lipids.pdf
Document Details
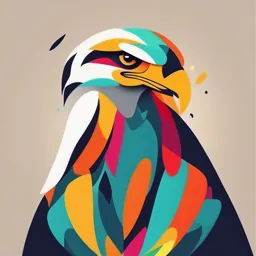
Uploaded by ResoluteMatrix
Tags
Full Transcript
CHAPTER 11 Hyperlipidemia and Dyslipidemia Paul G. Davis, PhD Peter W. Grandjean, PhD Stephen F. Crouse, PhD J. Larry Durstine, PhD L ipids are fats or derivatives of fat that are insoluble in water and must be bound to protein to be transported in blood. Cholesterol is a lipid that is an essential...
CHAPTER 11 Hyperlipidemia and Dyslipidemia Paul G. Davis, PhD Peter W. Grandjean, PhD Stephen F. Crouse, PhD J. Larry Durstine, PhD L ipids are fats or derivatives of fat that are insoluble in water and must be bound to protein to be transported in blood. Cholesterol is a lipid that is an essential component of cell membranes and a number of vital substances, including bile acids, steroid hormones, and vitamin D. High plasma cholesterol concentrations present a major public health problem, given that 38% of U.S. adults have a concentration ≥200 mg/dL and this substantially increases a person’s risk for atherosclerotic cardiovascular disease (ASCVD) (200). Triglycerides are other important blood lipids; they are major carriers of energy-containing fatty acids. A high triglyceride concentration can increase one’s risk of vascular and other diseases. ASCVD risk is also affected by the concentrations of different types of lipoproteins (lipid–protein complexes that transport cholesterol and triglycerides in the blood). Lipid and lipoprotein concentrations are majorly affected by genetics, diet, and, to a lesser extent, physical activity. Because of the high prevalence of elevated plasma cholesterol and triglycerides, professionals providing exercise testing and training, as well as patients themselves, should be keenly aware of ASCVD signs and symptoms, as well as the side effects of medications used to treat abnormal lipid concentrations. DEFINITION The terms hypercholesterolemia and hypertriglyceridemia represent unhealthily elevated plasma concentrations of cholesterol and triglycerides, respectively. Hyperlipidemia is a collective term that can represent either or both of the above. Although often used interchangeably with hyperlipidemia, the term dyslipidemia refers to abnormal lipid concentrations that may be high, but it can also refer to low levels of cholesterol associated with lipoproteins that are mostly cardioprotective in nature. Similar to dyslipidemia, dyslipoproteinemia describes the condition of abnormal lipoprotein levels. The most severe forms of dyslipidemia and dyslipoproteinemia are linked to genetic defects in cholesterol or triglyceride metabolism (26, 50, 172). Secondary dyslipidemia usually results from metabolically dysfunctional conditions that arise from the accumulation of ectopic fat (199), insulin resistance (80), diabetes mellitus (61, 146, 193), hypothyroidism (168), and renal insufficiency and nephrotic kidney disease (85, 90), some of which can be due to a high-fat or hypercaloric diet. Since lipids are not soluble in body fluids, they must combine with proteins to be transported in blood and other fluids in the body. Lipoproteins are spherical macromolecules composed of an outer shell of hydrophilic apolipoproteins and phospholipids, with the more hydrophobic triglycerides and cholesterol packaged in the core. In addition to providing structural integrity, apolipoproteins possess functional roles, such as activating or inhibiting enzymes and serving as ligands for receptors (table 11.1) (94). Scientists and clinicians use a variety of techniques to classify and study lipoproteins (77). In fact, the measurement technique dictates how lipoproteins are identified and described. The clinician should remember that the lipid and apolipoprotein composi- Acknowledgment: We wish to gratefully acknowledge and thank Benjamin Gordon, PhD, ACSM-CEP, for his previous work on this chapter. 167 168 Davis, Grandjean, Crouse, and Durstine Table 11.1 Major Human Apolipoproteins Apolipoprotein Major function ASCVD risk factor A-I LCAT activator; SR-BI ligand Inversely related with ASCVD risk A-II LCAT inhibitor or activator of heparin releasable hepatic triglyceride hydrolase or both; SR-BI ligand Not associated with ASCVD risk B-48 Required for synthesis of chylomicron Directly associated with ASCVD risk B-100 LDL receptor–binding ligand Directly associated with ASCVD risk (a) Similar characteristics between apo(a) and plasminogen, thus may have a prothrombotic role by interfering with function of plasminogen; possible acute phase reactant to tissue damage Directly associated with ASCVD risk C-I LCAT activator Not associated with ASCVD risk C-II LPL activator Not associated with ASCVD risk C-III LPL inhibitor, several forms depending on content of sialic acids Not associated with ASCVD risk D Core lipid transfer protein, possibly identical to the cholesteryl ester transfer protein Not associated with ASCVD risk E Remnant receptor–binding ligand, present in excess in the β-VLDL of patients with type III hyperlipoproteinemia and exclusively with HDL cholesterol Not associated with ASCVD risk ASCVD = atherosclerotic coronary artery disease; LCAT = lecithin-cholesterol acyltransferase; SR-BI = scavenger receptor class B type I; LDL = low-density lipoprotein; LPL = lipoprotein lipase; VLDL = very low-density lipoprotein; HDL = high-density lipoprotein. tion constantly changes in the circulation as these macromolecules interact with each other and with the surrounding body tissues. Clinicians generally discuss blood lipids and lipoproteins based on a traditional measurement technique called ultracentrifugation. To be consistent, we’ll describe general classes of lipoproteins based on gravitational density determined by ultracentrifugation methodology (table 11.2) (77). Development of arterial atherosclerotic lesions, characterized first by fatty streaks and later by fibrous plaque, is significantly related to concentrations of total blood cholesterol, low-density lipoprotein cholesterol (LDLc), and triglycerides and inversely with levels of high-density lipoprotein cholesterol (HDLc). As described in the Pathophysiology section, LDL is primarily responsible for the delivery of cholesterol to tissues throughout the body, including atherosclerotic plaque. HDL, on the other hand, has been shown to harvest cholesterol from plaque and deliver it to the liver. Chylomicrons and very low-density lipoproteins (VLDLs) are the major carriers of triglyceride although, unless triglyceride concentration is unusually high, chylomicrons are present only in trace amounts in fasted plasma. SCOPE A wealth of data supports the relationship between dyslipidemias and greater risk for ASCVD and other conditions (e.g., cerebrovascular disease, kidney disease). Arterial atherosclerotic lesions begin in childhood and increase in prevalence and size with age. As such, relationships between blood lipid concentrations and ASCVD morbidity and mortality have been regularly demonstrated across the life span (20, 149, 171). A meta-analysis of 49 randomized controlled trials of various lipid-lowering medications and supplements demonstrated a 20% reduction of major ASCVD events (myocardial infarction, coronary revascularization, or sudden cardiac death) per 1 mmol/L (39 mg/dL) decrease in LDL-c and a 16% reduction of events per 1 mmol/L (89 mg/dL) decrease in triglycerides. In an earlier meta-analysis, Genser and Marz (72) estimated that LDLc lowering in adults accounted for 75% of the variance in risk reduction for cardiovascular end points. Randomized controlled trials of various methods to lower nonHDLc (i.e., atherogenic cholesterol, LDLc + VLDLc) consistently demonstrate that achieving lower on-treatment levels is associated with lower absolute risk of ASCVD events (21, 140). Although low HDLc concentrations (240 mg/dL. LDLc and non-HDLc are the primary targets for lipid-lowering interventions (84, 105, 188). Approximately 69.6 million adults, or 29% of the U.S. adult population, have LDLc >130 mg/dL (200). Elevated LDLc is present in 29% of males and 30% of females, with Hispanic and NH Asian males having slightly higher averages (34% and 32%, respectively) and NH Black, Hispanic, and NH Asian females slightly lower (23%, 24%, and 25%, respectively). Low serum HDLc, generally defined as 150 mg/dL) are observed in approximately 22% of the U.S. adult population and tend to be higher than average in Hispanic, NH Asian, and NH white males (200). The percentage of U.S. adults aged 20 to 74 yr with hypercholesterolemia has decreased from 33% to 12% since 1960. Tracking over the same time, the population-wide average total cholesterol has decreased from 222 mg/dL to 191 mg/dL (188 mg/dL in males, 193 mg/dL in females) (32, 169, 179, 200). This reduction in individuals with hypercholesterolemia represents a Healthy People 2010 objective that was attained, although the Healthy People 2020 target of lowering the nation’s average cholesterol concentration to 177.9 mg/dL has not been attained at the time of this writing (200). Nevertheless, these population statistics are promising from a public health perspective, since the American Heart Association (AHA) estimates that the incidence of ASCVD can be reduced by 30% for every 10% decrease in total cholesterol among U.S. adults (169). Average LDLc values have also declined, from 129 to 112 mg/ dL. Much of the reduction in our population-wide total cholesterol and LDLc values has been attributed to dietary changes (30) and the increased use of lipid-lowering drugs (102, 104, 123). Indeed, the use of lipid-lowering medication has increased dramatically over the last few decades, and these drugs have had a greater prescription volume than any other drug class over this century. Statins are recommended by the major cardiology associations both before (primary prevention) and after (secondary prevention) cardiovascular events (73, 84, 97). As such, statins are by far the most frequently prescribed of the lipid-lowering agents, and approximately 75% of individuals taking statins do so for primary prevention (104, 123). Although the trends for recommended concentrations of total cholesterol and LDLc are promising, continued and more aggressive lipid-lowering interventions appear to be warranted. Of U.S. adults who have a 10 yr predicted ASCVD diagnosis risk of at least 7.5%, only about one-third are taking a statin, which is recommended as the first-line medication for hyperlipidemia. Self-reported statin use is only 66%, 65%, and 46% for individuals with LDLc ≥190 mg/dL, ASCVD, and diabetes, respectively (200). This problem is greater among younger adults compared with those aged 65 yr and older (102). Issues associated with reduced compliance in taking statins include occasional muscle pain and, since hyperlipidemia itself generally does not present symptoms, lack of “feeling better” after starting the medication. Moreover, the average HDLc and triglyceride values have not changed appreciably over the last five decades (32). This result is likely due to the increased incidence of overweight and obesity among our adult population over the same period (32, 62). In fact, Burke and colleagues (28) reported that fasting glucose and triglyceride concentrations were greater (8 to 17 mg/dL and 18 to 55 mg/dL), HDLc was lower (4 to 14 mg/dL), and concentrations of small LDL particles were greater (109 to 173 mmol/L) in obese adults versus individuals of normal weight across