6th Year Neurology Teaching Course 2018/2019 Lesson 1 PDF
Document Details
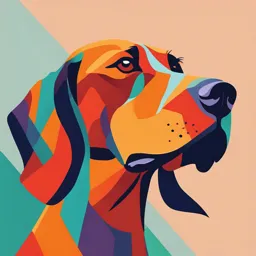
Uploaded by SmarterConstellation1885
Port Said Faculty of Medicine
2018
Dr. Saad Shawki Elsherifi
Tags
Summary
This document looks like a lecture notes for a 6th-year neurology course in the Port-Said Faculty of Medicine, 2018-19. It covers topics like neuroanatomy, divisions of the nervous system, neurophysiology, and basic neurotransmitters.
Full Transcript
Port-Said Faculty of Medicine Neuropsychiatry Department 6th year Neurology Teaching Course 2018/2019 Lesson 1 INTRODUCTION (Functional Neuroanatomy) by Dr. Saad Shawki Elsherifi Lect...
Port-Said Faculty of Medicine Neuropsychiatry Department 6th year Neurology Teaching Course 2018/2019 Lesson 1 INTRODUCTION (Functional Neuroanatomy) by Dr. Saad Shawki Elsherifi Lecturer of Neurology 1 INTRODUCTION Skeleton Of Lecture - Overview of neuroanatomy - Divisions of nervous system (CNS &PNS) - Ascending (sensory) & descending (motor) tracts - Overview of neurophysiology - Functions of different parts of nervous system - Basic neurotransmitters Introduction : The human nervous system consists of numerous components that are diverse in structure and function. Yet they function together, and in harmony, for the well being of the body as a whole. Its interconnected networks perform processing that is simultaneously local and distributed, serial and parallel, hierarchical and global. - Accordingly, structures of the nervous system can be described on multiple levels: 1- macroscopic brain divisions; 2- connecting pathways 3- individual brain cells; 4- receptors & neurotransmitters. 2 1. Macroscopic Divisions of nervous system Developmental hint The developing brain has three main divisions: the forebrain→ prosencephalon, the midbrain→ mesencephalon; and the hindbrain→ rhombencephalon. * The forebrain is the largest part of the nervous system in humans, and it is further subdivided into the telencephalon and diencephalon. The telencephalon (meaning “end brain” in Greek) is made up of the cerebral hemispheres and includes structures such as the cerebral cortex, white matter (WM), and basal ganglia (BG). The diencephalon is composed of the thalamus, hypothalamus, and associated structures. * The midbrain is a relatively short and narrow region connecting the forebrain and hindbrain. * The hindbrain is composed of the pons and cerebellum (metencephalon) together with the medulla (myelencephalon) The midbrain, pons, and medulla together form a connection between the forebrain and the spinal cord. Since the forebrain sits on top of the midbrain, pons, and medulla, almost like a cauliflower on its stalk, these structures are often referred to as the brainstem. The brainstem is the most evolutionarily ancient part of the human brain and is the part that most closely resembles the brains of fish and reptiles. It controls many of the most basic bodily functions necessary for survival, such as respiration, blood pressure, and heart rate. 3 2. Microscopic and Neurochemical Organization of the Nervous System Microscopically, the nervous system is composed of : nerve cells, or neurons, and support cells called glial cells (or simply glia). Neurons are the basic units of signaling in the nervous system, although glial cells may contribute as well. A typical neuron has a cell body containing the nucleus, relatively short processes called dendrites, which receive most inputs to the cell, and long processes called axons, which carry most outputs. Most mammalian neurons are multipolar meaning that they have several dendrites as well as several axons. Often, a single axon arising from the cell body will travel for a distance, and then one or several axon collaterals branch off the main axon to reach different targets. Some neurons are bipolar, with a single dendrite and a single axon arising from the cell body. Bipolar cells are often sensory neurons, such as those involved in vision or olfaction. Some bipolar neurons are called pseudo- unipolar, since their processes are initially fused and then split to produce two long axons. An example is dorsal root ganglion sensory neurons. Unipolar neurons, in which both axons and dendrites arise from a single process coming off the cell body, occur mainly in invertebrates. 4 Communication between neurons takes place mainly at specialized regions called synapses. Classically, synapses carry information from the axon terminals of one neuron to the dendrites of the next neuron. However, there are also axo-axonic and dendro-dendritic synapses, and some forms of communication can even occur in reverse, traveling from dendrites back to axons. At chemical synapses, chemical neurotransmitter molecules, stored mainly in synaptic vesicles, are released from presynaptic terminals of the neuron. They then bind to neurotransmitter receptors on the postsynaptic neuron, giving rise to either excitation or inhibition of the postsynaptic neuron. In some cases, communication also takes place at electrical synapses where direct electrical coupling of neurons occurs through specialized junctions. Neurons are electrically and chemically active. When excitatory synaptic inputs combine with endogenous trans-membrane currents to sufficiently excite a neuron, a transient voltage change called an action potential occurs, lasting about 1 millisecond. Action potentials can travel rapidly throughout the length of a neuron, propagating at rates of