vertical line passing through the COG toward directed toward the ground. Center of gravity (COG) the center of the earth. These are shown in Figure 8-15. is the balance point of an object at which torque on all sides is equal. It is also the point at which the planes of the body intersect, as shown in Figure 8-12. Sagittal plane Transverse plane Center of gravity Frontal plane A B Figure 8-13. Body proportions change as a person grows. Figure 8-12. The center of gravity is the point at which the (A) Adult can reach over top of head to touch opposite ear. three cardinal planes intersect. (B) Child can reach over head only part way. 2363_Ch08-093-112.qxd 12/9/10 5:35 PM Page 100 100 PART I Basic Clinical Kinesiology and Anatomy 5' 9" Stable A 5' 9" Unstable B Figure 8-14. In an adult, arm span and body height are equal. Neutral C Figure 8-16. Three states of equilibrium: (A) stable, (B) unstable, and (C) neutral. LOG There are basically three states of equilibrium (Fig. 8-16). Stable equilibrium occurs when an object is in a position where disturbing it would require its COG to be raised. A simple example is that of a brick. When the widest part of the brick is in contact with the surface (BOS), it is quite stable (Fig. 8-16A). To disturb it, the brick would have to be tipped up in any direction, thus raising its COG. The same could be said of a person lying flat on the floor. Unstable equilibrium occurs when COG only a slight force is needed to disturb an object. Balancing a pencil on its pointed end is a good example. A similar example is that of a person standing on one leg. Once balanced, it takes very little force to knock over the pencil or person (Fig. 8-16B). Neutral equilibrium exists when an object’s COG is neither raised nor lowered when it is disturbed. A good example is a ball. As the ball rolls across the floor, its COG remains the same (Fig. 8-16C). A person moving across the room while seated in a wheel- chair demonstrates neutral equilibrium. The following principles demonstrate the relation- ships between balance, stability, and motion: BOS 1. The lower the COG, the more stable the object. In Figure 8-17, both triangles have the same base of Figure 8-15. Center of gravity (COG), line of gravity support. However, the triangle on the left is taller, (LOG), and base of support (BOS). has a higher COG, and thus is more unstable 2363_Ch08-093-112.qxd 12/9/10 5:35 PM Page 101 CHAPTER 8 Basic Biomechanics 101 LOG Force COG Force Base of support A B A Figure 8-17. Relationship of height of center of gravity to stability. (A) Higher COG is less stable. (B) Lower COG is more stable. than the triangle on the right. It would take less force to disturb the taller triangle. 2. The COG and LOG must remain within the BOS for an object to remain stable. (Keep in mind that the LOG passes through the COG. Therefore, B what can be said of one can be said of the other. For the purpose of clarity, from this point for- ward, the term COG will be used.) The wider the BOS, the more stable the object. In the example in Figure 8-18A, the book is resting entirely on its BOS (tabletop) and is quite stable. As you push it off the edge (Fig. 8-18B), it becomes less stable. When its COG is no longer over its BOS (Fig. 8-18C), the book will fall. Another example is a woman standing upright on both feet (Fig. 8-19A). Her COG lies at or near C the center of the base of support. As she leans to Figure 8-18. Relationship of COG to BOS. (A) The book is very stable because its COG is in the middle of its BOS. the side (Fig. 8-19B), her COG moves toward the (B) The book is less stable because its COG is near the border of her BOS. As soon as her COG passes edge of its BOS. (C) The book is unstable and will fall beyond the BOS, she becomes unstable, and if because its COG is beyond its BOS. her posture is not corrected or if her BOS is not widened, she will fall. To lean farther without los- ing her balance, she could either raise her oppo- 5. The greater the friction between the supporting sur- site arm or widen her stance. In either case, her face and the BOS, the more stable the body will be. COG would move back over her BOS. Walking on an icy sidewalk is a slippery experience, 3. Stability increases as the BOS is widened in the because there is essentially no friction between the direction of the force. A person standing at a bus ice and the shoe. Sanding the sidewalk increases the stop on a very windy day would be more stable friction of the icy surface, thus improving traction. when facing into the wind and placing one foot Having a surface with a great deal of friction is not behind the other, thus widening the BOS in the always desirable. Pushing a wheelchair across a direction of the wind (Fig. 8-20). hardwood floor is much easier than pushing one 4. The greater the mass of an object, the greater its across a carpeted floor. The carpet creates more stability. This concept is observed by looking at friction, making it harder to push the wheelchair. the size of players on a football team. Linebackers 6. People have better balance while moving if they are traditionally heavier, and thus harder to push focus on a stationary object rather than on a over, but they are not particularly fast. Halfbacks, moving object. Therefore, people learning to walk whose job is to run with the ball, are much lighter with crutches will be more stable if they focus on (and easier to push over). It can be said that what an object down the hall rather than look down at is gained in stability is lost in speed and vice versa. their moving feet or crutches. 