Lipid Metabolism Part 2 PDF
Document Details
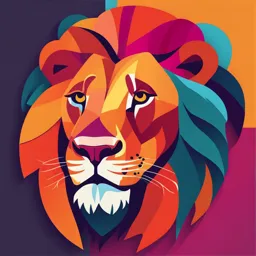
Uploaded by GenuineSwaneeWhistle
Universiti Putra Malaysia
2025
Nazhan Ilias
Tags
Summary
This document contains lecture notes on lipid metabolism, specifically portion 2, part of a veterinary biochemistry course for the 2024/2025 academic year at Universiti Putra Malaysia.
Full Transcript
Semester 1 2024/2025 VPP3021 VETERINARY BIOCHEMISTRY LIPID METABOLISM PART 2 Nazhan Ilias (DVM, PhD) Dept. of Veterinary Preclinical Sciences Faculty of Veterinary Medicine...
Semester 1 2024/2025 VPP3021 VETERINARY BIOCHEMISTRY LIPID METABOLISM PART 2 Nazhan Ilias (DVM, PhD) Dept. of Veterinary Preclinical Sciences Faculty of Veterinary Medicine Universiti Putra Malaysia [email protected] Digestion and Absorption of Dietary Lipids Ingestion of Dietary Lipids Dietary fats (mainly triglycerides, phospholipids, and cholesterol/cholesteryl esters) are consumed. Emulsification in the Small Intestine Bile salts emulsify dietary fats, breaking them into smaller droplets called micelles, making them more accessible for enzymatic digestion. Triglycerides will be digested with 3 different lipases (oral cavity: lingual lipase produced by sublingual and parotid salivary gland); gastric lipase produced by chief cells; and pancreatic lipase produced by pancreas). In the oral cavity and stomach, digestion of triglycerides is partial; majority take place in the small intestine. Cholesteryl esters will be converted to free cholesterol by cholesterol ester hydrolase in small intestine. Formation of Micelles Micelles are small, water-soluble lipid droplets consisting of: Fatty acids Monoglycerides Cholesterol Fat-soluble vitamins (A, D, E, K) Bile salts (to aid in solubilization of lipids). Micelles deliver lipid components to the intestinal epithelial cells (enterocytes) for absorption. Absorption in Enterocytes (Small Intestine Cells) Lipid Absorption Micelles deliver fatty acids, monoglycerides, and cholesterol to the brush border of the enterocytes. Lipids are absorbed into the enterocyte through simple diffusion except FC (NPC1L1). Reformation of Triglycerides Inside the enterocyte, fatty acids and monoglycerides are re- esterified to form triglycerides. Free cholesterol (cholesterol) re-esterified to form cholesteryl esters (CE) Formation of Chylomicrons The triglycerides are packaged along with cholesterol, phospholipids, and apolipoproteins (mainly apoB-48) into chylomicrons. Chylomicrons are large lipoproteins that transport dietary lipids from the intestines to other tissues. Transport in the Lymphatic System Chylomicrons are too large to enter the blood capillaries directly. They enter the lymphatic vessels through the lacteals (lymphatic vessels of the intestine). They travel through the lymphatic system and eventually enter the bloodstream at the left subclavian vein. Lipids to Peripheral Tissues (Chylomicron Remnants) Lipoprotein Lipase (LPL) In the bloodstream, lipoprotein lipase (LPL), located on the surface of endothelial cells (e.g., in muscle and adipose tissue), hydrolyzes the triglycerides in chylomicrons, releasing free fatty acids and glycerol. Free fatty acids are taken up by tissues such as muscle (for energy) and adipose tissue (for storage). Chylomicron remnants (with depleted triglyceride content) are left behind and contain mainly cholesterol and phospholipids. Chylomicron Remnants to Liver These remnants are taken up by the liver through specific receptors (e.g., LDL receptor-related protein (LRP). The liver can process these remnants, which are rich in cholesterol, for further use in bile acid synthesis or lipoprotein formation. Liver Processing and Formation of VLDL Liver Lipid Metabolism The liver synthesizes very low-density lipoproteins (VLDL), which are rich in triglycerides and cholesterol. VLDL is secreted into the bloodstream, where it serves to deliver triglycerides to peripheral tissues. VLDL to LDL Transformation VLDL particles circulate and interact with lipoprotein lipase (LPL), which hydrolyzes the triglycerides in VLDL. As triglycerides are removed, VLDL particles become intermediate-density lipoproteins (IDL). IDL is further processed in the liver to form low- density lipoproteins (LDL), which are primarily composed of cholesterol and phospholipids. LDL – Cholesterol Transport to Peripheral Tissues LDL Function LDL serves as the primary carrier of cholesterol to peripheral tissues (e.g., muscle, adrenal glands, and gonads). LDL receptors on the surface of these cells mediate endocytosis of LDL particles, facilitating the uptake of cholesterol for membrane synthesis, steroid hormone production, or bile acid formation. LDL Receptor-Mediated Endocytosis Once inside the cell, cholesterol is