Spinal Cord Anatomy Lectures 1-10 PDF
Document Details
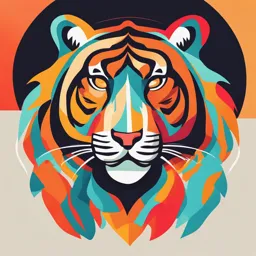
Uploaded by IntuitiveAustin
Assiut University
Dr. Merry Beniamen Kostandy
Tags
Summary
These lectures provide a detailed overview of spinal cord anatomy, including its gross features, spinal nerves, plexuses, and clinical correlations. The material covers several key aspects, such as dermatomes for clinical diagnoses and the blood supply of the spinal cord.
Full Transcript
Lecture (1): Spinal cord I (Anatomy) Source: First Aid USMLE, Page: 446 – 447 Source: Nervous System Basic science and clinical condition. Page: 495 & 498 Source: Nervous System Basic science and clinical condition. Page: 60.62 Source: Kaplan neuroscience USMLE Lectur...
Lecture (1): Spinal cord I (Anatomy) Source: First Aid USMLE, Page: 446 – 447 Source: Nervous System Basic science and clinical condition. Page: 495 & 498 Source: Nervous System Basic science and clinical condition. Page: 60.62 Source: Kaplan neuroscience USMLE Lecture notes 2021; Pages: 251-255. Specific learning Objectives 1- Describe main gross anatomical features of nervous system including: brain stem, spinal cord, spinal and cranial nerves and organs of special sense Contents: By the end of the lecture the student will be able to: 1. Describe the anatomy of the spinal cord 2. Describe the anatomy of the spinal nerves. 3. List the body plexuses and their formation. 4. Interpret anatomical facts with the clinical manifestations of some vascular lesions (anterior spinal artery infarction and cauda equina and conus medullaris syndromes 5. Organizes the landmark dermatomes on a diagram of the human body. 6. Correlate the anatomy of the spinal cord with the lumbar puncture procedure (level and structures that the needles pass through). 7. Correlate anatomical facts with the injury of spinal cord and vertebral column. NARS: (4.1,). ANATOMY OF THE SPINAL CORD The spinal cord continues caudally from the brain stem as a long cordlike structure. It is about 45 cm long. It is located within the vertebral canal which provides structural protection. It is held in place by: 1-Spinal nerves roots. 2- Denticulate ligaments The spinal cord is a segmental structure, giving rise to 31 bilaterally paired spinal nerves along the cord: Cervical nerves (C1–C8) Thoracic nerves (T1–T12) Lumbar nerves (L1–L5) Sacral nerves (S1–S5) Coccygeal nerve Levels of the Spinal Cord Extends from the foramen magnum to the level of the disc between L1 and L2 vertebra. At birth, it extends much lower (to the L3 vertebral level). Different spinal cord segments levels can be related to specific vertebral levels: 1 Dr. Merry Beniamen Kostandy Vertebral level Spinal cord segment level Cervical vertebrae e.g. C5 Add 1, e.g. C6 Upper thoracic, T1–T6 Add 2 segments Lower thoracic, T7–T9 Add 3 segments T10 L1–L2 T11 L3–L4 T12 L5 L1 Conus medullaris Figure 1-1: Topographic relations of the spinal cord segments, spinous processes, and bodies of the vertebrae, intervertebral foramina Cervical spinal nerves C1–C7 exit above the vertebra with the same number. Spinal nerve C8 exits below the C7 vertebrae. 2 Dr. Merry Beniamen Kostandy Spinal nerves below C8 exit below the vertebra with the same number (e.g. L1 nerve exits between L1 and L2). Each spinal segment receives sensory input from dermatomal regions of the body and sends motor output to myotomal regions. Conus medullaris: it is the terminal end of the spinal cord at L3 in newborns and at the lower border of L1 in adults (Fig.4-1). Cauda equina (“tail of the horse”): multiple separate motor and sensory roots, which exit the vertebral canal through the lumbar intervertebral and sacral foramina. Meninges: The spinal cord and spinal roots are covered by three layers of meninges (Fig. 4-2) the dura, arachnoid and pia mater and further protection is provided by the presence of cerebrospinal fluid (CSF), which surrounds the cord in the subarachnoid space. Spinal cord enlargements (Fig.4-1): The size of the spinal cord is not uniform along its length. It has: The cervical enlargement (C5 through T1) gives rise to the rootlets that form the brachial plexus, which innervates the upper limbs. The lumbar enlargement (L1 through S3) gives rise to rootlets that form the lumbar and sacral plexuses, which innervate the lower limbs. Cross-Section of the Spinal Cord (Fig.4-3) The spinal cord has a narrow lumen which is called the central canal. The