up to about 60 meters per second along the cell membrane. Classically, action potentials travel from the dendritic end 5 of the neuron along its axon to reach presynaptic terminals, where communication can occur with the next neuron. Action potentials trigger release of neurotransmitter molecules from synaptic vesicles, allowing chemical communication with the postsynaptic cell. Axons are often insulated by specialized glial cells that form a lipid myelin sheath, thereby speeding the rate of action potential conduction. The myelin-forming glial cells in the CNS are oligodendrocytes; in the PNS they are Schwann cells. Voltage-gated ion channels are concentrated in short, exposed segments of the axon called nodes of Ranvier. Conduction of action potentials from node to node occurs rapidly by a process called saltatory conduction. Neurons form the functional cellular units responsible for communication, and throughout the nervous system, they are characterized by their distinctive size and shapes (e.g., bipolar, unipolar, multipolar). Supporting cells include the neuroglia (e.g., astrocytes, oligodendrocytes), satellite cells, and other specialized cells that optimize neuronal function, provide maintenance functions, or protect the nervous system. 6 Chemical neurotransmitters have two general types of functions. One is to mediate rapid communication between neurons through fast excitatory or inhibitory electrical events known as excitatory postsynaptic potentials(EPSPs) and inhibitory postsynaptic potentials (IPSPs). Fast EPSPs and IPSPs occur on the timescale of tens of milliseconds and rapidly move the membrane voltage of the postsynaptic neuron between states more or less likely to fire an action potential. The postsynaptic neuron summates EPSPs and IPSPs arising from many presynaptic inputs. The second function of chemical neurotransmitters is neuromodulation, generally occurring over slower time scales. Neuromodulation includes a broad range of cellular mechanisms involving signaling cascades that regulate synaptic transmission, neuronal growth, and other functions. Neuromodulation can either facilitate or inhibit the subsequent signaling properties of the neuron. MORPHOLOGY OF SYNAPSES: Neurons communicate with each other and with effector targets at specialized regions called synapses. The top figure shows a typical motor neuron that receives numerous synaptic contacts on its cell body and associated dendrites. Incoming axons lose their myelin sheaths, exhibit extensive branching, and terminate as synaptic boutons (synaptic terminals or knobs) on the motor neuron. The lower figure shows an enlargement of one such synaptic bouton. Chemical neurotransmitters are contained in synaptic vesicles, which can fuse with the presynaptic membrane, release the transmitters into the synaptic cleft, and then bind to receptors situated in the postsynaptic membrane. This synaptic transmission results in excitatory, inhibitory, or modulatory effects on the target cell. Some of the more important and common neurotransmitters are summarized in Table 2.2. Neurotransmitters can be small molecules such as acetylcholine, amino acids like glutamate, or larger molecules such as peptides. Depending on the specific receptors 7 present, neurotransmitters can mediate fast neurotransmission through EPSPs or IPSPs, or they may have facilitatory or inhibitory neuromodulatory effects on neuronal signaling. Some neurotransmitters have different actions at different synapses or even at the same synapse when a mixture of receptor types is present. In addition, more than one type of neurotransmitter molecule is often released, even at a single synapse. In the CNS, the most common excitatory neurotransmitter is glutamate; the most common inhibitory neurotransmitter is GABA (gamma-aminobutyric acid).In the PNS, acetylcholine is the main transmitter at neuromuscular junctions, and both acetylcholine and norepinephrine are important in the autonomic nervous system. 8 3. CNS Gray Matter and White Matter; PNS Ganglia and Nerves Areas of the CNS made up mainly of myelinated axons are called white matter. Areas made up mainly of cell bodies are called gray matter. Most of the local synaptic communication between neurons in the CNS occurs in the gray matter, while axons in the white matter transmit signals over greater distances. The surface of the cerebral hemispheres is covered by a unique mantle of gray matter called the cerebral cortex, which is far more developed in higher mammals than in other species. Beneath this lies the white matter, which conveys signals to and from the cortex. Gray matter is also found in large clusters of cells called nuclei located deep within the cerebral hemispheres and brainstem. Examples include the basal ganglia, thalamus,and cranial nerve nuclei. In the cerebral hemispheres the gray matter cortex is outside, while the white matter is inside. In the spinal cord the opposite is true: White matter pathways lie on the outside, while the gray matter is in the center. In the brainstem, gray matter and white matter regions are found both on the inside and on the outside, although most of the outside surface is white matter. Several different names with similar meaning are used for white matter pathways in the CNS, including tract, fascicle, lemniscus, and bundle. In general, pathways carrying signals toward a structure are called afferent, while those carrying signals away from a structure are called efferent. A white matter pathway that connects structures on the right and left sides of the CNS is called a commissure. Axons in the PNS form bundles called peripheral nerves, or simply nerves. Clusters of cell bodies in the PNS are referred to as ganglia. 