ethnicities. PATHOPHYSIOLOGY Atherosclerotic disease processes originate in early childhood and continue to develop through the interactions of genes with modifiable and nonmodifiable environmental exposures. These exposures influence a complex network of lipid and lipoprotein pathways that provide transportation for delivery, metabolism, or elimination. Chapter 11 Hyperlipidemia and Dyslipidemia Lipoprotein Metabolic Pathways are internalized into the core of the chylomicron and enter the circulatory system via the lymphatic system and the thoracic duct. Blood chylomicrons then react with LPL (apo C-II facilitates this reaction), hydrolyzing the triglyceride core and releasing fatty acids. During this process, some of the phospholipids and apolipoproteins are transferred to nascent HDL particles, while other apolipoproteins of the chylomicron remnants bind to apo E and apo B-48 receptors on the surface of hepatic (liver) cells, allowing these remnants to be removed from circulation and catabolized. A similar endogenous pathway exists for VLDLs synthesized by the liver. In this pathway, LPL breaks down the VLDL triglyceride core, releasing fatty acids that are taken up by extrahepatic tissue. The remnant intermediate-density particles interact with LPL and HL to form LDL, with the remaining VLDL remnants being removed from the blood by hepatic apo E receptors. Low-density lipoprotein particles formed in this process are the primary cholesterol carriers and deliver LDLc to extrahepatic tissue, where LDL receptors located on the cell’s surface mediate its uptake. Once the LDLc is recognized by the LDL-apo B-100/ apo E receptor, LDL is moved inside the cell and used for cellular metabolic needs. This process also initiates a negative feedback response within the cell, causing a reduction in cellular cholesterol synthesis while promoting cholesterol storage. As these processes occur, cellular LDL receptor synthesis is suppressed and further cellular LDL uptake is halted. Blood lipoproteins provide a system of transportation for the movement of lipids between the intestine, liver, and extrahepatic tissue (figure 11.1). Several reviews deal with the complex interactions that take place within the circulation (45, 71, 74, 132, 183). Important enzymes and transfer proteins facilitate these interactions: lipoprotein lipase (LPL), hepatic lipase (HL), lecithin–cholesterol acyltransferase (LCAT), and cholesteryl ester transfer protein (CETP). Although scientists and clinicians recognize the dynamic exchange of lipids and lipoproteins among the various lipoprotein classes and their subfractions, the transport of cholesterol and triglycerides is generally described in terms of two general processes (26, 45, 50, 198). The LDL receptor pathway consists of a sequence of chemical steps designed for the delivery of lipids to extrahepatic tissue (45). A second pathway, reverse cholesterol transport, involves a sequence of chemical reactions necessary for returning lipids from peripheral tissue to the liver for metabolism and excretion (53, 198). These two intravascular transport processes are described next in further detail. LDL Receptor Pathway Dietary, or exogenous, fat is absorbed by the small intestine as fatty acids and free cholesterol. During absorption, these lipids combine with apolipoproteins B-48, A-I, A-II, A-IV, and E and Liver Ileum CM receptor (Apo E) C and Tg pool HDL3 CE Tg 11 10 LDL receptor (Apo B-100,E) HDL3 6 4 CE Tg 6 LDL CE Tg VLDL CMr FC IDL FC LDL receptor (Apo B-100,E) CE Tg 9 5 CM FC FC CE Tg 7 CE Tg 1 C 171 3 CE Tg FC 2 LPL 8 LPL C CE Tg Extrahepatic tissues Figure 11.1 Transport of lipids between the intestine, liver, and extrahepatic tissues. See the section LDL Receptor Pathway for details. Apo = apolipoprotein; C = cholesterol; CE =E8177/Ehrman/Fig. cholesteryl ester; FC = free cholesterol; TG = triglyceride; CM = chylomicron; 11.01/680788/HR/R1 CMr = chylomicron remnant; HDL = high-density lipoprotein; IDL = intermediate-density lipoprotein; LDL = low-density lipoprotein; VLDL = very low-density lipoprotein; LPL = lipoprotein lipase. 172 Davis, Grandjean, Crouse, and Durstine Reverse Cholesterol Transport macrophages and vascular endothelial cells), has high affinity for HDL-associated apo A-I and apo A-II, and allows bidirectional flux of cholesterol between cells and HDL. As such, SR-BI, which contains a lipophilic tunnel-like structure, allows HDL to both “harvest” cholesterol from atherosclerotic lesions and “unload” it into the liver and steroid hormone–producing tissues. Unlike some of the other hepatic lipoprotein receptors, SR-BI takes cholesterol into the liver while preserving the spherical structure of HDL, allowing it to remain in circulation to participate further in reverse cholesterol transport. As mentioned in the Scope section of this chapter, although HDL can participate in reverse cholesterol transport, raising HDLc concentration pharmaceutically does not typically decrease ASCVD risk, and this risk may be paradoxically elevated in people with abnormally high HDLc levels. Hence, HDLc concentration does not tell the whole story. For example, optimal reverse cholesterol transport relies on proper structure and function of both the HDL-associated apolipoproteins and their receptors. Malfunction of either could cause more cholesterol to be trapped within HDL, with the unloading of cholesterol from HDL to the liver being disrupted (175). In fact, a rare SR-B1 mutation has been associated with higher HDLc and is more likely to be present in people with a history of ACSVD (217). In addition to its role in cholesterol efflux from atheroma and transport to the liver, HDL may possess antioxidative, anti-inflammatory, and antithrombotic properties and may also contribute to vascular endothelial repair. Cholesterol is moved to the liver for catabolism by a process termed reverse cholesterol transport (figure 11.2). This elimination of cholesterol from the peripheral tissue involves several processes. The most notable pathway uses nascent HDL particles secreted by the liver and enriched with free cholesterol and phospholipid derived from LPL-mediated chylomicron and VLDL catabolism. In this process, free cholesterol is esterified by the action of LCAT, with apo A-I as a cofactor. The cholesteryl ester is moved into the HDL3 core, causing a chemical gradient with a constant cholesterol supply for the LCAT reaction. As the HDL 3 core expands, the HDL3 is transformed into a more lipid-rich HDL2 particle. While this process is happening, reverse cholesterol transport proceeds in both indirect and direct pathways. In an indirect pathway, CETP facilitates an exchange for the newly formed HDL2 cholesteryl ester with triglyceride obtained from triglyceride-rich lipoprotein (TRL) remnants. The remaining lipid-depleted TRL remnants are transported to the liver for metabolism and removal from circulation. A second set of reactions mediated by hepatic HL removes triglyceride from the HDL2 particle that previously gained triglyceride by CETP action. Smaller, denser HDL3 particles return to the circulation once HL-mediated triglyceride removal is complete. In direct pathways, HDLc (especially from cholesteryl ester– rich HDL2 particles, because of the content and morphology of their apolipoproteins) is withdrawn from circulation through various hepatic receptors. One important receptor in this process is the scavenger receptor class B type I (SR-BI). The SR-BI exists on both hepatic and extrahepatic cells (including atherosclerotic Liver CE CE Tg ApoE receptor Lipoprotein catabolism products SR-BI (Apo A-i, A-II) Nascent HDL CE LCAT HDL3 HDL2 CE Tg LCAT Tg CE Tg CE CETP TRLs and remnants CE To extrahepatic tissues Extrahepatic tissues Figure 11.2 Reverse cholesterol transport. See text for details. Apo = apolipoprotein; CE = cholesteryl ester; TG = triglyceride; HDL = high-density lipoprotein; TRL = triglyceride-rich lipoprotein; LCAT = lecithin–cholesterol acyltransferase; CETP = cholesteryl ester transfer protein; SR-BI = scavenger receptor class B type I. E8177/Ehrman/Fig. 11.02/680789/HR/R2 Chapter 11 Hyperlipidemia and Dyslipidemia Postprandial Lipemia Normally, blood triglycerides will not appreciably increase or will quickly and modestly rise after a meal and then steadily decline (121, 122). Generally, the time needed after a meal for blood triglyceride levels to return to fasting levels is 6 to 8 h. Exaggerated or prolonged lipemia is associated with increased ASCVD risk (121, 126). Elevated postprandial lipemia (PPL) may initiate harmful events associated with endothelial dysfunction and arterial plaque buildup (47, 153). Possibly the most significant finding is that PPL fosters the formation of the highly atherosclerotic small, dense LDL particles while reducing the concentration of HDLc. Prolonged PPL also promotes inflammation, oxidative stress, and thrombosis (blood clot) formation (204). Genetic factors affect the magnitude of the postprandial responses (156), but postprandial lipid responses are also affected by exercise (142, 158) and nutrient composition (128, 153). Metabolic Dyslipidemia The metabolic syndrome (see chapter 12) is a well-recognized but contentious concept in the scientific literature and in clinical practice (70, 166, 187). There are ongoing large-scale efforts to understand the complex pathophysiology that arises from the ectopic fat accumulation, insulin resistance, and dysfunction in glucose and lipid metabolism that result (55, 167, 199). An accompanying complication is hypertriglyceridemic dyslipidemia, which occurs mostly because of overproduction of TRLs. In recent years scientists have focused on the mechanisms regulating the overproduction of these atherogenic apo B–containing lipoproteins, and evidence from animal models and human studies has identified hepatic VLDL overproduction as a critical underlying factor in the development of hypertriglyceridemia and metabolic dyslipidemia (which is characterized by the combination of high triglyceride and low HDLc concentrations) (12, 13). Metabolic dyslipidemia is a common component of metabolic syndrome and was associated with a 48% higher risk of ASCVD events in overweight or obese adults with type 2 diabetes mellitus in the Look AHEAD (Action for Health in Diabetes) study (110). Elevation of bloodborne fatty acids observed with metabolic dyslipidemia affects the vascular endothelium by reducing nitric oxide production, inducing adhesion characteristics, facilitating oxidative damage and inflammation, and resulting in diminished vascular compliance and reactivity (34, 204). Oversupply of these fatty acids to the liver facilitates triglyceride synthesis, reduces apolipoprotein B-100 degradation, and enhances the production of triglyceride-rich VLDL. Elevated plasma triglyceride concentrations and TRLs can be further exacerbated by impaired LPL activity (145). The increased actions of HL and CETP, observed with insulin resistance and an overabundant amount of liver fat, potentiate the transfer of triglyceride from VLDL to HDL and result in the formation of small, dense LDL. These smaller, dense LDL are 173 less likely to be taken up by the normal LDL receptor pathway, more readily penetrate the vascular endothelium, and are more susceptible to oxidative damage (12). Once in the subendothelial tissue, the modified LDL induces vascular inf lammation and contributes to atherosclerotic plaque accumulation