spinal cord is incompletely divided into two symmetrical halves by a deep anterior median fissure and a shallower posterior median sulcus along the longitudinal length of the cord. The two halves are interconnected by two commissures across the median plane: Anterior white commissure (at the bottom of the anterior median fissure) Grey commissure containing the central canal. Commissure: is a band of white matter or grey matter connecting a part of the CNS on one side with the same part on the other side 3 Dr. Merry Beniamen Kostandy Figure 2-2: The Spinal Cord: Its Meninges and Spinal Roots Figure 1-3: Cross section of the spinal cord Grey matter: centrally located and shaped like a butterfly that contains the cell bodies of neurons that send projections either to the periphery or up through the spinal cord tracts. 4 Dr. Merry Beniamen Kostandy It can be subdivided into different functional regions: The dorsal (posterior) grey column or horn, which is associated with sensory perception. Neurons in the dorsal horn project to higher levels of the CNS to carry sensations to the brain stem, cerebral cortex or cerebellum(forming ascending tracts). The ventral (anterior) grey column or horn, contains motor neurons whose axons exit the cord via the ventral root, on their way to the muscles. The lateral horn, present at thoracic and upper two or three lumbar segments, where the cell bodies of the sympathetic preganglionic fibers are found. In the second, third and fourth sacral segments similar group of cells found and give rise to preganglionic parasympathetic fibers. White matter: is divided into three funiculi: anterior, lateral, and posterior. The white matter of the spinal cord is occupied by short(intersegmental) tracts, long ascending tracts and long descending tracts. Tract: is a group of nerve fibers in the central nervous system which have the same origin, the same termination and the same function. Tracts of the spinal cord include: Motor pathways travel away from the brain. The names of motor pathways begin with the brain structure and end with -spinal (ie, corticospinal tract). Sensory pathways travel toward the brain. The names of sensory pathways begin with spino- and end with the brain structure (ie, spinothalamic tract) Blood Supply Arterial supply 1- Anterior spinal artery: formed by union of two arteries each arises from the vertebral artery. It supplies the anterior two thirds of the cord. 2- Posterior spinal arteries arise from vertebral arteries, supply the posterior third of the cord. 3- Segmental radiculospinal arteries. Give rise to anterior and posterior radicular arteries that accompany the anterior and posterior roots of the spinal nerves. (Fig. 4- 4) Venous drainage 5 Dr. Merry Beniamen Kostandy Veins of the spinal cord drain into six longitudinal channels that communicate with the dural sinuses superiorly and drain into the internal (epidural) and external vertebral venous plexus. Figure 1-4: Blood supply of the spinal cord SPINAL NERVES The peripheral nervous system (PNS) consists of: 1. 12 pairs of cranial nerves. 2. 31 pairs of spinal nerves. 3. The peripheral part of the autonomic nervous system. Spinal nerves are formed by the union of dorsal and ventral roots. Dorsal roots transmit sensory information from skin, muscle and joints. The dorsal root contains a swelling called the dorsal root ganglion that houses the first order neurons of all the sensory pathways (Fig.4-5). Ventral roots transmit motor and autonomic information to the periphery. Spinal nerves divide into dorsal and ventral rami. The dorsal rami: serve sensorimotor functions of the back. Ventral rami: Cervical, lumbar and sacral ventral rami merge with adjacent rami to form four major plexuses: Cervical plexus C1-C4 Brachial plexus C5-T1 Lumbar plexus L1-L4 Sacral plexus L4-S4 The thoracic ventral rami project directly to the thoracic and abdominal walls. 6 Dr. Merry Beniamen Kostandy White communicating rami: Contain myelinated preganglionic sympathetic fibers. Found only from T1 to L3 in conjunction with the lateral horn and intermediolateral cell column. Gray communicating rami: Contain unmyelinated postganglionic sympathetic fibers. Figure 1-5: Formation of the spinal nerve Spinal nerves possess: Two types of sensory fibers: General somatic sensory --- General visceral sensory Two types of motor fibers: General somatic motor --- General visceral motor AUTONOMIC NERVOUS SYSTEM The autonomic nervous system (ANS) is concerned with the motor innervation of smooth muscle, cardiac muscle, and glands of the body. Anatomically and functionally, the ANS is composed of 2 motor divisions: (1) sympathetic and (2) parasympathetic In both divisions, 2 neurons form an autonomic pathway (Fig. 4-6). l-Preganglionic neurons have their neuronal cell bodies in the CNS, their axons exit in cranial and spinal nerves. 