9 4. Spinal Cord and Peripheral Nervous System The human nervous system develops in segments similar to those of simpler animals, such as segmented worms. As already described, the segments in the head expand and fuse together, forming the cerebral hemispheres and brainstem. Twelve pairs of cranial nerves exit these segments. The spinal nerves arise from the segments of the spinal cord. Each segment gives rise to both sensory and motor nerve roots on each side of the body. Throughout the nervous system, motor systems tend to be more ventral, or anterior, and sensory systems more dorsal, or posterior. The same holds true for the spinal cord. Thus, dorsal nerve roots convey mainly afferent sensory information into the dorsal spinal cord, while ventral nerve roots carry mainly efferent motor signals from the ventral spinal cord to the periphery. The segments and nerve roots of the spinal cord are named according to the level at which they exit the bony vertebral canal. Thus, there are cervical, thoracic, lumbar, and sacral nerve roots. During development, the bony vertebral canal increases in length faster than the spinal cord. Therefore, the spinal cord ends at the level of the first or second lumbar vertebral bones (L1 or L2). Below this the spinal canal contains a collection of nerve roots known as the cauda equine (Latin for “horse’s tail”), which continue down to their exit points. The sensory and motor nerve roots join together a short distance outside the spinal cord and form a mixed sensory and motor spinal nerve. Control of the arms and legs requires much more signal flow than does control of the chest and abdomen. Thus, the nerves controlling the extremities give rise to elaborate meshworks referred to as the brachial plexus for the arms and the lumbosacral plexus for the legs. In addition, the spinal cord contains a relatively increased amount of gray matter in these segments, causing the overall thickness of the cord to be greater. These regions of the cord are called the cervical enlargement and the lumbosacral enlargement, respectively. 10 11 12 5- Autonomic Nervous System In addition to the sensory and motor pathways already described, the PNS includes some specialized neurons that are involved in controlling such automatic functions as heart rate, peristalsis, sweating, and smooth muscle contraction in the walls of blood vessels, bronchi, sex organs, the pupils, and so on. These neurons are part of the autonomic nervous system. The autonomic nervous system has two major divisions): The sympathetic division arises from thoracic and lumbar spinal levels T1 to L3 (the thoracolumbar division). It releases the neurotransmitter norepinephrine onto end organs and is involved in such “fight or flight” functions as increased heart rate and blood pressure, bronchodilation, and increased pupil size. The parasympathetic division, in contrast, arises from the cranial nerves and from sacral spinal levels S2 to S4 (the craniosacral division).It releases acetylcholine onto end organs and is involved in more sedentary functions "rest or digest" , such as increasing gastric secretions and peristalsis, slowing the heart rate, and decreasing pupil size. The sympathetic and parasympathetic pathways are controlled by higher centers in the hypothalamus and limbic system as well as by afferent sensory information from the periphery. The enteric nervous system is considered a third autonomic division and consists of a neural plexus, lying within the walls of the gut, that is involved in controlling peristalsis and gastrointestinal secretions. 13 The autonomic nervous system (ANS) is a two-neuron chain, with the preganglionic neuron arising from the central nervous system and synapsing on a postganglionic neuron located in a peripheral autonomic ganglion. Acetylcholine is the neurotransmitter in both the sympathetic and parasympathetic ganglia. The parasympathetic division of the ANS releases acetylcholine at its postganglionic synapses and is characterized as having cholinergic (C) effects, whereas the sympathetic division releases predominantly noradrenaline (norepinephrine) at its postganglionic synapses, causing adrenergic (A) effects (except on sweat glands, where acetylcholine is released). Although acetylcholine and noradrenaline are the chief transmitter substances, other neuroactive peptides often are colocalized with them and include such substances as gammaaminobutyric acid (GABA), substance P, enkephalins, histamine, glutamic acid, neuropeptide Y, and others. 14 6. Cerebral Cortex: Basic Organization and Primary Sensory and Motor Areas The cerebral cortex is not a smooth sheet, but rather has numerous infoldings or crevices called sulci. The bumps or ridges of cortex that rise up between the sulci are called gyri. The cerebral hemispheres have four major lobes: the frontal, temporal, parietal,and occipital. 6.1 Lobes of the Cerebral Hemispheres The frontal lobes are, appropriately, in the front of the brain and extend back to the central sulcus of Rolando. The frontal lobes are separated inferiorly and laterally from the temporal lobes by an especially deep sulcus called the Sylvian fissure, or lateral fissure. (The term fissure is sometimes used to refer to deep sulci.) The parietal lobes are bounded anteriorly by the central sulcus but have no sharp demarcation from the temporal lobes or the occipital lobes when viewed from the lateral side of the brain. When viewed from the medial aspect, the parieto-occipital sulcus can be seen more easily, separating the parietal from the occipital lobes. 