and lesion instability (127). At the same time, the activities of HL and CETP and dysfunctional apolipoprotein metabolism contribute to greater hepatic HDL clearance and a reduction in HDLc and HDL particle numbers (34). Effects on HDL number and composition further impair reverse cholesterol transport and attenuate HDL antioxidant potential. CLINICAL CONSIDERATIONS Elevated LDLc proportionally increases one’s risk for ASCVD (112). Although national guidelines for treating dyslipidemias have existed since the early 1980s and some improvement has been seen, many individuals at the turn of the century were still not achieving previously recommended goals or residual ASCVD risk remained despite LDLc lowering (161). Accordingly, in 2002, the Adult Treatment Panel III (ATP III) of the National Institutes of Health’s National Cholesterol Education Program (161) called for aggressive clinical management of dyslipidemias and emphasized achievement of targeted lipid-lowering goals based on ASCVD risk. These recommendations have been updated by various organizations over the years, based on evolving treatments and knowledge, culminating in consensus guidelines (2018) that tailor treatment based on an individual’s lipid and lipoprotein profile combined with their risk for or presence of ASCVD (84). Although proper nutrition and physical activity are recognized as staples of good health and ASCVD prevention, these guidelines pay particular attention to the implementation of pharmaceutical treatment, which is discussed in the History and Physical Examination, Diagnostic Testing, and Treatment sections. Signs and Symptoms Given that most physical manifestations of dyslipidemic diseases are absent, these conditions are considered silent diseases. Because signs and symptoms are not physically evident, dyslipidemic diagnosis is based almost solely on the lipid profile itself; thus, the primary means for dyslipidemia diagnosis are beyond the scope of practice for the typical clinical exercise physiologist. Most importantly, each dyslipidemic condition requires laboratory testing to determine the specific abnormal blood lipid or lipoprotein level, allowing for classification and diagnosis. Remember that each type of dyslipidemia carries a different level of atherosclerotic disease risk, making the evaluation of individual lipid and lipoprotein levels crucial. In addition to rare symptoms caused by dyslipidemia, pharmaceutical treatment of dyslipidemia may cause side effects that, although not highly common, can sometimes be quite serious. These are discussed in the Treatment section. 174 Davis, Grandjean, Crouse, and Durstine Hypercholesterolemia Typical hypercholesterolemia has no outward physical symptoms; however, more severe forms of hypercholesterolemia are accompanied by physical signs. For example, familial hypercholesterolemia, a rare genetic condition of hypercholesterolemia, results in drastically reduced LDL clearance rates. As a result, blood total cholesterol levels can reach 500 mg/dL or more. Such extreme blood cholesterol levels can sometimes cause xanthomas (depositions of yellowish cholesterol-rich material) to form in tendons and subcutaneous tissues. Another more common genetic form is familial polygenic hypercholesterolemia, the most common cause of elevated serum cholesterol concentrations. In this case LDLc is mildly to markedly elevated (140-300 mg/dL), with serum triglyceride concentrations generally within the normal range (172). Another prominent genetic form is familial combined hypercholesterolemia, which is typified by elevations of both total cholesterol and triglyceride concentrations (27). All forms of hypercholesterolemia increase the risk for premature ASCVD; however, familial hypercholesterolemia carries the highest risk (75). Hypertriglyceridemia In addition to familial combined hypercholesterolemia, other genetic conditions can result in elevated triglycerides. Three primary isoforms of apo E exist—E2, E3, and E4. Apo E2 promotes hypertriglyceridemia and is associated with increased ASCVD risk due to poor binding to the apo E receptor. Because receptor binding is required for triglyceride hydrolysis and removal from blood, both blood chylomicrons and VLDL–triglyceride levels increase (46). Deficient levels of blood apo C-II, a cofactor for LPL activity, are also associated with elevated blood triglyceride levels because of limited clearance of chylomicrons and VLDL (25, 67). Familial LPL deficiency, usually found in childhood, is characterized by severe hypertriglyceridemia with episodes of abdominal pain, recurrent acute pancreatitis, and development of cutaneous xanthomas (14). Blood chylomicron clearance is impaired, causing blood triglycerides to accumulate and the plasma to appear milky. These physical signs usually decrease or resolve with restriction of total dietary fat to 20 g/d or less (67). Other genetic defects involving single nucleotide polymorphisms and haplotypes directly influence lipoprotein–triglyceride transport in the blood (156). Familial hypertriglyceridemia is caused by a genetic defect and is passed on in an autosomal-dominant fashion. In this condition, VLDLs are overproduced, causing elevated blood VLDL–triglyceride concentrations. Familial hypertriglyceridemia does not usually become noticeable until puberty or early adulthood. Obesity and high blood glucose and insulin concentrations often accompany this genetic form of dyslipidemia (67). History and Physical Examination Chapter 4 provides general interview and physical examination procedures. For the most part, the patient with hyperlipidemia or dyslipidemia will not present differently from the average person. An exception is the rare presence of xanthomas. If xanthomas are suspected in a patient who has not been previously diagnosed with hyperlipidemia or dyslipidemia, this should be brought to the attention of their physician. In some cases, lipid-lowering medications such as statins and fibric acid, when used individually or in combination, have the potential to cause muscle damage, releasing proteins such as myoglobin into the blood (e.g., rhabdomyolysis) and causing reduced exercise performance. Besides reducing exercise performance, these proteins are harmful to the kidneys. Elevated levels may lead to kidney dysfunction or failure and, in extreme cases, death (2, 57). Although rhabdomyolysis is fairly rare, patients on lipid-lowering medications who are experiencing sudden or increasing muscle pain should curtail exercise training and refrain from undergoing exercise testing until they have been cleared by their physician. Although hyperlipidemia and dyslipidemia can exist on their own, they are often accompanied by other conditions, such as obesity, diabetes mellitus, or ASCVD. Such conditions should obviously be taken into consideration when planning exercise testing or training. As long as potential comorbidities have been screened appropriately, patients with hyperlipidemia or dyslipidemia can safely complete exercise testing and begin a moderate-intensity exercise program that progresses to vigorous intensity (5). Diagnostic Testing Common dyslipidemias are diagnosed by obtaining a blood sample and measuring the blood lipid profile. Dyslipidemias traditionally were classified according to the Frederickson classification system, in which the pattern of lipoproteins was described using electrophoresis or ultracentrifugation (68). Later clinical classification for dyslipidemias was adopted from the NCEP-ATP III guidelines; updates establish priorities and rationale for treating and managing blood lipids for primary and secondary ASCVD prevention (161). Clinical screening for dyslipidemia includes analysis of the blood lipid profile measured every 5 yr, beginning at age 20. LDLc levels are measured directly or most likely estimated using the Friedewald formula (LDLc = total cholesterol − HDLc − [triglyceride/5]) (69). However, the Friedewald formula is not used when an individual’s triglyceride levels exceed 400 mg/dL. The basic lipid profile of total cholesterol, LDLc, HDLc, and triglyceride concentrations may not provide the clinician with enough information to properly diagnose some dyslipidemias. In these cases, the clinician may order more sophisticated analytic procedures to determine lipoprotein particle numbers and sizes or apolipoprotein concentrations (77). Another strategy for determining whether intermediate-risk or selected borderline-risk adults should undergo statin therapy is to obtain a coronary artery calcification score via computerized tomography, with detection of calcification generally indicating pharmaceutical treatment (84). Some clinicians include the measurement of postprandial lipids in patients who exhibit refractory hypertriglyceridemia (i.e., hypertriglyceridemia that remains after treatment). Assessment Chapter 11 Hyperlipidemia and Dyslipidemia of triglyceride and other lipid parameters, such as the number and size of TRLs, apolipoprotein responses, and calculated non-HDLc during the postprandial period, may help the clinician develop a specific therapeutic strategy for ameliorating the dyslipidemia (143, 149-151). Exaggerated triglycerides can prohibit an accurate assessment of the primary lipid target (LDLc), contribute to vascular endothelial dysfunction, and increase ASCVD risk (121, 149). A strong argument can be made that PPL is a serious but treatable condition since most people live in a postprandial state throughout the day and those with PPL may not fully clear blood lipids from a previous meal before eating again (121, 143, 149). Typically, a patient undergoes 8 to 12 h of overnight fasting before consuming a test meal with known amounts of fats, protein, and other macronutrients. Before ingestion of the test meal, blood samples are taken, and after the meal is ingested, blood samples are taken at timed intervals for up to 8 h. The changes in blood triglyceride are plotted and a removal curve is developed. The more quickly blood triglycerides are removed after the fat test meal, the less the ASCVD risk (122, 151). In 2018, the AHA and the American College of Cardiology (ACC) spearheaded the efforts of 12 medical associations to release the Guideline on the Management of Blood Cholesterol (referred to as “the Guideline” in the remainder of this chapter) (84). To assist with pharmaceutical decision making, the Guideline employs pooled cohort equations to determine 10 yr ASCVD risk. Patients who meet criteria for one of four high-risk groups are treated with statins using dosages supported by evidence from randomized controlled trials (188). The four groups identified in the Guideline are patients with ASCVD, patients with LDLc ≥190 mg/dL, patients between 40 and 75 yr of age with diabetes mellitus and not classified in groups 1 or 2, and patients between 40 and 75 yr of age with a 10 yr ASCVD risk of ≥7.5% and not classified in any of the other groups. LDLc is the primary target for intervention through the use of statins for high-intensity therapy (intended to lower LDLc by ≥50% of the baseline lipid profile) or moderate-intensity therapy (intended to lower LDLc by 30 to 49% of baseline), depending on risk group. Because evidence from randomized clinical trial data does not support specific LDLc targets for reducing ASCVD, monitoring LDLc is recommended to assess adherence to and need for adjustment of treatment regimens, rather than to reach