2363_Ch08-093-112.qxd 12/9/10 5:35 PM Page 102 102 PART I Basic Clinical Kinesiology and Anatomy A B Figure 8-19. Relationship of COG to BOS. (A) She is Figure 8-20. Wider base of support in direction of force stable—her COG is in the middle of her BOS. (B) She is increases stability. less stable because her COG is near the edge of her BOS. Simple Machines of motion), but not both. However, the basic rule of all simple machines is that the advantage gained in power In engineering, various machines are used to change the is lost in distance. Sometimes, a great deal of power is magnitude or direction of a force. The four simple needed, such as moving a heavy rock. Other times, dis- machines are the lever, the pulley, the wheel and axle, tance (range of motion) is needed, such as swinging a and the inclined plane. Examples of each of these tennis racket. Wheelbarrows, crowbars, manual can machines, except for the inclined plane, can be found in openers, scissors, golf clubs, and playground seesaws the human body. The lever, the wheel and axle, and the are but a few examples of levers. Different types of levers inclined plane allow a person to exert a force greater can also be found in the human body. Each type of lever than could be exerted by using muscle power alone; the will favor power or distance, but not both. pulley allows force to be applied more efficiently. This To understand the structure and function of levers, increase in force is usually at the expense of speed and you should be familiar with certain terms. A lever is can be expressed in terms of mechanical advantage, rigid and can rotate around a fixed point when a force which will be described later. is applied. A bone is an example of a lever in the human body. The fixed point around which the lever rotates is the axis (A), sometimes referred to as the fulcrum. In the Levers body, the joint is the axis. The force (F), sometimes There are three classes of levers, each with a different called the effort, that causes the lever to move is usually purpose and a different mechanical advantage. We use muscular. The resistance (R), sometimes called the levers daily to help us accomplish various activities. load, that must be overcome for motion to occur can Usually a lever will favor either power or distance (range include the weight of the part being moved (arm, leg, 2363_Ch08-093-112.qxd 12/9/10 5:35 PM Page 103 CHAPTER 8 Basic Biomechanics 103 etc.), the pull of gravity on the part, or an external weight being moved by the body part. When determin- ing a muscle’s role (force or resistance), it is important to use the point of attachment to the bone, not the A F A R muscle belly, as the point of reference. When determin- ing the resistance of the part, use its COG. The force arm (FA) is the distance between the force and the axis, while the resistance arm (RA) is the dis- B tance between the resistance and the axis (Fig. 8-21). F A R The arrangement of the axis (A) in relation to the force Figure 8-22. First-class lever. FAR (F = force; A = axis; (F) and the resistance (R) determines the type of lever. R = resistance). (A) A is closer to R. (B) A is closer to F. The longer the FA, the easier it is to move the part. Conversely, the longer the RA, the harder it is to move the part. Remember, there is always a trade-off. With have to push the ruler down very far. You have just the longer FA, the part will be easier to move, but the demonstrated that with a longer FA (or a shorter RA), FA will have to move a greater distance. When the RA 1. It is easy to move the resistance (book), is longer, it won’t have to move as far, but it will be 2. The resistance is moved only a short distance, and harder to move. 3. The force has to be applied through a long Classes of Levers distance. In a first-class lever, the axis is located between the However, with a shorter FA (or a longer RA), force and the resistance: 1. It is harder to move the resistance, First-class lever F _______________ R 2. The resistance moves a longer distance, and A 3. The force is applied through a short distance. If the axis is close to the resistance, the RA will be shorter This is an example of a first-class lever, because the and the FA will be longer. Therefore, it will be easy to axis is in the middle, with the force on one side and resist- move the resistance. If the axis is close to the force, just the ance on the other. By placing the axis close to the resist- opposite will occur; it will be hard to move the resistance. ance, you have a lever that favors force. By placing the axis Try this with a pencil (axis), a ruler (force), and a fairly close to the force, you have a lever that favors distance heavy book (resistance). Something