15 In addition to these four major lobes, an additional region of cerebral cortex called the insular cortex lies buried within the depths of the Sylvian fissure. The insula is covered by a lip of frontal cortex anteriorly and parietal cortex posteriorly, called the frontal operculum and parietal operculum, respectively (operculum means “covering” or “lid” in Latin). The limbic cortex was formerly referred to as the “limbic lobe,” but this terminology is no longer generally used. The two cerebral hemispheres are separated in the midline by the interhemispheric fissure, also known as the sagittal or longitudinal fissure. A large, C-shaped band of white matter called the corpus callosum (meaning “hard body”) connects both homologous and heterologous areas in the two hemispheres. 16 6.2 Surface Anatomy of the Cerebral Hemispheres in Detail Although there is some variability, the sulci and gyri of the cerebral hemispheres form certain fairly consistent patterns. On the lateral surface, the frontal lobe is bounded posteriorly by the central sulcus, as already noted. The gyrus running in front of the central sulcus is called the precentral gyrus. The remainder of the lateral frontal surface is divided into the superior, middle, and inferior frontal gyri by the superior and inferior frontal sulci. Similarly, the lateral temporal lobe is divided into superior, middle, and inferior temporal gyri by the superior and middle temporal sulci. The most anterior portion of the parietal lobe is the postcentral gyrus, lying just behind the central sulcus. The intraparietal sulcus divides the superior parietal lobule from the inferior parietal lobule. The inferior parietal lobule consists of the supramarginal gyrus (surrounding the end of the Sylvian fissure) and the angular gyrus (surrounding the end of the superior temporal gyrus) On the medial surface, the corpus callosum is clearly visible, consisting of the rostrum, genu, body, and splenium. The amygdaloid gyrus (cingulum means “girdle” or “belt”) surrounds the corpus callosum, running from the paraterminal gyrus anteriorly to the isthmus posteriorly. The central sulcus does not usually extend onto the medial surface, but the region surrounding it is called the paracentral lobule. The portion of the medial occipital lobe below the calcarine fissure is called the amygdal (meaning “little tongue”), while the portion above the calcarine fissure is called the cuneus (meaning “wedge”). Just in front of the cuneus, the medial parietal lobe is called the precuneus. On the inferior surface, the orbital frontal gyri can be seen, which lay on top of the orbital ridges of the eye. More medially, the olfactory sulcus (containing the olfactory bulb) separates the orbital frontal gyri from the gyrus rectus (meaning “straight gyrus”). On the inferior surface of the temporal lobe, the inferior temporal sulcus separates the inferior temporal gyrus from the occipitotemporal, or fusiform, gyri. More medially, the collateral sulcus, continuing anteriorly as the rhinal sulcus, separates the fusiform gyri from the parahippocampal gyrus. Finally, on the superior surface, many of the same landmarks seen on the lateral surface are again visible. 17 6.3 Primary Sensory and Motor Areas The primary motor cortex lies in the precentral gyrus in the frontal lobe. This area controls movement of the opposite side of the body. The primary somato-sensory cortex is in the postcentral gyrus in the parietal lobe and is involved in sensation for the opposite side of the body. Note that the precentral and postcentral gyri are separated by the central sulcus and that (as in the spinal cord) motor areas lie anterior to somatosensory areas. The primary visual cortexis in the occipital lobes along the banks of a deep sulcus called the calcarine fissure. The primary auditory cortex is composed of the transverse gyri of Heschl, which are two fingerlike gyri that lie inside the Sylvian fissure on the superior surface of each temporal lobe. Sensory and motor pathways are usually topographically organized. These somatotopic maps on the cortex are sometimes called the motor or sensory homunculus (“little man”). Interestingly, the primary somatosensory cortex and primary motor cortex represent sensation and movement, respectively, for the opposite side of the body. Knowledge of the levels at which the somatosensory and motor pathways cross over in the nervous system can be helpful for clinical neuroanatomical localization. The primary visual cortex represents visual inputs from the opposite visual field. Thus, the left half of the visual field for each eye is mapped to the right primary visual cortex. Information reaching the primary auditory cortex is less lateralized and represents more of a mixture of inputs from both ears (the input from the opposite ear is slightly stronger, but this is usually not clinically detectable). 