specific LDLc concentrations. Although LDLc is the primary treatment target, severe hypertriglyceridemia (fasting triglycerides ≥500 mg/dL or ≥5.6 mmol/L) with a 10 yr ASCVD risk of ≥7.5% can also indicate statin therapy. When statin therapy does not perform to the extent expected, or when the optimal statin dose cannot be tolerated because of side effects, additional medications may be prescribed to help manage elevated LDLc or triglyceride. These medications are discussed in the Treatment section (84). The Guideline focuses on pharmaceutical treatment, but it also emphasizes lifestyle behavior modification to address both the primary and secondary treatment of hyperlipidemia and dys- 175 lipidemia. Physical activity, weight loss and weight management, dietary modification, and the consumption of functional foods are described in the Guideline. In addition, proper treatment (both behavioral and pharmaceutical) of secondary conditions (e.g., diabetes mellitus, hypothyroidism) can help control hyperlipidemia and dyslipidemia (84). Exercise Testing Secondary forms of dyslipidemia are void of signs and symptoms for other comorbidities (e.g., ASCVD or renal insufficiency), and in these cases exercise testing should follow established protocols used in populations at risk for ASCVD. Exercise testing protocols for individuals at risk for ASCVD have been developed by the AHA and the American College of Sports Medicine (ACSM) (see chapter 5). The possibility of dyslipidemia patients having latent ASCVD is higher than in the healthy population. Considering this possibility, the clinical exercise physiologist should be aware of and understand the contraindications for exercise and exercise testing for this group. Contraindications for general exercise testing have been identified and described by the ACSM and AHA (64). An in-depth review of each patient’s medical history is performed to find possible contraindications and to stratify the patient’s level of risk. As stated before, exercise is not contraindicated for individuals with a dyslipidemic blood profile. Rather, attention is given to possible existing comorbidities. When diseases are suspected or present, the protocols for testing a patient are likely to change. The clinical exercise physiologist should pay careful attention to the facility’s procedures for determining eligibility for exercise testing, including obtaining a physician’s release and having the appropriate personnel on hand for exercise and functional tests. The clinical exercise physiologist should be cognizant and observant of signs and symptoms that may suggest underlying or latent ASCVD while testing patients with dyslipidemia. Special considerations for cardiovascular, musculoskeletal, and flexibility testing of patients with hyperlipidemia and dyslipidemia follow. Cardiovascular Testing The purpose of cardiovascular testing in an individual with dyslipidemia is to help diagnose ASCVD, determine the functional capacity of the individual, and help determine an appropriate exercise intensity range. Various appropriate cardiovascular tests exist for the dyslipidemic population, and as previously stated, cardiovascular testing should follow established protocols unless comorbidities are present (see chapter 5). When comorbidities are present, testing protocols should allow for the needs of the individual and their condition(s). Also, when exercise testing and training are being considered for patients with a medical condition, a referral and a medical evaluation performed by a physician are required before testing (64). If a patient with dyslipidemia is otherwise healthy, the anticipated responses to an exercise test are no different from what is 176 Davis, Grandjean, Crouse, and Durstine expected in healthy individuals. However, some special circumstances may exist. Prescribed medications can alter anticipated exercise responses. Unexplained muscle pain can indicate rhabdomyolysis, and the patient should be evaluated by a physician before exercise testing. Also, chronically high cholesterol levels can result in the formation of tendon xanthomas. Individuals with this condition may experience biomechanical problems that contribute to reduced exercise performance. Exercise testing is a crucial step in evaluating the health and fitness status of patients with dyslipidemia. This information is extremely valuable for creating an exercise prescription. Musculoskeletal Testing The purpose of musculoskeletal testing in a patient with dyslipidemia is to determine musculoskeletal strength, endurance, and performance. Various appropriate muscular strength and endurance tests are available for use in the dyslipidemic client. Most often one-repetition maximum tests are used; however, if comorbidities are present, other tests may be employed (159). Other less hazardous tests include the estimation of one-repetition maximum (see chapter 5). Special attention should always be given to any possible orthopedic issues the patient may have, as well as to potential tendon xanthomas in patients with severe hypercholesterolemia and to statin-induced myalgia (muscle soreness). Flexibility Testing The purpose of flexibility testing in a patient with dyslipidemia is to determine the range of motion in all joints and, most practically, to assist in the assessment of functional abilities as they relate to exercise prescription and activities of daily living. Patients with dyslipidemia can perform testing protocols for the healthy population (see chapter 5). Treatment The primary goal in the clinical management of dyslipidemias is to reduce the global risk for ASCVD. Attention focuses on reducing the severity and number of traditional ASCVD risk factors. The current goals and recommendations for managing dyslipidemia are described in consensus statements for dyslipidemia, high blood pressure, obesity, diabetes, and physical activity (51, 84, 181, 208). Aggressive treatment, often including the use of lipid-lowering medication, is of primary importance for preventing future ASCVD outcomes in dyslipidemia patients with documented or known disease (8, 63, 85, 97, 99, 195). The AHA advocates teamwork by physicians and allied health professionals (e.g., nutritionists, nurses, clinical exercise physiologists) to help patients normalize their blood lipids and improve their health. The fundamental interventions for individuals with dyslipidemia are to engage in regularly practiced physical activity, consume a heart-healthy diet, lose weight, and prevent weight regain after weight loss. All characteristics of secondary dyslipidemia are mitigated when these lifestyle behaviors are consistently practiced (63). In addition, efforts should be made to quit smoking, improve stress management, and practice individually appropriate behavioral techniques for long-term adherence to healthy lifestyle changes (8, 63, 106). A clinician’s guide explains how to prescribe exercise for most patients and delineates useful strategies for promoting healthy lifestyle changes (29). Strategies for improving patient adherence to lifestyle behavior changes are also available to the physician and members of the collaborative health care team (59, 63, 106). Diet Adopting a healthy diet is crucial for treating dyslipidemia and managing healthy blood lipid concentrations (8, 82, 106, 116, 130, 219). Evidenced-based dietary recommendations from the AHA, the NCEP-ATP III, and the Dietary Approaches to Stop Hypertension (DASH) diet are widely used in clinical practice (36, 106, 125, 161). A Mediterranean-style diet is consistent with the National Lipid Association’s recommendations for a dietary fat intake of 25% to 35% of total calories (106). This type of diet, composed of whole grain foods, fruit, vegetables, fish, fiber, and nuts, has demonstrated efficacy in mitigating ASCVD risk and reducing future ASCVD events (179). The benefits of a Mediterranean-style diet partly come from the low content of saturated fats, trans fats, cholesterol, sodium, red meat, simple sugars, and refined grains (19, 63, 195). In addition, the Mediterranean diet provides nutrients such as omega-3 fatty acids, polyunsaturated fatty acids, soluble fiber, and carotenoids, all of which are associated with cardiometabolic health (19). Although red wine is often associated with the Mediterranean diet, the health benefits of moderate alcohol consumption, such as an increase in HDLc and enhanced vascular endothelial function, appear to be unrelated to the type of alcoholic beverage that is consumed (49). Adopting a Mediterranean diet will help individuals with dyslipidemia balance macronutrient intake and avoid the temptation to try one of the ever-present fad diets. A fad diet may generally be characterized as one that is based on omitting an important food group and promising unsupported benefits. Current fad diets include those that recommend high or low-fat intake, high carbohydrate consumption, or high protein intake. Except for Mediterranean fare, diets with fat intake exceeding 35% are likely to include too much saturated fat, contributing to elevated LDLc, insulin resistance, and weight gain (82, 125). Low-fat, high-carbohydrate diets promote features of atherogenic dyslipidemia, such as elevated triglycerides and lower HDLc. High-protein diets can increase blood phosphorus levels and may lead to hypercalciuria, acidosis, and insulin resistance, especially in individuals with poor renal function (82, 125, 219). Functional foods contain biologically active substances that impart medicinal or health benefits beyond their basic nutritional components. These foods appear promising in the prevention and treatment of ASCVD (184). What makes functional foods attractive to the clinician and patient is that they do not appear to have major side effects and therefore are safely incorporated into the diet (63). In addition, functional foods can impart health benefits Chapter 11 Hyperlipidemia and Dyslipidemia 177 when substituted into or added to the current diet without major alterations in eating habits or changes in nutrient intake. For individuals who are taking lipid-lowering medications, the effects of drug therapy can be augmented when combined with functional foods because these foods exert their effects differently from medications (15, 106). Functional foods that can be effective for blood lipid management include soluble fiber, plant stanols and sterols, psyllium, flaxseed, a variety of nuts, omega-3 fatty acids, garlic, flavonoids found in dark chocolate, and soy protein. Literature reviews on the topic provide a more thorough understanding of the proposed mechanisms of action and lipid-lowering effects (15, 93, 173, 184). The AHA’s recommendations for managing dyslipidemias delineate a practical approach to including functional foods in an individualized plan (63). Dietary habits are hard to break, and the clinician must think beyond itemized recommendations for dietary nutrient composition. Patients should be provided with resources to make incremental and lasting behavioral changes in meal planning, food selection, and food preparation. Contingencies for dietary challenges, such as food choices and portion control when dining out, and habits associated with altered eating patterns need to be addressed (8, 59, 63). Weight Loss Physical Activity and Exercise Table 11.3 summarizes the major medications prescribed to treat hyperlipidemia and dyslipidemia. The primary