2 Postganglionic neurons have cell bodies in autonomic ganglia in the peripheral nervous system (PNS) 7 Dr. Merry Beniamen Kostandy Figure 1-6. Autonomic Nervous System Sympathetic Nervous System: The preganglionic cell bodies of the sympathetic nervous system are found in the lateral horn grey matter of spinal cord segments T1– L3 (thoracolumbar outflow). The postganglionic cell bodies of the sympathetic system are found in one of 2 types of motor ganglia in the PNS: l-Sympathetic chain 2-Collateral or prevertebral (found only in abdomen or pelvis) Parasympathetic Nervous System: The preganglionic cell bodies of the parasympathetic nervous system are found in the CNS in one of 2 places (craniosacral outflow): l -Parasympathetic nuclei of cranial nerves III, VII, IX, and X. 2 -Sacral segments S2, 3 and 4 The postganglionic cell bodies of parasympathetic nervous system are found in terminal ganglia that are usually located near the organ innervated or in the wall of the organ. Dermatomes A dermatome is the area of skin supplied by the cutaneous branches of an individual spinal nerve (Fig.4-7). knowledge of the skin regions corresponding to particular dermatomes plays an important role in the clinical diagnosis of peripheral nerve lesions. Landmark dermatomes C2 Posterior half of skull C3 High turtleneck shirt C4 Low-collar shirt C5 Lateral shoulder 8 Dr. Merry Beniamen Kostandy When the diaphragm or the surrounding abdominal structures are inflamed and cause diaphragmatic irritation (ie, cholecystitis, ruptured spleen), the patient often feel pains in the shoulder because the corresponding dermatome shares a C3–C5 nerve root with the phrenic nerve (referred pain). C6 lateral forearm and thumb. T7 At the xiphoid process T10 At the umbilicus (Important point of referred pain in early appendicitis) L1 At the Inguinal Ligament L4 Front of the knee S2, S3, S4 Perianal area (Saddle area) Myotome: the muscles innervated by a single ventral root. Muscles sharing a common primary action are supplied by the same segment and opposing muscles by segments in sequence with the former. Reflexes are used to test the integrity of the spinal cord at their corresponding levels. Achilles reflex = S1, S2 Patellar reflex = L3, L4 Biceps and brachioradialis reflexes = C5, C6 Triceps reflex = C6, C7 Herniation of the intervertebral disc: Over 90% of clinically evident herniated discs occur at L4/L5 and L5/S1. Cauda equina syndrome: Lesion of the L3-Co nerve roots. Is characterized by unilateral muscle atrophy, radicular pain and areflexia with loss of pain in saddle area. It has a gradual onset. Conus medullaris syndrome: Lesion of S3-Co segments. Is characterized by bilateral loss of sensation in saddle- shaped area and severe incontinence with sudden onset. 9 Dr. Merry Beniamen Kostandy Figure 1-7: Dermatomes distribution. 10 Lecture (2): Spinal cord II (Anatomy) Source: First aid for the basic sciences (organ systems): Chapter 6, Page 446 – 447 Source: Nervous System Basic science and clinical condition. Page: 60-62 Source: Kaplan neuroscience USMLE Lecture notes 2021; Pages: 262-274 Specific learning Objectives 1- Select common investigations relevant to the findings on physical examination, laboratory investigations and histopathology and in the following common clinical problems: spinal cord lesions, stroke. 2- Formulate a broad differential diagnosis for each problem, based on the clinical encounter and investigations done to date in a stable patient presenting with one of the following straight forward problems: e.g. paralysis. 3- Describe main gross anatomical features of nervous system including brain stem, spinal cord, spinal and cranial nerves and organs of special sense. Contents: By the end of the lecture the student will be able to: 1. Describe the normal anatomy of the main ascending (sensory) and descending (motor) tracts. 