18 6.4 Cell Layers and Regional Classification of the Cerebral Cortex The majority of the cerebral cortex is composed of neocortex, which has six cell layers, labeled I through VI, counting from the surface inward. In a few regions associated with the limbic system, less than six layers are present. Neocortical circuitry is quite complex, and we will describe only a few of the major connections of each layer here. Layer Icontains mainly dendrites of neurons from deeper layers as well as axons. Layers II and III contain neurons that project mainly to other areas of cortex. Layer IV receives the majority of inputs from the thalamus. Layer V projects mostly to subcortical structures other than the thalamus, such as the brainstem, spinal cord, and basal ganglia. Layer VI projects primarily to the thalamus. In addition to these connections, numerous other circuits exist between and within the cortical layers that are beyond the scope of this discussion. The relative thickness of the cell layers varies according to the main function of that area of cortex. For example, the primary motor cortex has large efferent projections to the brainstem and spinal cord, which control movement. It receives relatively little direct sensory information from thalamic relay centers. Therefore, in the primary motor cortex, layer V is thicker and has many more cell bodies than layer IV. The opposite holds for primary visual cortex, where layer IV contains many cell bodies and layer V is relatively cell poor. Association cortex has a cellular structure that is intermediate between these types. A variety of classification schemes exist for different regions of the cerebral cortex based on microscopic appearance and function. The most widely known of these was published by Korbinian Brodmann in 1909. Based on his studies conducted with the microscope, Brodmann parceled the cortex into 52 cytoarchitectonic areas, each assigned a number corresponding to the order in which he prepared the slides. 19 7. Motor System Motor control involves a delicate balance between multiple parallel pathways and recurrent feedback loops. We will now provide an overview of the most important motor pathways and of the cerebellum and basal ganglia, which are major feedback systems. 7.1 Main Motor Pathways The most important motor pathway in humans is the corticospinal tract. The corticospinal tract begins mainly in the primary motor cortex, where neuron cell bodies project via axons down through the cerebral white matter and brainstem to reach the spinal cord. The corticospinal tract is sometimes called the pyramidal tract because of its triangular shape in the medulla. The majority of fibers in the corticospinal tract (about 85%) cross over to control movement of the opposite side of the body. This crossing over, known as the pyramidal decussation, occurs at the junction between the medulla and the spinal cord. Thus, lesions occurring above the pyramidal decussation produce contralateral (opposite-sided) weakness with respect to the lesion, while lesions below the pyramidal decussation will produce ipsilateral (same-sided) weakness. There are several other descending motor pathways that exist in addition to the corticospinal tract e.g. rubrospinal. Tectospinal, vestibulospinal…etc. Motor neurons that project from the cortex down to the spinal cord or brainstem are referred to as upper motor neurons (UMNs). UMNs form synapses onto the lower motor neurons(LMNs), which are located in the anterior horns of the central gray matter of the spinal cord or in brainstem motor nuclei. The axons of LMNs project out of the CNS via the anterior spinal roots or via the cranial nerves to finally reach muscle cells in the periphery. 7.2 Cerebellum and Basal Ganglia The motor system is called upon to perform many delicate and complicated tasks. Therefore, in order to refine the output of the motor system, multiple feedback systems are employed, including the cerebellum and basal ganglia.These two systems do not 20 themselves project directly to the LMNs. Instead, the cerebellum and basal ganglia act by modulating the output of the corticospinal and other descending motor systems. The cerebellum and basal ganglia both receive major inputs from the motor cortex. The cerebellum also receives significant inputs from the brainstem and spinal cord. The cerebellum and basal ganglia, in turn, project back to the motor cortex via the thalamus. 21 8. Somatosensory System Sensation from the body is conveyed by parallel pathways mediating different sensory modalities that travel to the central nervous system. We will now review the most important sensory pathways and introduce the thalamus, a major relay center for signals of all kinds (sensory and other) that travel to the cerebral cortex. 8.1 Main Somatosensory Pathways Somatic sensation refers to the conscious perceptions of touch, pain, temperature, vibration, and proprioception (limb or joint position sense). There are two main pathways in the spinal cord for somatic sensation: 1. The posterior column pathways : convey proprioception, vibration sense, and fine, discriminative touch. 