stored or used based on the cell’s needs. The LDL receptor is recycled back to the cell surface to bind more LDL. HDL – Reverse Cholesterol Transport Formation of HDL The liver and intestines secrete high-density lipoproteins (HDL), which are rich in apolipoprotein A-I (apoA-I) and contain some phospholipids and cholesterol. HDL is involved in the reverse cholesterol transport pathway, where it picks up excess cholesterol from peripheral tissues (e.g., macrophages in arterial walls) and returns it to the liver. Mature HDL Function In the liver, HDL can transfer cholesterol to VLDL or be processed further into bile acids for excretion. This process helps maintain cholesterol homeostasis and protects against atherosclerosis. Types of Lipoproteins Pandrangi, S. L., Chittineedi, P., Chikati, R., Mosquera, J. A. N., Llaguno, S. N. S., Mohiddin, G. J.,... & Maddu, N. (2022). Role of lipoproteins in the pathophysiology of breast cancer. Membranes, 12(5), 532. Triglyceride Overview Triglycerides (TGs) are the primary form of energy storage in animals. They consist of three fatty acid molecules esterified to a glycerol backbone. TG metabolism involves two key processes: Lipogenesis: The synthesis of triglycerides from smaller precursors. Lipolysis: The breakdown of triglycerides into glycerol and free fatty acids. (3-carbon alcohol) Glycerol 3 Fatty acid chains (saturated or unsaturated) Lipogenesis (Synthesis of Triglycerides) Lipogenesis is the process of synthesizing triglycerides and fatty acids. It predominantly occurs in the liver and adipose tissue during the fed state. STEPS IN LIPOGENESIS 1. Glucose Metabolism and Acetyl-CoA Production 2. Cytoplasmic Acetyl-CoA Formation Fatty Acid 3. Formation of Malonyl-CoA Synthesis 4. Fatty Acid Synthesis 5. Triglyceride Assembly Triglyceride assembly Fatty acids are activated by CoA to form fatty acyl-CoA. Glycerol-3-phosphate, derived from glucose metabolism, is esterified with fatty acyl-CoA to form triglycerides. TGs are stored in lipid droplets within adipocytes or exported as very low-density lipoproteins (VLDLs) from the liver. Regulation of Lipogenesis ACTIVATED BY Insulin (promotes ACC activity, glucose uptake, and glycerol-3-phosphate availability). High-carbohydrate diets (increase glycolysis and Acetyl-CoA availability). Citrate (allosteric activator of ACC). INHIBITED BY Glucagon and epinephrine (via cAMP-mediated phosphorylation and inhibition of ACC). Long-chain fatty acyl-CoA (feedback inhibition). Lipolysis (Breakdown of Triglycerides) Lipolysis is the process of hydrolyzing triglycerides into glycerol and free fatty acids (FFAs), which are used for energy during fasting or physical activity. This occurs primarily in adipose tissue. STEPS IN LIPOGENESIS 1. Activation of Hormone-Sensitive Lipase (HSL) 2. Triglyceride Hydrolysis 3. Release and Transport of FFAs 4. Glycerol Utilization 5. Fatty Acid Oxidation Lipolysis (Breakdown of Triglycerides) STEPS IN LIPOGENESIS Activation of Hormone-Sensitive Lipase (HSL) During fasting, glucagon and epinephrine bind to their receptors on adipocytes, increasing cyclic AMP (cAMP) levels. cAMP activates protein kinase A (PKA), which phosphorylates and activates HSL. Triglyceride Hydrolysis ATGL hydrolyzes triglycerides into diacylglycerol (DAG) and then HSL hydrolyzes DAG into monoacylglycerol (MAG). Monoacylglycerol lipase (MGL) hydrolyzes MAG into glycerol and FFAs. Lipolysis (Breakdown of Triglycerides) Release and Transport of FFAs FFAs diffuse out of adipocytes and bind to albumin in the bloodstream for transport to energy-demanding tissues (e.g., muscle and liver). Glycerol Utilization Glycerol is transported to the liver, where it enters gluconeogenesis or glycolysis after conversion to glycerol-3-phosphate by glycerol kinase. Fatty Acid Oxidation: FFAs are activated to fatty acyl-CoA and transported into mitochondria via the carnitine shuttle. In mitochondria, they undergo β-oxidation, generating Acetyl-CoA, NADH, and FADH2 for ATP production via the Krebs cycle and oxidative phosphorylation. Regulation of Lipolysis ACTIVATED BY Glucagon, epinephrine, norepinephrine (via cAMP/PKA pathway). Cortisol (enhances the expression of lipolytic enzymes). INHIBITED BY Insulin (stimulates phosphodiesterase activity to degrade cAMP, reducing HSL activation). High glucose and TG availability (signaling energy abundance). Cholesterol Overview Cholesterol is a vital lipid molecule essential for: 1. Membrane structure: Maintaining fluidity and stability of cell membranes. 