targets for lipidlowering therapy are LDLc and non-HDLc (105, 188). Statin medications are the therapy used to lower LDLc (104). In standard dosages, statins typically reduce LDLc by 18% to 55%, but they vary in their efficacy for lowering triglycerides, increasing HDLc, and modifying other aspects of atherogenic dyslipidemia like small, dense LDL particles (83). In addition to its lipid-lowering effects, statin treatment can reduce serum uric acid levels (9), improve renal function (10, 144), and lower ASCVD events in patients with metabolic syndrome and diabetes mellitus (1, 18, The amount of physical activity recommended for improving and maintaining health in most adults is very attainable. The health benefits of regularly practiced physical activity, such as improved triglyceride and HDLc concentrations, blood pressure, blood glucose control, and cardiovascular function, can be enjoyed regardless of weight change or in the absence of noticeable improvements in fitness (8, 33, 92, 107, 124, 131). The following section on exercise prescription discusses the amount and type of physical activity appropriate for individuals with dyslipidemia. Weight loss is a top priority for overweight and obese patients with dyslipidemia. The current recommendations are to achieve a 7% to 10% reduction in body weight over a 6- to 12-month period through caloric restriction and increased physical activity. Most scientists and practitioners agree that this target is best attained through a modest reduction in daily caloric intake (i.e., lowering total calories by 500 to 1,000 kcal) (51, 96). Achieving a modest weight loss of 7% to 10% will positively affect all lipid characteristics (96, 160, 218). Daily physical activity adds to the caloric deficit and imparts health benefits that may not be achieved through hypocaloric diets alone (35, 51, 92, 116, 206). Weight loss achieved through healthy lifestyle behavior can be the most effective means of preventing and treating dyslipidemia (194, 209, 212, 214). Additional health benefits can be realized with greater weight loss and long-term maintenance of the lower body weight (51, 135, 160, 165, 206). Therefore, individuals who respond well to the initial weight loss goal should be encouraged to continue behaviors conducive to further weight loss or maintenance of their new lower body weight. Pharmacology: Lipid-Altering Medications Practical Application 11.1 EFFECTS OF GRAPEFRUIT Although grapefruit and grapefruit juice are healthful, providing many important nutriments, they can interact with dozens of medications and have undesirable effects. The chemicals in grapefruit do not interact directly with the medication. Rather these chemicals bind to an intestinal enzyme, blocking its action and making the passage of the medication from the gut to the bloodstream easier. As a result, blood medication levels may rise faster and remain at higher than normal levels; in some cases, the abnormally high levels are dangerous. Regarding statins and grapefruit use, some statins are affected more than others. For example, blood levels of atorvastatin (Lipitor), simvastatin (Zocor), and lovastatin (Mevacor) are boosted more than fluvastatin (Lescol), pravastatin (Pravachol), or rosuvastatin (Crestor) after grapefruit juice consumption. As a general recommendation, anyone taking a statin should not consume grapefruit or grapefruit products. The patient should contact their dietitian, pharmacist, or physician with any questions concerning grapefruit or grapefruit juice consumption and statin use. 178 Davis, Grandjean, Crouse, and Durstine 39, 61, 163, 180, 186). Statins are so named because statin is the common suffix (e.g., atorvastatin, rosuvastatin) of the medication class more formally called 3-hydroxy-3-methylglutaryl coenzyme A (HMG-CoA) reductase inhibitors. These medications interfere with a key enzyme responsible for cholesterol synthesis. As such, statins inhibit cholesterol production by the liver, and because of the resulting lower intracellular cholesterol availability, the number of LDL receptors increases in hepatic and other tissues. Downstream effects are stabilization of atherosclerotic lesions and decreased endothelial dysfunction. The most common side effects reported with statin therapy are elevated liver enzymes, myopathy, myalgia, and dyspepsia (190, 191). Statins should be avoided or discontinued in patients with liver disease or elevated liver enzymes and during pregnancy (58, 123, 155). When very high-risk ASCVD patients (history of multiple major ASCVD events or a single ASCVD event with multiple high-risk conditions) and patients with primary hypercholesterolemia (LDLc ≥190 mg/dL or ≥4.9 mmol/L) do not achieve desired LDLc concentrations with maximally tolerated statin doses, supplemental lipid-lowering medications may be prescribed (84). The first such drug recommended is ezetimibe. Ezetimibe inhibits cholesterol uptake by the intestine, resulting in a typical reduction in LDLc of 13% to 20% and upregulation of LDL receptors. Compared with statins, ezetimibe’s side effects are less common and less severe, but they may include gastrointestinal discomfort and worsening of statin-induced myalgia. A similar class of medications, bile acid sequestrants, may be prescribed instead of or in addition to ezetimibe. Bile acid sequestrants promote cholesterol elimination through the digestive tract by binding bile acids in the intestine. Although LDLc tends to decrease by 15% to 30%, compliance is poor because the medicine is unpalatable to most patients (it is administered in powder form) and it can cause considerable gastrointestinal discomfort. The use of bile acid sequestrants may not be indicated for some patients because these medications interfere with the absorption of several pharmacological agents, such as digoxin, thyroid hormones, and warfarin. They can also increase triglyceride concentration, especially in patients who are already hypertriglyceridemic (58). Otherwise, the potential for harmful side effects is rather low, so bile acid sequestrants may be prescribed to younger individuals facing long-term pharmacologic management of dyslipidemia (83). In addition to elevated triglyceride concentrations, constipation and gastrointestinal disturbances are commonly reported. If the addition of an intestinal-acting medication to statin therapy does not result in the desired LDLc concentration in highrisk patients, a newer medication class, proprotein convertase subtilisin/kexin type 9 (PCSK9) inhibitors, may be prescribed (84). PCSK9 is an endogenous protease that binds to the LDL receptor and renders it inactive once taken into the hepatocyte via endocytosis. PCSK9 inhibitors, administered through subcutaneous injection once or twice monthly, are monoclonal antibodies that bind to PCSK9, which prevent it from binding the LDL receptor, allowing the LDL receptor to be recycled back to the hepatocyte surface once LDL has been removed. PCSK9 inhibitors are usually very effective in lowering LDLc (43%-64%) and appear to be fairly safe (upper and lower respiratory tract infections and injection site reactions are the most common side effects). However, at this writing, safety has not been validated past 3 yr. The medications are also quite expensive, with projected lifetime cost exceeding the projected monetary savings from the prevention of cardiac events. Studies in the United States revealed that only 1.5% of patients with primary hypercholesterolemia receive PCSK9 medications, and when prescribed, this therapy was approved for reimbursement in only 61% of Medicare cases and 24% of third-party provider cases (98). In 2020, the U.S. Food and Drug Administration approved bempedoic acid for the treatment of hyperlipidemia. Like statins, this medication class inhibits hepatic production of cholesterol, but it interrupts the process earlier in the synthetic pathway. Clinical trials have shown bempedoic acid to reduce LDLc by 15% to 25% (16) and up to 38% when combined with ezetimibe (192). Evidence suggests that side effects are relatively low, with the most common being elevated uric acid, nasopharyngitis, and urinary tract infections. Although there are no current guidelines on the use of bempedoic acid, it is anticipated that it might substitute for statin therapy when that is not well tolerated. At this writing, a clinical trial is ongoing to determine whether bempedoic acid can reduce ASCVD events (17). Elevated fasting and postprandial triglycerides, low HDLc, and small, dense LDL particles can still contribute to residual ASCVD risk after LDLc goals are achieved (22, 83). This atherogenic dyslipidemia is approximated in the clinic through calculation of nonHDLc and measurement of triglyceride concentrations (48, 121). A triglyceride concentration of ≥175 mg/dL (1.9 mmol/L) indicates significant elevation of VLDL in the blood, and a level ≥500 mg/ dL (5.6 mmol/L) indicates significant presence of chylomicrons (84). Although statins are typically considered as first-line therapy for hypertriglyceridemia, an additional medication is sometimes needed to bring triglyceride concentration below 500 mg/dL, which is important to prevent the onset of pancreatitis. In addition to pharmaceutical therapy, attention should be paid to secondary factors that can cause blood triglyceride levels to rise, such as obesity, diabetes, hypothyroidism, and medications that might increase triglycerides (e.g., oral estrogens, β-blockers, thiazide diuretics, glucocorticoids, rosiglitazone, bile acid sequestrants). Omega-3 fatty acid supplementation can also reduce triglyceride concentration. Importantly, a meta-analysis showed that each 1 g/d of eicosapentaenoic acid was associated with a 7% reduction in major ASCVD events, while no significant association was shown with docosahexaenoic acid (138). Fibrates and niacin are both effective for lowering triglycerides, increasing HDLc, and increasing LDL particle size (82, 130, 195, 203). Fibrates reduce fatty acid uptake by the liver, thereby slowing hepatic triglyceride synthesis and VLDL production. They may Chapter 11 Hyperlipidemia and Dyslipidemia 179 Table 11.3 Pharmacology Medication class and name Primary effects Exercise effects Important considerations HMG-CoA reductase inhibitors Atorvastatin (Lipitor) Pravastatin (Pravachol) Rosuvastatin (Crestor) Simvastatin (Zocor) Others Blocks cholesterol synthesis; increases tissue LDL receptors No direct effect Benefits: convenient dosage schedule; useful in those with multiple risk factors; low drug interaction potential; low level of system toxicity Side effects: increased liver enzymes; myopathy; myalgia; dyspepsia Contraindications: liver disease; known or suspected pregnancy; in some instances, use with erythromycin, antifungal agents, cyclosporine Cholesterol uptake inhibitor Ezetimibe [Zetia]) Inhibits intestinal cholesterol uptake No direct effect Benefits: indicated when LDLc goal is not met with maximally tolerated statin dose; side effects generally less severe than those of statins Side effects: fever; gastrointestinal discomfort; headache; myalgia; upper respiratory tract infections Contraindications: liver disease; known or suspected pregnancy Bile acid sequestrants Cholestyramine (Prevalite, Questran) Colestipol (Colestid) Binds bile acids in the intestine (facilitates cholesterol excretion) No direct effect Benefits: low system toxicity; use permitted in children and during