2. The anterolateral pathways : convey pain, temperature sense, and crude touch. Some aspects of touch sensation are carried by both pathways, so touch sensation is not eliminated in isolated lesions of either pathway. Note that the primary sensory neuron cell bodies are located outside the CNS in the dorsal root ganglia and that they have bifurcating axons, with one long process extending to the periphery and one into the spinal cord. For localizing clinical lesions, the knowledge of where in the CNS the two main sensory pathways cross over is equally as important as the knowledge that the corticospinal tract crosses over at the pyramidal decussation. Therefore, we will briefly outline the two main sensory pathways here. POSTERIOR COLUMN PATHWAY Primary sensory neuron axons carrying information about proprioception, vibration sense, and fine touch enter first the spinal cord via the dorsal roots and then the ipsilateral white matter dorsal (posterior) columns to ascend all the way to the dorsal column nuclei in the medulla. Here they make synapses onto the secondary sensory neurons, which send out axons that cross 22 over to the other side of the medulla. These axons continue to ascend, now on the contralateral side, and synapse in the thalamus, and from there neurons project to the primary somatosensory cortex in the postcentral gyrus. ANTEROLATERAL PATHWAY Primary sensory neurons carrying information about pain, temperature sense, and crude touch also enter the spinal cord via the dorsal roots. However, these axons make their first synapses immediately in the gray matter of the spinal cord. Axons from the secondary sensory neurons cross over to the other side of the spinal cord and ascend in the anterolateral white matter, forming the spinothalamic tract. After synapsing in the thalamus, the pathway again continues to the primary somatosensory cortex. 23 8.2 Thalamus The thalamus is an important relay center. Nearly all pathways that project to the cerebral cortex do so after synapsing in the thalamus. The thalami are gray matter structures located deep within the cerebral white matter just above the brainstem and behind the basal ganglia. They are shaped somewhat like eggs, with their posterior ends angled outward, together forming an inverted V in horizontal sections. The thalamus consists of multiple nuclei. Each sensory modality, including vision, hearing, taste, and somatic sensation, has a different nuclear area where synapses occur before the information is relayed to the cortex (olfactory inputs are an exception and do not pass directly through the thalamus). Non-sensory pathways also relay in the thalamus. For example, there are thalamic nuclei that process information coming from the basal ganglia, cerebellum, limbic pathways, and brainstem reticular formation on the way to the cortex. An important feature of thalamic circuits is the reciprocal nature of cortical–thalamic connections. Thus, virtually all cortical regions project strongly via layer VI back to the thalamic areas from which their major inputs arise. As we discussed earlier in this chapter, the thalamus, together with the hypothalamus and epithalamus, form the diencephalon. The hypothalamus is an important region for control of autonomic, neuro-endocrine, limbic, and other circuits. The epithalamus encompasses several small nuclei, including the pineal body, habenula, and parts of the pretectum. 24 9. Internal Capsule We have seen that a large number of nerve fibres interconnect the cerebral cortex with centres in the brainstem and spinal cord, and with the thalamus. Most of these fibres pass through the interval between the thalamus and caudate nucleus medially, and the lentiform nucleus laterally. This region is called the internal capsule. Above, the internal capsule is continuous with the corona radiata; and, below, with the crus cerebri (of the midbrain). The internal capsule may be divided into the following parts : (a) The anterior limb lies between the caudate nucleus medially, and the anterior part of the lentiform nucleus laterally. (b) The posterior limb lies between the thalamus medially, and the posterior part of the lentiform nucleus on the lateral side. (c) The genu ; the angle between the anterior and posterior limbs of the internal capsule (d) retrolentiform part, Some fibres of the internal capsule lie behind the posterior end of the lentiform nucleus. (e) sublentiform part, Some other fibres pass below the lentiform. 25 26 10. Blood Brain Barrier The blood-brain barrier (BBB) is the cellular interface between the blood and the central nervous system (CNS; brain and spinal cord). It serves to maintain the interstitial fluid environment to ensure optimal functionality of the neurons. This barrier consists of the capillary endothelial cells with an elaborate network of tight junctions and astrocytic foot processes (It has been estimated that these processes cover about eighty five percent of the capillary surface) that abut the endothelium and its basement membrane. The movement of large molecules and other substances (including many drugs) from the blood to the interstitial space of the CNS is restricted by the BBB. Some areas of the brain (and related structures) appear to be devoid of a blood-brain barrier. These include the pineal body, the hypophysis cerebri, the choroid plexus, the median eminence (hypothalamus) and some specialised areas of ependyma in the walls of the third and fourth ventricles. The blood brain barrier can break down following ischaemia or infection in the brain. 