2. Precursor roles: Synthesis of bile acids, steroid hormones, and vitamin D. 3. Lipoprotein transport: Transport of lipids in the bloodstream. Cholesterol synthesis occurs in most tissues, with the liver and intestines being the primary sites. It involves a multi-step enzymatic pathway starting from Acetyl-CoA and requires significant energy input from ATP and reducing power from NADPH. Cholesterol Cholesteryl esters Steps in Cholesterol Synthesis 1. Formation of Mevalonate 2. Conversion of Mevalonate to Isopentenyl Pyrophosphate (IPP) 3. Formation of Squalene 4. Conversion of Squalene to Lanosterol 5. Conversion of Lanosterol to Cholesterol Steps in Cholesterol Synthesis Formation of Mevalonate Acetyl-CoA Condensation: Two molecules of Acetyl-CoA condense to form acetoacetyl-CoA, catalyzed by thiolase. Acetoacetyl-CoA combines with another Acetyl-CoA to form 3- hydroxy-3-methylglutaryl-CoA (HMG-CoA), catalyzed by HMG-CoA synthase. HMG-CoA is reduced to mevalonate by HMG-CoA reductase, the rate- limiting enzyme of cholesterol synthesis. This step requires NADPH. Conversion of Mevalonate to Isopentenyl Pyrophosphate (IPP) Mevalonate undergoes a series of phosphorylations by mevalonate kinase and phosphomevalonate kinase to form 5- pyrophosphomevalonate. Decarboxylation of 5-pyrophosphomevalonate produces isopentenyl pyrophosphate (IPP), an activated isoprene unit. Steps in Cholesterol Synthesis Formation of Squalene IPP isomerizes to dimethylallyl pyrophosphate (DMAPP). IPP and DMAPP condense to form geranyl pyrophosphate (GPP). Another IPP molecule is added to GPP to form farnesyl pyrophosphate (FPP). Two FPP molecules condense to form squalene, catalyzed by squalene synthase. Conversion of Squalene to Lanosterol Squalene is first converted to squalene epoxide by squalene monooxygenase, requiring oxygen and NADPH. Squalene epoxide undergoes cyclization to form lanosterol, catalyzed by oxidosqualene cyclase. Steps in Cholesterol Synthesis Conversion of Lanosterol to Cholesterol Lanosterol is converted to cholesterol through a series of 19 additional steps, involving demethylation, reduction, and isomerization reactions. Regulation of Cholesterol Synthesis KEY REGULATORY MECHANISM: HMG-COA REDUCTASE 1. Feedback Inhibition 2. Hormonal Regulation 3. Sterol Regulatory Element-Binding Proteins (SREBPs) 4. Phosphorylation and Dephosphorylation CASE 1: Severe Spontaneous Atherosclerosis in cats Clinical Presentation: Two adult Korat cats exhibited progressive heart failure symptoms, ultimately leading to their deaths. Pathological Findings: Post-mortem examinations revealed severe atherosclerotic lesions in large and medium-sized arteries. These lesions featured fibrous caps and lipid cores with substantial accumulations of cholesterol crystals, closely mirroring advanced human atherosclerosis. Absence of Predisposing Factors: Neither cat had underlying diseases or medical treatments that could have predisposed them to atherosclerosis, suggesting a potential genetic predisposition within this breed. Implications for Research: The findings indicate that Korat cats may serve as a valuable model for studying human atherosclerosis, given the similarities in disease manifestation. Atherosclerosis Lipid Metabolism Cats have a distinct lipid metabolism compared to humans. They tend to maintain lower plasma cholesterol levels and a different lipoprotein profile that makes them less prone to cholesterol deposition in arterial walls. Dietary Habits Cats are obligate carnivores, and their natural diet (rich in protein and low in carbohydrates) does not favor the development of the lipid abnormalities often associated with atherosclerosis in humans. Physiological Factors Cats have fewer pro-inflammatory states that could exacerbate endothelial dysfunction, which is a key event in atherosclerosis and their arterial walls may have structural or functional adaptations that provide resistance to plaque formation. Genetic Resistance Cats may have inherent genetic factors that protect against the development of atherosclerotic plaques. For example, their natural lipid-handling mechanisms might reduce the likelihood of cholesterol accumulation in the arteries. Lifespan and Disease Development Atherosclerosis is often a chronic disease that develops over many years. The shorter lifespans of cats compared to humans might mean they do not live long enough for significant atherosclerotic disease to manifest. Atherosclerosis Chronic condition where arteries become narrowed and stiff due to the buildup of fatty deposits (plaques) on their inner walls. These plaques consist of cholesterol, lipids, calcium, and cellular debris. Over time, they can restrict blood flow, weaken the arterial wall, or lead to complications such as blood clots, heart attack, or stroke. It is primarily driven by inflammation, high cholesterol, and damage to the arterial endothelium. Nazhan own work (2023) created with BioRender.com. "Seek knowledge from the cradle to the grave"