pregnancy Side effects: GI distress; constipation; interferes with absorption of warfarin, digoxin, and thyroxine Contraindications: bile duct obstruction; dysbetalipoproteinemia; triglyceride >200 mg/dL PCSK9 inhibitors Alirocumab (Praluent) Evolocumab (Repatha) Inhibits LDL receptor catabolism No direct effect Benefits: can be prescribed when LDLc goal is not met with maximally tolerated statin dose and ezetimibe or bile acid sequestrant Side effects: upper and lower respiratory tract infections; injection site reaction Contraindication: hypersensitivity to the medication Bempedoic acid (Nexletol) Blocks cholesterol synthesis No direct effect General: Recently approved by FDA; not yet shown to lower ASCVD events (research is ongoing); available as a combination medication with ezetimibe Benefits: may be prescribed when LDLc goal is not met with maximally tolerated statin dose and/or ezetimibe; converted to active form in liver but not muscle, lowering likelihood of myalgia Side effects: hyperuricemia and gout symptoms in patients with history of gout; tendon damage, especially if over age 60 or if taking corticosteroids or fluroquinolones; upper respiratory tract infection; urinary tract infection Fibric acid derivatives Fenofibrate (Fibricor, Triglide, Trilipix, others) Gemfibrozil (Lopid) Decreases triglyceride synthesis and VLDL production; increases lipoprotein lipase activity No direct effect Benefits: used with well-controlled diet to reduce the number of small, dense atherogenic LDL particles; is generally better tolerated than niacin; also decreases fibrinogen levels Side effects: GI distress; gallstones; myopathy; increased excretion of uric acid Contraindications: renal disease; liver disease Nicotinic acid Decreases VLDL production; decreases liver clearance of HDL particles No direct effect Benefits: low cost and availability (OTC); favorably improves all atherogenic components of the lipid profile; often used with bile acid binding resins Side effects: flushing; GI distress; hyperglycemia; hyperuricemia Contraindications: severe hypotension; liver disease; diabetes; gout; hyperuricemia; active peptic ulcer LDL = low-density lipoprotein; LDLc = LDL cholesterol; GI = gastrointestinal; PCSK9 = proprotein convertase subtilisin/kexin type 9; VLDL = very low-density lipoprotein; HDL = high-density lipoprotein. 180 Davis, Grandjean, Crouse, and Durstine also lower serum triglyceride and increase HDLc by stimulating the activity of LPL (155, 157). Common side effects of fibrates include constipation and those reported for statins. Fibrates are contraindicated for people with hepatic or renal dysfunction (81). Of the two main fibrates, fenofibrate may be prescribed as an adjunct to statin therapy, but the Guideline recommends against prescribing gemfibrozil with statins because of its higher risk of severe myopathy (84). Niacin primarily reduces serum triglyceride and LDLc by suppressing hepatic synthesis of VLDL. A long-standing limitation for niacin has been poor tolerance of its side effects (23), although extended-release forms have improved tolerance. Niacin therapy often results in flushing, skin rashes, gastrointestinal problems, and pruritus (58, 157). It is not recommended for individuals with hypotension, liver dysfunction, or peptic ulcers or for patients with diabetes mellitus because it can increase blood glucose concentrations (81). Prescription of niacin has decreased in recent years after two large, randomized trials failed to demonstrate a reduction of ASCVD events or mortality when niacin was added to statin therapy in order to increase HDLc (79, 103). However, a systematic review and meta-analysis suggests that niacin monotherapy may reduce the incidence of acute coronary syndrome, stroke, and revascularization (44). Even so, the Guideline does not mention niacin as a major option in lipid management (84). When encountering patients on niacin therapy, the clinical exercise physiologist should remind the patient to take this medication before going to sleep at night to avoid experiencing the symptoms of flushing and hypotension during the day and, especially, with exercise. The clinical exercise physiologist should make the patient aware of these side effects so they may be distinguished from symptoms of heavy exertion. Up to 25% of patients taking lipid-lowering medications, particularly statins and fibric acid derivatives, may experience some form of medication intolerance that includes muscle inflammation and muscle damage (2). Rhabdomyolysis, a severe side effect arising from medication-induced muscle damage, is characterized by the presence of myoglobin in the blood. Rhabdomyolysis is also characterized by reduced and dark urine output and a general feeling of weakness. Since kidney damage may result, a patient experiencing these symptoms should immediately contact their physician. Choosing to follow a Mediterranean-style diet, incorporating functional foods in the diet, exercising regularly, and even modest weight loss are each effective means for improving blood lipid levels. When these strategies are combined, they may work in concert to improve blood lipids. If patients respond conservatively to each of these therapeutic lifestyle changes, they may expect to lower LDLc and triglyceride levels and improve HDLc to the same magnitude that would be expected with lipid-lowering medications (63, 106). For patients who are not on lipid-lowering medication, the lifestyle strategies could be enough to keep them off these medicines. For patients already taking these drugs, lifestyle strategies may add to their medicine’s effect. However, this does not always occur, and further research on combination therapy is clearly needed (3, 158). For all patients, these therapeutic lifestyle changes will provide health benefits above and beyond what can be attained with lipid-lowering medication alone. EXERCISE PRESCRIPTION Exercise can have a profound impact either directly or indirectly (i.e., weight changes) on blood lipid and lipoprotein levels. Although various exercise modalities can provide health benefits that may ultimately improve lipid status, the majority of evidence suggests that cardiorespiratory exercise, or endurance-type activity, is preferred, although a yet-defined combination program may be optimal. Nevertheless, the specific doses for optimizing improvements have not been completely defined. Here we review currently recommended programming. Cardiorespiratory Exercise The ranges of physical activity volumes recommended in the 2008 U.S. Department of Health and Human Services’ Physical Activity Guidelines for Americans (182) and informed by position statements from the ACSM (37, 92, 148) are appropriate for individuals with dyslipidemia (table 11.4). These guidelines suggest ranges of 150 to 300 min of moderate-intensity or 75 to 150 min of vigorous-intensity physical activity per week, or some combination of the two. A dose–response relationship is recognized, with physical activity at the upper ends of these ranges likely to result in more positive health benefits. Indeed, some of the earliest research investigating the effect of exercise training on lipid and lipoprotein concentrations indicated that a threshold of 8 to 10 mi (12.9 to 16 km) of running per week is necessary to significantly change HDLc concentration (211, 213). Because the lipid- and lipoprotein-related benefits of physical activity rely more on the total volume of activity performed than on the intensity of the activity (54, 124), walking an equivalent distance is thought to yield similar benefits. Assuming common walking and running speeds (e.g., 3 and 6 mph [4.8 and 9.7 kph], respectively), this 8 to 10 mi threshold falls within the lower end of the moderate-intensity and vigorous-intensity time ranges mentioned. In support of a dose–response relationship, Kraus and colleagues (124) reported greater favorable changes in LDL particle size and HDLc and triglyceride concentrations in dyslipidemic men and women who averaged 20 mi (32 km) of running per week versus those who ran or walked an average of 12 mi (19 km) weekly. In addition, only doses of exercise training as high as or higher than that listed in table 11.4 resulted in improved cholesterol efflux from cultured macrophages exposed to plasma or serum from overweight or obese adults, suggesting that higher exercise training volumes may be necessary to improve certain aspects of HDL function (178). Therefore, although fulfilling the minimum recommendations in the Physical Activity Guidelines for Americans is likely beneficial, exercising at or slightly above the upper end of these guidelines may be necessary to optimize Chapter 11 Hyperlipidemia and Dyslipidemia 181 Practical Application 11.2 CLIENT–CLINICIAN INTERACTION When interacting with patients who have hyperlipidemia and dyslipidemia, the clinical exercise physiologist must clearly outline the dangers and implications of having altered levels of cholesterol. This will help communicate the seriousness of the patient’s condition and the importance of ameliorating it. The patient can then be given information about how to improve their condition, including the benefits of high-volume aerobic exercise. The clinical exercise physiologist needs to be simple and direct in highlighting the improvements seen in HDL and triglycerides with exercise training. In addition, emphasis should be placed on the beneficial effects of exercise on overall ASCVD risk. This information is particularly important for patients with multiple risk factors. Regarding aerobic training, the patient needs to understand that the benefits are increased with greater exercise energy expenditure (i.e., approximately 300 min/wk of moderate-intensity physical activity or 150 min/ wk of vigorous-intensity physical activity). Daily or near-daily exercise is advocated. Daily physical activity may be split into two or more sessions, if needed, to attain the desired exercise volume (181). The safest approach to accomplishing this goal is to begin an exercise program with a nonfatiguing amount of moderate-intensity exercise and to gradually build the volume (frequency, duration, intensity, or a combination) lipid and lipoprotein metabolism. In fact, in some cases, practice of physical activity at the lower end of the recommended levels may result in a stabilization of lipid and lipoprotein levels rather than an actual improvement. It should be noted, however, that a sedentary person’s lipid and lipoprotein profile is likely to worsen over time, particularly if they are gaining weight, so such stabilization should be recognized as a positive effect if lipid and lipoprotein levels had previously been getting worse. In addition, as mentioned in the next section on exercise training, regularly practiced physical activity can directly affect the metabolism of some lipids and lipoproteins, while alteration of others may depend on changes in body composition. Therefore, energy expenditure at the upper end of the continuum, combined with prudent nutrition, is the best behavioral prescription for improving the overall blood lipid and lipoprotein profile. Although progressing toward 300 min/wk of moderateintensity or 150 min/wk of vigorous-intensity physical activity is recommended, little research is available to suggest the number of days per week the activity should be performed. Studies employing single sessions of exercise