11. Brainstem and Cranial Nerves The brainstem is composed of the midbrain, pons, and medulla. It is connected to the diencephalon rostrally, the cerebellum dorsally, and the spinal cord caudally. Most of 27 the cranial nerves arise from the brainstem. The cranial nerves are analogous in some ways to the spinal nerves, having both sensory and motor functions. However, they also carry out more specialized functions relating to the organs of the head. Examination of the cranial nerves provides crucial information about the functioning of the nervous system. In addition to the cranial nerve nuclei and pathways, the brainstem is tightly packed with numerous other important nuclei and white matter tracts. All information passing between the cerebral hemispheres and the spinal cord must pass through the brainstem. Therefore, lesions in the brainstem can have a devastating effect on sensory and motor function. In addition, the brainstem contains nuclei that play important roles in the motor system; nuclei that produce nausea and vomiting in response to certain chemicals; modulatory nuclei containing the neurotransmitters norepinephrine, serotonin, dopamine, and acetylcholine, which project widely throughout the CNS; nuclear areas involved in pain modulation; and nuclei controlling heart rate, blood pressure, and respiration, among other functions. An important region of the brainstem that contains many of these nuclei is the reticular formation. Named for the network-like appearance of its fibers in histological sections, the reticular formation extends throughout the central portions of the brainstem from the medulla to the midbrain. The more caudal portions of the reticular formation in the medulla and lower pons tend to be involved mainly in motor and autonomic functions. The rostral reticular formation in the upper pons and midbrain plays an important role in regulating the level of consciousness, influencing higher areas through modulation of thalamic and cortical activity. Thus, lesions that affect the ponto-mesencephalic reticular formation can cause lethargy and coma. Cortical, thalamic, and other forebrain networks are also important in maintaining consciousness. Therefore, the level of consciousness can also be impaired in bilateral lesions of the thalami or in bilateral (or large unilateral) lesions of the cerebral hemispheres. Note that mass lesions above the brainstem often cause impaired consciousness indirectly when they exert pressure on the brainstem through mass effect, thus distorting or compressing the reticular formation and thalamus. 28 12. Limbic System Several structures in the brain are referred to collectively as the limbic system because they are located near the medial edge or fringe (limbus in Latin) of the cerebral cortex. These structures have evolved, from a system devoted mainly to olfaction in simpler animals, to perform diverse functions, including the regulation of emotions, memory, appetitive drives, and autonomic and neuro-endocrine control. The limbic system includes certain cortical areas located in the medial and anterior temporal lobes, anterior insula, inferior medial frontal lobes, and amygdaloid gyri. It also includes deeper structures such as the hippocampal formation and the amygdale, located within the medial temporal lobes, several nuclei in the medial thalamus, hypothalamus, basal ganglia, septal area, and brainstem. These areas are interconnected by a variety of pathways, including the fornix—a paired, arch-shaped white matter structure that connects the hippocampal formation to the hypothalamus and septal nuclei. Lesions in the limbic system can cause deficits in the consolidation of immediate recall into longer-term memories. Thus, patients with lesions in these areas may have no trouble recalling remote events but have difficulty forming new memories. In addition, limbic dysfunction can cause behavioral changes and may underlie a number of psychiatric disordersand epileptic 29 seizures that may begin with emotions such as fear, memory distortions such as déjà vu, or olfactory hallucinations. 13. Association Cortex In addition to the primary motor and sensory areas described earlier, the cerebral cortex contains a large amount of association cortex, which carries out higher-order information processing. In unimodal association cortex, higher-order processing takes place mostly for a single sensory or motor modality. Unimodal association cortex is usually located adjacent to a primary motor or sensory area; for example, unimodal visual association cortex is located adjacent to the primary visual cortex, and unimodal motor association cortex (premotor cortex and supplementary motor area) is located adjacent to the primary motor cortex. Heteromodal association cortex is involved in integrating functions from multiple sensory and/or motor modalities. Language is usually perceived first by the primary auditory cortex in the superior temporal lobe when we are listening to speech or by the primary visual cortex in the occipital lobes when 30 we are reading. From here, cortical–cortical association fibers convey information to Wernicke’s area in the dominant (usually left) hemisphere. Lesions in Wernicke’s area cause deficits in language comprehension, also sometimes called receptive or sensory aphasia, or Wernicke’s aphasia. Broca’s area is located in the frontal lobe, also in the left hemisphere, adjacent to the areas of primary motor cortex involved in moving the lips, tongue, face, and larynx. Lesions in Broca’s area cause deficits in the production of language, with relative sparing of language comprehension. This is called expressive or motor aphasia, or Broca’s aphasia. * The parietal lobe is divided by the intraparietal sulcus into a superior and inferior parietal lobule. Lesions in the inferior parietal lobule in the left hemisphere can produce an interesting constellation of abnormalities, including difficulty with calculations, right–left confusion, inability