small that doesn’t (range of motion) and speed. If you place the axis midway roll easily would make a better axis; otherwise, have between the force and the resistance (assuming they are someone hold the pencil in place. The ruler—or even the same weight), the lever favors balance. another long pencil—can be used, but it must be a rigid Figure 8-23 shows a worker carrying two bundles of bar. Place the ruler about 2 inches under the book so it hay. Each bundle (one is force and the other is resistance) will stay under the book as the book is raised. Place the pencil perpendicularly under the ruler near the book (Fig. 8-22A). You have created a long FA and a short RA. Push down on the outer end of the ruler and notice two things: (1) how easy it is to raise the book, and (2) how far down you have to push the ruler. Next, move the pencil (axis) out toward the other end of the ruler, and push down on the ruler (Fig. 8-22B). This time you should notice that it is harder to raise the book, but you didn’t Resistance arm Force arm F R Resistance Axis Force Figure 8-23. First-class lever. The two loads (F and R) are Figure 8-21. Components of a lever. balanced on the shoulders. 2363_Ch08-093-112.qxd 12/9/10 5:35 PM Page 104 104 PART I Basic Clinical Kinesiology and Anatomy (force) must contract to pull the weight of your head up A against gravity (resistance). If you look up to the sky, your head would rock back and you would use your anterior neck muscles to pull your head into the upright position (Fig. 8-24B). Although force and resistance may A change places, depending on the motion, the axis is R always in the middle of a first-class lever. F In a second-class lever, the resistance is in the middle, with the axis at one end and the force at the other end: R F Second-class lever _______________________ A The wheelbarrow is an example of a second-class lever (Fig. 8-25). The wheel at the front end is the axis, the wheelbarrow contents are the resistance, and the person B pushing the wheelbarrow is the force. If we assume that the wheelbarrow is carrying a load of heavy bricks, we can apply the earlier statement that the longer the FA, the easier it is to move the part and the longer the RA, the harder it is to move the part. If we put all the bricks as close to the R A F Resistance arm Figure 8-24. Head moving on neck demonstrates a first- class lever. In (A), the axis is the head posteriorly moving on the vertebral column and is located between force (the extensor muscles), and resistance (weight of the head itself). In (B), the axis is the head moving anteriorly on the vertebral column and is located between the force (flexor muscles) and A Force Axis the resistance (weight of head). is approximately the same weight and distance from the axis. The shoulder is the axis. If one bundle is heavier, it would have to be moved closer to the axis to keep the overall load balanced. An example of a first-class lever in the human body is Resistance arm the head sitting on the first cervical vertebra, moving up and down in cervical flexion and hyperextension. The vertebra is the axis, the resistance is the weight on one side of the head, and the force is the muscle pulling down on the opposite side of the head. The force and resistance will change places, depending on which way the head is B tipped. For example, as shown in Figure 8-24A, if your Force Axis head is tipped toward your chest and you want to return Figure 8-25. Second-class lever. (A) RA is shorter. to the upright position, your posterior neck muscles (B) RA is longer. 2363_Ch08-093-112.qxd 12/9/10 5:35 PM Page 105 CHAPTER 8 Basic Biomechanics 105 wheel as possible (Fig. 8-25A), we now have a long FA (Fig. 8-27). The axis is the front of the boat tied to the and a short RA. The wheelbarrow should be fairly easy to dock. The force is the person pushing on the boat, and move. However, if we move the bricks to the other end of the resistance is the weight of the boat. If the person push- the wheelbarrow (Fig. 8-25B), the FA remains the same es close to the front of the boat as in Fig. 8-27A, it will be length, but the RA is longer. The wheelbarrow is now harder to move the boat but the back of the boat will harder to move because we have lengthened the RA. swing farther away from the dock. Conversely, if the per- There are relatively few examples of second-class son pushes farther back on the boat as in Fig. 8-27B, the levers in the body; however, the action of the ankle plan- boat stern won’t swing away from the dock as far, but it tar flexor muscles when a person stands on tiptoe is one will be easier to move. In this case, the RA doesn’t change, (Fig. 8-26). In this case, the axis is the metatarsopha- but the FA does. When the FA is shorter, the boat is hard langeal (MTP) joints in the foot, the resistance is the to push but moves a greater distance. When the FA is tibia and the rest of the body weight above it, and the lengthened, the boat is easier to push but doesn’t move as force is provided by the ankle plantar flexors. Therefore, far. In other words, any gain in distance is lost in power. the resistance (body weight) is between the axis (MTP joint) and the force (plantar flexors). The RA is only slightly shorter than the FA. This lever favors power because a relatively small force (the muscle) can move a large resistance (the body). However, the body can be A raised only a fairly short distance. This again proves the basic rule of simple machines—what is gained in power (raising the body weight) is lost in distance (the body can’t be raised very far). F R Force A A Resistance Axis F R Figure 8-26. Plantar flexors lifting body weight demonstrates a second-class lever. A third-class lever has force in the middle, with resistance and the axis at the opposite ends: B Figure 8-27. Moving boat tied to dock demonstrates a F R Third-class lever ___________________ third-class lever (axis, force, resistance). A is the point where A the boat is held against the dock. F is where the person pushes (or pulls) the boat away (toward) the dock, and An example of this type of lever is a person moving R is the weight of the boat. In (A), it will be easier to move one end of a boat either toward or away from a dock the boat, while in (B) it will be harder. 2363_Ch08-093-112.qxd 12/9/10 5:35 PM Page 106 106 PART I Basic Clinical Kinesiology and Anatomy The advantage of the third-class lever is speed and Why are there so many third-class levers (which favor distance. This is, by far, the most common lever in the speed and distance) and so few second-class levers (which body. In the example of elbow flexion (Fig. 8-28), the favor power) in the body? Probably because the advantage axis is the elbow joint, the biceps muscle exerts the gained from increased speed and distance is more impor- force, and the resistance is the weight of the forearm tant than the advantage gained from increased power. and hand. For the hand to be truly functional, it must Examine the roles of the biceps and the brachioradialis be able to move through a wide range of motion. The muscles in elbow flexion (Fig. 8-29). They both cross the resistance, in this case, will vary depending on what, if elbow but attach to the radius at very different places. The anything, is in the hand. biceps muscle attaches to the proximal end of the radius, while the brachioradialis muscle attaches to the distal end. The biceps muscle acts as the force in a third-class lever because it attaches between the axis (elbow) and the resistance (COG of forearm/hand; Fig. 8-29A). The bra- chioradialis muscle is the force in a second-class lever (Fig. 8-29B) because it attaches at the end of the forearm, putting the resistance (COG of forearm/hand) in the Force middle. For example, say that each muscle is capable of contracting approximately 4 inches. Remember that a muscle can shorten to half of its resting length. Therefore, the brachioradialis muscle will be able to move the distal Axis Resistance end of the forearm and, subsequently, the hand approxi- mately 4 inches, because its attachment is near the Figure 8-28. The biceps demonstrating a third-class lever. distal end. The biceps muscle, with its attachment at the Axis Force Resistance Muscle uses more force A to move joint farther Axis Resistance Force Muscle needs less force but B moves joint shorter distance Figure 8-29. Third-class levers favor distance (A), and second-class levers favor force (B). 2363_Ch08-093-112.qxd 12/9/10 5:35 PM Page 107 CHAPTER 8 Basic Biomechanics 107 proximal end, will move the proximal end of the forearm if you put a weight in the hand, the COG of the resistance approximately 4 inches, which will move the hand at the is now located farther from the axis than the force (mus- distal end much farther, say 12 inches. Because the main cle), as depicted in Figure 8-30B. Therefore, the brachio- function of the upper extremity is to allow the hand to radialis is now working as a third-class lever. move through a wide range, it makes sense that most The direction of the movement in relation to gravity muscles act as third-class levers, favoring range of motion. is another factor that will affect lever class. For example, the biceps illustrated in Figure 8-31A is a third-class Factors That Change Class lever because it contracts concentrically to flex the Under certain conditions, a muscle may change from a elbow. The muscle is the force and the forearm is the second-class (axis-resistance-force) to a third-class lever resistance. The force is between the axis and the resist- (axis-force-resistance), and vice versa. For example, the ance; therefore, it is a third-class lever. If you put a brachioradialis has been described as a second-class weight in the hand, it will still be a third-class lever. lever, with the weight of the forearm and hand being However, if the muscle contracted eccentrically, it will the resistance. Using the middle of the forearm as its become a second-class lever. What has changed? As the COG, the weight of the forearm and hand (R) is locat- elbow extends, moving the same direction as the pull of ed between the axis (elbow joint) and the force (distal gravity, the biceps must contract eccentrically to slow muscle attachment), as shown in Figure 8-30A. However, the pull of gravity. Gravity and its pull on the forearm becomes the force. The biceps becomes the resistance slowing elbow extension (Fig. 8-31B). With the resist- ance now in the middle between the force and the axis, the biceps becomes a second-class lever. There are many applications of leverage in rehabilita- tion. The importance of levers can be seen in such things as saving energy or making tasks possible when Force Axis Force A Resistance Resistance A Axis Resistance Force Axis Axis Force B B Resistance Figure 8-30. (A) The brachioradialis as a second-class Figure 8-31. The biceps acts as a third-class lever when lever. (B) It becomes a third-class lever when a weight is contracting concentrically (A), and a second-class lever placed in the hand. when contracting eccentrically (B). 