typically show that lipid and lipopro- over a number of weeks (5). This method is prudent in terms of both safety and program compliance and may also help distinguish normal exercise-induced muscle soreness from myalgia that can occur from statin or fibrate treatment. Patients should be instructed to inform the clinical exercise physiologist whenever their medications are changed or the dosages are increased. In such cases, increases in exercise volume should be withheld for a couple of weeks, and any unusual muscle soreness should be noted. In addition, any sudden or severe muscle pain that cannot be logically explained by a recent increase in physical activity should be brought to the attention of both the patient’s physician and clinical exercise physiologist. Along with aerobic exercise, a calorie-restricting diet can be an effective tool in decreasing total cholesterol, non-HDLc, LDLc, and triglycerides while increasing HDLc. Therefore, the clinical exercise physiologist should ultimately encourage the client to combine high volumes of aerobic exercise of moderate to vigorous intensity with a calorierestricting diet to result in high weekly caloric deficits. The collaborative team approach, invoking the knowledge and expertise of the clinical exercise physiologist and the clinical nutritionist and the advocacy and guidance of a physician, is strongly recommended. tein concentrations change the most 1 or 2 d afterward and that they return toward baseline by 3 d. In addition, these short-term benefits likely accumulate from one exercise session to the next (i.e., exercising before lipid and lipoprotein concentrations return to baseline may stimulate further improvement) (6, 91, 202). Thus, a sensible approach might be to allow no more than 1 or 2 d of inactivity between exercise sessions. This routine would require performing leisure-time physical activity on at least 3 d across the week. Because of the amount of time involved, most people require 5 d/wk or more to complete the optimal amount of moderate-intensity physical activity. On the other hand, a person’s daily dose of physical activity does not need to be completed all at once. Limited research indicates that breaking physical activity up into three sessions of 10 min or more within a day is at least as effective in altering blood lipid and lipoprotein concentrations as is continuous physical activity (4, 141, 147). In summary, people should accumulate 150 to 300 min of moderate-intensity or 75 to 150 min of vigorous-intensity dynamic physical activity using large muscle groups, or some combination of the two, throughout each week. To optimize lipid and lipopro- 182 Davis, Grandjean, Crouse, and Durstine Table 11.4 Exercise Prescription Review Time (Duration) Type (Mode) Work toward continuous moderateintensity activity: 40%59%. HRR or VO2R; in absolute terms, 3-6 METs is generally recognized as moderate intensity At least 30 min/d Dynamic, largemuscle exercise (walking, jogging, cycling, elliptical training) Increase duration and frequency. Work toward daily activity beyond moderate intensity; intensity does not appear to be as influential as duration. More positive benefits are observed as the total volume of activity increases, which can be best reflected by increasing duration. Follow the general prescription guidelines for cardiorespiratory exercise found in chapter 6. 2 or 3 sessions per week on nonconsecutive days 8-12 reps for most exercises; for those new to resistance training and older individuals, 8-15 reps for most exercises 1 set of 8-10 exercises involving all major muscle groups Body weight, free weights, bands, water exercises Increase repetitions to achieve upper end of range (e.g., 12-15 reps) and then add resistance so that 8 reps can be achieved. Sets may be added, but training time is doubled with this option. Follow the general prescription guidelines for resistance exercise found in chapter 6 At least 2 sessions per week Stretching to the point of feeling tightness or slight discomfort Static stretches: hold for 10-60 s PNF stretches: 3-6 s of light to moderate contraction (20%-75% of MVC) followed by 10-30 s of assisted stretching; work toward 60 s for each exercise Repeat each stretch 2-4 times Static (active or passive), dynamic, ballistic, and PNF stretches are all effective Increase frequency to a daily routine. Also consider neuromotor exercises (e.g., activities such as yoga and tai chi that focus on balance agility, coordination, and gait) to maintain ROM and physical function. Training method Frequency Intensity Cardiorespiratory At least every other day Resistance Range of motion Progression Important considerations. HRR = heart rate reserve; VO2R = oxygen uptake reserve; METs = metabolic equivalents of task; MVC = maximal voluntary contraction; PNF = proprioceptive neuromuscular facilitation. Chapter 11 Hyperlipidemia and Dyslipidemia tein changes, clients are encouraged to progress to the upper end of the exercise dose range. Leisure-time physical activity should be practiced on at least 3 d throughout the week, and 5 d or more are required for most people to meet the dose recommendations. Moderate- to vigorous-intensity physical activity may be accumulated throughout the day and does not necessarily need to be accomplished within a single daily session. Resistance Exercise Resistance training alone may have a very limited effect on improving blood lipid and lipoprotein concentrations (54, 114). However, given that resistance exercise has numerous benefits not associated with lipoprotein metabolism, patients with dyslipidemia should still follow the recommendations presented in chapter 6. Focusing on a higher than minimally recommended volume of resistance training is not necessary, unless needed to improve other aspects of health. Range of Motion Exercise Although it provides no known benefit to lipid and lipoprotein profiles, range of motion exercise is important to overall fitness and should be practiced as discussed in chapter 6. Unless there are certain comorbidities, no special considerations for dyslipidemia patients exist. EXERCISE TRAINING Exercise is a valuable therapeutic treatment for improving blood lipids and reducing ASCVD risk (38, 40, 95, 131, 205). Several studies and decades of research provide accumulating evidence of the benefits of exercise training on blood lipids and lipoproteins. Although many initial studies were either cross-sectional or were conducted in individuals with normal lipid concentrations, more recent approaches have attempted to clarify the expected improvements among dyslipidemia patients. Cardiorespiratory Exercise Blood lipid profiles of physically active groups generally reflect a reduced risk for the development of ASCVD compared with those of their inactive counterparts (53, 65, 154). Strong evidence exists for the presence of lower triglyceride and greater HDLc concentrations in physically active individuals. Triglyceride levels are almost always lower in endurance athletes, aerobically trained people, and physically active individuals when compared with sedentary controls. Significant triglyceride differences of up to 50% exist between these groups in over half of all related cross-sectional studies. Blood levels of HDLc are between 9% and 59% higher in those having physically demanding jobs and in individuals engaged in endurance exercise compared with their less active counterparts (53, 54, 205). Only limited evidence suggests that people who are physically active exhibit lower concentrations of total cholesterol and LDLc concentrations than those who are less active. 183 In longitudinal studies, total cholesterol and LDLc infrequently change with exercise training in either men or women. When these lipid fractions are altered with exercise training, the reductions are minimal or moderate, averaging only 4% to 7% when compared with values in nonexercising control subjects (53, 54, 86). HDLc and triglyceride are more responsive to regular exercise than are total cholesterol and LDLc, based on the frequency of reported changes. Significantly greater HDLc concentrations are reported after exercise training in over half of the reviewed publications, while reductions in triglyceride levels are found in a third of the related literature (53, 54, 205). When HDLc is significantly elevated after exercise training, the increases are similar in men and women, ranging from 4% to 22%. In addition to increasing HDLc, exercise boosts the antioxidant properties of HDL (189, 197). Combined with reductions in triglyceride, the elevated antioxidant potential of HDL is thought to attenuate the inflammation and oxidative stress that contribute to vascular dysfunction and ASCVD (139, 164, 170, 174, 207). Significant reductions in triglyceride concentrations range from 4% to 37% after aerobic exercise training in males; the magnitude of change is similar in women but is seen less frequently (table 11.5). Resistance training also has positive but more modest effects on blood lipid and lipoprotein concentrations than observed for aerobic exercise (113, 114). Exercise and Dyslipidemia A meta-analysis of lipid changes in normo- and hyperlipidemic groups suggested that exercise training may have only limited influence in lowering total cholesterol and LDLc. The effects of exercise training on HDLc and triglyceride also favored more conservative estimates, with HDLc increases averaging 4% and triglyceride decreases averaging 6% to 19% (86). LDLc is lowered by a modest 5.5 mg/dL (95% CI: −9.9 to −1.2 mg/dL) through regularly practiced walking programs lasting 8 wk or more (119). In men, the pooled average reduction in total cholesterol and increase in HDLc were only 2%, whereas triglyceride was decreased by 9% (113). In both men and women, walking lowered non-HDLc by a pooled mean of 5.6 mg/dL (95% CI: −8.8 to −2.4 mg/dL), which equated to a modest 4% decrease (118). The pooled estimated changes in total cholesterol, LDLc, HDLc, and triglyceride concentrations indicate that individuals with documented ASCVD respond similarly to regular exercise training (115). Solid evidence exists that regular moderate-intensity aerobic exercise can increase LDL and HDL particle sizes in men and women (87, 124). In women, however, the lipid responses appear to be more favorable among those who are at greater risk for heart disease versus their healthy counterparts (134). Overweight and Obesity Meta-analytic results of 13 studies and 31 groups of overweight and obese adults were strikingly similar to those reported for most adults regardless of weight status (86, 113, 118). Total cholesterol 184 Davis, Grandjean, Crouse, and Durstine Table 11.5 a Lipid and Lipoprotein Changes Associated With Exercise Lipid/Lipoprotein Single exercise session Exercise training Triglyceride Decrease of 7% to 69% Approximate mean change 20% Decrease of 4% to 37% Approximate mean change 24% Cholesterol No changea No changeb LDL cholesterol No change No change Small, dense LDL cholesterol particles Literature unclear Can increase LDL particle size, usually associated with triglyceride lowering Lp(a) No change No change HDL cholesterol Increase of 4% to 18% Approximate mean change 10% Increase of 4% to 18% Approximate mean change 8% No change unless the exercise session is prolonged (see text). b No change if body weight and diet do not change (see text). decreased by 2% with endurance exercise training of at least 8 