to identify fingers by name (finger agnosia), and difficulties with written language. This group of abnormalities is called Gerstmann’s syndrome. Performance of complex motor tasks, such as brushing one’s teeth or throwing a baseball, requires higher-order planning before the primary motor cortex can be activated. Motor planning appears to be distributed in many different areas of cortex. Thus, diffuse lesions of the cortex, or sometimes more focal lesions affecting the frontal or left parietal lobe, can produce abnormalities in motor conceptualization, planning, and execution called apraxia. The parietal lobes also play an important role in spatial awareness. Thus, lesions in the parietal lobe, especially in the nondominant (usually right) hemisphere, often cause a distortion of perceived space and neglect of the contralateral side. For example, right parietal lesions can cause left hemineglect. With this syndrome, patients will often ignore objects in their left visual field, but they may see them if their attention is strongly drawn to that side. They may be unaware of any weakness or other deficits on that side( anosognosia). Patients may also display a phenomenon called extinction, in which a tactile or visual stimulus is perceived normally when it is presented to one side only, but when it is presented on 31 the side opposite the lesion simultaneously with an identical stimulus on the normal side, the patient neglects the stimulus on the side opposite the lesion. * The frontal lobes are the largest hemispheres and contain vast areas of association cortex. Lesions in the frontal lobes cause a variety of disorders in personality and cognitive functioning. Frontal release signs are “primitive” reflexes that are normal in infants, such as grasp, root, suck, and snout reflexes, but that can also be seen in adults with frontal lobe lesions. In addition, patients with frontal lobe lesions may have particular difficulty when asked to perform a sequence of actions repeatedly or to change from one activity to another. In doing these tasks they tend to perseverate, meaning that they repeat a single action over and over without moving on to the next one. Personality changes with frontal lobe lesions may include impaired judgment, a cheerful lack of concern about one’s illness, inappropriate joking, and other disinhibited behaviors. Other patients with frontal lobe lesions may be abulic (opposite of ebullient), with a tendency to stare passively and to respond to commands only after a long delay. Frontal lesions can also cause a characteristic unsteady magnetic gait, in which the feet shuffle close to the floor, and urinary incontinence. * Lesions in the visual association cortex in the parieto-occipital and inferior temporal lobes can produce a variety of interesting phenomena, including prosopagnosia (inability to recognize faces), achromatopsia (inability to recognize colors), palinopsia (persistence or reappearance of an object viewed earlier), and other phenomena. Seizures in the visual association cortex can cause elaborate visual hallucinations. 32 14. Blood Supply to the Brain and Spinal Cord 1) BRAIN There are two pairs of arteries that carry all the blood supply to the brain and one pair of draining veins. The internal carotid arteries form the anterior blood supply, and the vertebral arteries, which join together in a single basilar artery, form the posterior blood supply. The anterior and posterior blood supplies from the carotid and vertebrobasilar systems, respectively, join together in an anastomotic ring at the base of the brain called the circle of Willis. The main arteries supplying the cerebral hemispheres arise from the circle of Willis. Ordinarily, however, the anterior and middle cerebral arteries derive their main blood supply from the internal carotid arteries (anterior circulation), while the posterior cerebral arteries derive their main supply from the vertebrobasilar system (posterior circulation). The main arteries supplying the brainstem and cerebellum also arise from the vertebral and basilar arteries. These include 33 the superior,anterior inferior, and posterior inferiorcerebellar arteries. Venous drainage for the brain is provided almost entirely by the internal jugular veins. 2) SPINAL CORD The spinal cord receives its blood supply from the anterior spinal artery, which runs along the ventral surface of the cord in the midline and from the paired posterior spinal arteries, which run along the right and left dorsal surfaces of the cord. The anterior and posterior spinal arteries are supplied in the cervical region mainly by branches arising from the vertebral arteries. In the thoracic and lumbar regions, the spinal arteries are supplied by radicular arteries arising from the aorta. 34 35 36 15. CSF circulation and brain ventricles CIRCULATION OF CEREBROSPINAL FLUID CSF circulates through the four brain ventricles (two lateral ventricles and a third and fourth ventricle) and in the subarachnoid space surrounding the brain and spinal cord. Most of the CSF is reabsorbed into the venous system through the arachnoid granulations and through the walls of the capillaries of the central nervous system and pia mater. 37 Scheme to show the major tracts passing through the brainstem. SC = superior colliculus. RN = red nucleus. VN = vestibular nuclei. RFP = reticular formation of pons. RFM = reticular formation of medulla. IC = inferior colliculus. SO = superior olivary nucleus 38