2363_Ch08-093-112.qxd 12/9/10 5:35 PM Page 108 108 PART I Basic Clinical Kinesiology and Anatomy strength is limited. To summarize, less force is required if you put the resistance as close to the axis as possible and apply the force as far from the axis as possible. Pulleys Peroneus longus A pulley consists of a grooved wheel that turns on an axle with a rope or cable riding in the groove. Its purpose is to either change the direction of a force or to increase or decrease its magnitude. A fixed pulley is a simple pul- ley attached to a beam. It acts as a first-class lever with F on one side of the pulley (axis) and R on the other. It is Force used only to change direction. Clinical examples of this Lateral malleolus can be found in overhead and wall pulleys (Fig. 8-32) Axis and in home cervical traction units. In the body, the lat- eral malleolus of the fibula acts as a pulley for the ten- Resistance don of the peroneus longus and changes its direction of Figure 8-33. The lateral malleolus acts as a pulley, allowing pull (Fig. 8-33). Another example of a pulley is a Velcro the peroneus longus to change its direction of pull. strap on a shoe. The strap passes through a slot and folds over on itself. A movable pulley has one end of the rope attached supported by both segments of the rope on either side of to a beam; the rope runs through the pulley to the other the pulley so it has a mechanical advantage of 2. It will end where the force is applied. The load (resistance) is require only half as much force to lift the load because suspended from the movable pulley (Fig. 8-34). The pur- the amount of force gained has doubled. Although only pose of this type of pulley is to increase the mechanical half of the force is needed to lift the load, the rope must advantage of force. Mechanical advantage is the num- be pulled twice as far. In other words, it is easier to pull ber of times a machine multiplies the force. The load is the rope, but the rope must be pulled a much farther dis- tance. The human body has no examples of a movable pulley. Fixed pulley Axis Force Movable pulley Resistance Rope must be One half the pulled twice as force is needed far to move the weight (resistance) Figure 8-34. A movable pulley has a mechanical advantage Figure 8-32. Fixed pulley. Its purpose is to change direction. for force. 2363_Ch08-093-112.qxd 12/9/10 5:35 PM Page 109 CHAPTER 8 Basic Biomechanics 109 Wheel and Axle The wheel and axle is another type of simple machine. It is actually a lever in disguise. The wheel and axle consists of a wheel, or crank, attached to and turning together with an axle. In other words, it is a large wheel connected to a smaller wheel and typically is used to increase the force exerted. Turning around a larger wheel or handle requires less force, whereas turning around a smaller axle requires a greater force. An example of a wheel and axle is a faucet handle (Fig. 8-35). The handle is the wheel and Wheel radius = 2 inches the stem is the axle. Turning the faucet requires a certain amount of force made easier by a longer force arm (wheel radius; Fig. 8-36A). However, take off the handle and you are left with only the axle (Fig. 8-36B). Try turning it and A you will realize that a great deal more strength is needed to do so. Simply stated, the larger the wheel (handle) in relation to the axle, the easier it is to turn the object. Just like the lever—in which the longer the FA, the greater the force—the wheel and axle provides greater force with a larger wheel. Assume that you are treating a person who has severe arthritis in the hands and is unable to turn faucet han- dles easily. If you replace the handle (Fig. 8-37A) with a long, lever-type handle (Fig. 8-37B), you still have a wheel and axle. Visualize the handle as one spoke of the Axle radius = 1Ⲑ8 inch wheel with the rest of the spokes missing. The longer faucet handle is easier to turn (force advantage), but the B handle must be turned a greater distance. Figure 8-36. The wheel of the faucet handle (A) has a longer radius than the axle (B). Therefore, the larger wheel is To give an example of a wheel and axle in the human easier to turn than the smaller axle. body, think of performing passive shoulder rotation on a patient. It can best be visualized by looking down on the shoulder from a superior view (Fig. 8-38). The shoulder joint serves as the axle, and the forearm serves as the wheel. With the elbow flexed, the wheel is much Wheel longer than the axle and thus much easier to turn. Inclined Plane Axle Although there are no examples of an inclined plane in the human body, the concept of wheelchair accessibility Radius Radius A B Figure 8-37. Typical faucet handles. Note that (A) has Figure 8-35. A faucet handle demonstrates a wheel a shorter radius and requires more force to turn the and axle. wheel than (B). 