wk. Triglycerides were lowered by 11%, and LDLc and HDLc were not significantly affected (117). More rigorous physical activity programs are required for weight loss, and they result in greater health benefits (51); healthful changes in blood lipids and lipoproteins, particularly total cholesterol and LDLc, are greatest when physical activity is accompanied by weight loss (209, 210, 212). These findings are consistent with an initial meta-analysis of 95 studies examining the question of exercise-induced lipid changes with and without weight loss (196). Immediate and Transient Effects of a Single Exercise Session The characteristic antiatherogenic lipid profile of physically active and exercise-trained individuals was recognized long ago as primarily a transient response to the last session of physical activity or exercise and independent of chronic exercise training (152). Early support for this acute response hypothesis came from studies showing that blood triglyceride concentration was reduced for up to 2 d after a single aerobic exercise session, and this beneficial reduction was evident even in men with hyperlipidemia (31, 101, 152). Pioneering work in this field provided evidence that HDLc concentrations were higher and total cholesterol concentrations lower shortly after a single session of exhaustive exercise (56, 120, 190). Total cholesterol and LDLc responses to a single exercise session are highly variable. Small postexercise reductions in total cholesterol (3%-5%) have been reported for male and female hyper- and normocholesterolemic subjects (24, 41, 42, 78, 109, 162). Lower serum LDLc has been reported in trained men immediately and up to 72 h after completion of intense endurance events (24, 60) and in women after exercise of relatively high intensity and volume (76, 162). In contrast, LDLc remained unaffected in normolipidemic obese women after 1 h of exercise (216), and LDLc concentration may increase or decrease 5% to 8% in hypercholesterolemic men after exercise (41, 42, 78). Low-density lipoprotein particle size, a measure of the atherogenic potential of LDL, was not altered in either normal or hypercholesterolemic women by a single aerobic exercise session (42, 215). However, an increase in LDL particle size has been shown to follow completion of a marathon in men (129). The transient effects of exercise on LDL may not always benefit health, because circulating LDL may be more susceptible to harmful oxidation after very intense, long-duration exercise such as running a marathon (133). A single aerobic exercise session of sufficient volume can raise serum HDLc. The postexercise increase in HDLc is strikingly like what is generally attributed to long-term endurance exercise training. However, HDLc levels peak 24 to 48 h after exercise and last up to 72 h before returning to preexercise levels (54). More stable lipid and lipoprotein changes sometimes take several months to achieve (131). Acutely, an exercise energy expenditure threshold of about 350 kcal is enough to elevate HDLc in deconditioned individuals (41), but a caloric expenditure threshold of 800 kcal or more may be needed in well-conditioned individuals (60). The average density of HDL is reportedly reduced for up to 2 d after aerobic exercise (suggesting larger HDL particle size), a finding that provides further evidence that exercise acutely influences lipoprotein metabolism (42). A single endurance exercise session can lower blood triglyceride concentrations (176, 201), and this exercise effect is observed in apparently healthy normo- and hyperlipidemic men (24, 41, 42, 60, 66, 78). Similar to the HDLc responses to a single exercise session, the exercise effect on serum triglyceride is influenced by the training status of the subjects and volume of exercise performed. Regardless of mode or intensity, the exercise effect is Chapter 11 Hyperlipidemia and Dyslipidemia lost after about 48 to 72 h (54). Existing evidence also supports a relationship between preexercise triglyceride concentration and the magnitude of the postexercise change. In other words, people with elevated preexercise serum triglyceride concentrations exhibit the greatest postexercise reductions, while those with relatively low preexercise triglyceride concentrations show only modest or no change after exercise (42, 43, 78, 109). Genetic variations are known to play a role in triglyceride metabolism (108, 156); however, very little data exist on the transient lipid-altering effects of a single exercise session in individuals with genetically determined hypertriglyceridemia. Postprandial lipemia is lower in the hours after completion of aerobic exercise of sufficient volume (136, 142, 158) but is increased when exercise is withdrawn for several days (89). Together, these data suggest that the beneficial influence of exercise on circulating lipids and lipoproteins is an acute phenomenon that is lost rather quickly after cessation of exercise, even in the most highly trained individuals (95). The message for the clinical 185 exercise physiologist is that exercise must be repeated regularly to maintain the acute benefit. Resistance Exercise Resistance exercise appears to have a small beneficial influence on blood lipids that is somewhat similar to that reported for endurance exercise. Meta-analyses on up to 69 studies including up to 2,158 male and female adult participants provide evidence that regular resistance training modestly lowers total cholesterol, LDLc, nonHDLc, and triglyceride while increasing HDLc, as long as the training volume (resistance, repetitions, or sets, or a combination) increases progressively over the course of the exercise program (40, 114). The clinician should interpret these findings with caution and continue to review the literature in this area; relatively fewer calories are expended in resistance versus aerobic activity, and therefore resistance training per se may be less effective than endurance activities for modifying blood lipid levels (185). Research Focus Exercise and HDL Function Exercise is thought to enhance reverse cholesterol transport and increase HDLc. However, evidence provides insight on how aerobic exercise improves antioxidant properties of HDL and the role HDL plays in transporting lipid peroxides (197). The purpose of this investigation was to determine the influence of exercise on endogenous antioxidant potential and lipid oxidation in LDL and HDL fractions. Twenty-four male endurance runners underwent a progressive treadmill running protocol to exhaustion. Exercise was strenuous (between 20 and 22 min, with the last 6-8 min above the anaerobic threshold). Blood samples were obtained after standardized preexercise procedures and just before exercise, 15 min after exercise, and again at 90 min postexercise. Lipoproteins were separated from serum obtained from the blood samples. Lipids were extracted from the lipoprotein fractions, and measures of LDLc, HDLc, and oxidized lipids were estimated from standardized benchtop methods. Serum was measured for total antioxidant capacity, the HDL-associated antioxidant paraoxonase, and lipoprotein oxidation resistance. Greater HDLc and lower LDLc concentrations were observed within 90 min of completing the treadmill exercise. Total antioxidant capacity was enhanced along with HDL-associated paraoxonase. LDL lipid peroxides were reduced; however, HDL lipid peroxides increased after exercise. The lipid peroxide changes favored greater HDL peroxide transport. Based on Valimaki et al. (197). Exercise acutely increases the concentrations of oxidized HDL lipids while having an opposite effect on oxidized LDL lipid concentrations. The distribution shift is opposite of what occurs in the hours after eating a high-fat meal. Results from the serum analyses were interpreted to suggest that the increase in HDL lipid peroxides was not attributed to a transfer among LDL and HDL fractions. Rather, greater oxidized HDL lipids were thought to arise from enhanced HDL lipid peroxide clearing function, where products of lipid oxidation were scavenged by HDL at sites of formation and transported back to the liver. The reduced LDL lipid peroxide levels were not due to a decrease in LDLc, because the ratio of oxidized LDL lipid to LDLc decreased after exercise. The authors speculate that an increased HDL antioxidant capacity may have protected LDL lipids from oxidizing postexercise; however, this interpretation was not directly tested. This study offers strong evidence that exercise induces biochemical changes beyond altering lipoprotein lipid concentrations. Potentially harmful lipid peroxides in LDL are diminished, and HDL transport of lipid peroxides and antioxidant function are improved. The study highlights the key role exercise plays in modifying lipoprotein lipids and the inflammation and oxidative stress associated with lipid transport. 186 Davis, Grandjean, Crouse, and Durstine Clinical Exercise Bottom Line Dyslipidemia patients have an elevated risk of cardiovascular disease. Therefore, the patients themselves and professionals providing exercise testing and training should be keenly aware of ASCVD signs and symptoms. A dyslipidemic condition alone does not necessitate a change in exercise testing protocols or intensity for exercise training. Patients with dyslipidemia could have preexisting comorbidities, such as coronary artery disease, obesity, and diabetes, that would significantly change exercise testing or training protocols. Up to 25% of patients taking lipid-lowering medications, particularly statins and fibric acid derivatives, may experience some form of medication intolerance that includes muscle CONCLUSION Exercise is essential for management of hyperlipidemia and dyslipidemia, with proven benefits that likely extend to a reduction in mortality and morbidity. Transient improvements in some lipid fractions, such as HDL cholesterol and triglyceride, may occur with just one exercise session, while more stable changes sometimes take several months to achieve. A comprehensive lifestyle inflammation and muscle damage. Rhabdomyolysis, a severe side effect arising from medication-induced muscle damage, is characterized by the presence of myoglobin in the blood, reduced and dark urine output, and a general feeling of weakness. Since kidney damage may result, a patient experiencing these symptoms should immediately contact their physician. Flushing, sweating, and nausea are associated with niacin use. The clinical exercise physiologist should remind the patient to take this medication before going to sleep at night to avoid these unwanted side effects during the day and especially with exercise. The patient should be made aware of these side effects so they can distinguish between the effect of the drug and sensations they might experience during exercise. treatment approach should be of utmost priority to best attain targeted patient cholesterol goals. As such, in addition to exercise programming, better nutrition choices and body composition improvements are needed along with appropriate medications, when necessary. A multidisciplinary team approach may prove most beneficial for helping patients achieve long-term success in meeting stringent guidelines. Online Materials Visit HKPropel to access a link to the references, the case studies with discussion questions, and a quiz to help you review key concepts and test your understanding of the material covered in this chapter.