2363_Ch08-093-112.qxd 12/9/10 5:35 PM Page 110 110 PART I Basic Clinical Kinesiology and Anatomy and the ramp is 24 feet long, it would be fairly easy to propel the wheelchair up this long ramp (Fig. 8-39A). If the ramp is only 12 feet long, it would be much steeper. The person would not have to propel the wheelchair as far but would have to use more force to do so (Fig. 8-39B). Repeating the basic rule of simple ed machines: the advantage gained in force (decreased Flex rm = a effort needed) is lost in distance (longer ramp needed). fore eel Wh Points to Remember The effect of forces can be linear, parallel, or concurrent. A force couple occurs when forces act together but in opposite directions to provide the same motion. A scalar quantity describes magnitude, whereas vector also includes direction. Shoulder joint = Axle Forces can be stabilizing, angular, or dislocating. Figure 8-38. The upper extremity acting as a wheel and axle. Gravity has an effect on all objects, and its force is always downward. Stability is affected by an object’s COG often depends on this type of simple machine. An and BOS. inclined plane is a flat surface that slants. It exchanges The three classes of levers have different increased distance for less effort. The longer the length purposes and mechanical advantages, of a wheelchair ramp, the greater the distance the wheel- depending on the relationship of the axis, chair must travel; however, it requires less effort to pro- the force, and the resistance. pel the chair up the ramp, because the ramp’s incline is Changing the length of the FA or RA will less. For example, if a porch is 2 feet from the ground make the part easier or harder to move. Fixed pulleys in the human body change the direction of a muscle’s force. The wheel and axle, much like the lever, can A longer ramp increase the force. Inclined planes can exchange increased dis- requires less force tance for decreased effort. A A shorter ramp requires more force B Figure 8-39. Inclined plane as a wheelchair ramp. A longer ramp (A) requires less force but greater distance to reach a certain height. A shorter ramp (B) requires more force but less distance to reach same height. 2363_Ch08-093-112.qxd 12/9/10 5:35 PM Page 111 CHAPTER 8 Basic Biomechanics 111 Review Questions 1. Putting a weight cuff in which position would require more effort at the shoulder joint to move the weight cuff through shoulder range of motion? Explain your answer. a. Cuff positioned at the wrist b. Cuff positioned at the elbow 2. Two people have the same weight and BOS, but one is on stilts. Which person is more 8. In terms of BOS, why is it more difficult for a per- stable? Why? son in a wheelchair to balance on only the back a. The person on stilts wheels (“wheelie”) rather than on all four wheels? b. The person not on stilts 9. Two people are standing on the same side of a 3. What is the resultant force of the following muscles? patient’s bed. They plan to move the patient a. Two heads of the gastrocnemius toward them by pulling on the draw sheet. This move would be what type of force: linear, parallel, concurrent, or force couple? 10. Prior to moving the patient, what can the people do to increase their own stability? b. Sternal and clavicular portions of the pectoralis 11. When cracking an almond with a nutcracker, will major the almond be easier to crack if it is closer to the axis or closer to the end of the handles? Why? 12. Does the figure below represent forces that are linear, parallel, or concurrent? Why? 4. You are given two different sets of instructions. The first instruction tells you to run 5 miles, and the second instruction tells you to walk 30 feet to the north. Circle the correct answer. a. Running 5 miles is a vector/scalar quantity. b. Walking 30 feet to the north is a vector/scalar quantity. 5. A delivery person has several boxes stacked on a hand truck. Would the person have to use more force to push the hand truck when the hand truck is more horizontal or more vertical? Why? 6. Compare the push rims of a standard wheelchair and a racing wheelchair. Note that the racing 13. Give an example of bony structures at the knee act- wheelchair has much smaller push rims. What ing as a pulley to increase the angle of pull. is the advantage of smaller push rims to a wheel 14. Explain why a person leans to the right when carry- chair racer? ing a heavy suitcase in the left hand. If the suitcase 7. Label the BOS, COG, and LOG for the object was very heavy, what might the person do with her shown here. The object is of uniform density right arm? Why? throughout its shape. Can this object remain 15. Why are rubber tips put on the ends of crutches? upright without support? Why?