2. Interpret anatomical facts with the clinical manifestations of some vascular lesions. NARS: (1.6.1.10,.4.1). Tracts of the Spinal Cord Figure 2-1: Transverse section in the spinal cord DESCENDING (MOTOR) PATHWAYS 1- Pyramidal pathway 2-Extrapyramidal pathway 1-Pyramidal pathway: Includes two tracts: a-Corticospinal tracts b-Corticobulbar tract 1 Dr. Merry Beniamen Kostandy CORTICOSPINAL TRACTS Include two tracts: 1-Lateral (crossed)corticospinal tract: Origin: One third from the giant cells of Betz in Primary motor cortex (Brodmann area 4). One third from Premotor cortex and supplementary motor cortex (Brodmann area 6). One third from parietal lobe (Brodmann area 3,1,2). Course: The axons pass through (Fig.2-2). Corona radiata The posterior limb of the internal capsule In the midbrain pass through the crus cerebri. In the pons the fibers continue through the basilar part. In the medulla, forming the “pyramids” and decussate in the pyramidal decussation. Before the decussation, the lateral and ventral corticospinal tracts run together. At the pyramids in the medulla, 85–90% of corticospinal fibers decussate and form the lateral corticospinal tract; the remaining 10–15% continue as the ventral corticospinal tract. In the spinal cord. The axons travel along the corticospinal tract in the lateral funiculus. Termination: Motor neurons in the ventral horn of the spinal cord. 2- Ventral (direct) corticospinal tract: Course: Same as that of the lateral corticospinal tract BUT The ventral corticospinal tract does not decussate and continues ipsilaterally along the ventral white matter of the spinal cord. Termination: On motor neurons within the ventral horn of the spinal cord bilaterally. It is only present in upper part of the spinal cord ending at midthoracic level. 2 Dr. Merry Beniamen Kostandy CORTICOBULBAR TRACT It is the part of the pyramidal tract that is concerned with the voluntary movements of muscles of the head, neck, and face. Fibers pass through the genu of internal capsule and descend to the brain stem where they end on the motor cranial nerve nuclei. All motor nuclei of the cranial nerve, have a bilateral corticobulbar innervation. except those of the lower facial muscles (lower half of motor facial nucleus) and the portion of hypoglossal nerve nucleus that innervates genioglossus muscle as they receive corticobulbar fibers of the opposite side only. Note that muscles involved in chewing, swallowing and talking, have a bilateral innervation. 2-EXTRAPYRAMIDAL PATHWAYS: They include tracts that originate in the brainstem, carrying motor fibres to anterior (ventral) horn cells of the spinal cord. a-Rubrospinal tract (crossed) Arise from red nucleus in midbrain – it lies in lateral funiculus b-Medial (bilateral)and lateral vestibulospinal tracts Arise from vestibular nuclei (in Medulla) it lies in the anterior funiculus. c-Medial and lateral reticulospinal tracts Arise from reticular formation of the pons and medulla respectively. Medial tract lies in the anterior funiculus while lateral reticulospinal tract in the lateral funiculus. d-Tectospinal tract Arise from the colliculi (tectum) of midbrain of opposite side to cervical segment of spinal cord. 3 Dr. Merry Beniamen Kostandy Figure 2-2: Corticospinal tracts SENSORY PATHWAYS I- FOR CONSCIOUS PERCEPTION: 1. Dorsal Column Medial Lemniscus pathway. 2. Spinothalamic tracts. 4 Dr. Merry Beniamen Kostandy II- FOR UNCONSCIOUS PERCEPTION: 1. Spinocerebellar tracts 3. Spinotectal tract 2. Spino-olivary tract 4. Spinoreticular tract Dorsal column–medial lemniscal pathway: Mediates conscious appreciation of proprioceptive and tactile sensations First-order neurons: Cell bodies in the dorsal root ganglia. Axons project to the spinal cord and give rise to the following (Fig.2-3): The gracile tract (medial) arises from sensory axons in the lower extremities and ascends the spinal cord and synapses on the gracile nucleus. The cuneate tract (lateral) arises from sensory axons in the upper extremity and ascends the spinal cord and synapses on the cuneate nucleus. Since the cuneate fasciculus carries sensory axons from the upper extremity to the spinal cord, it does not exist below T2 segment of the spinal cord. Second-order neurons: Cell bodies of gracile and cuneate nuclei of the medulla. Their axons decussate in the medulla as internal arcuate fibers(Fig.2-3). Then ascend in the contralateral side forming the medial lemniscus. Third-order neurons: Ventral posterolateral (VPL) nucleus of the thalamus. posterior limb of the internal capsule primary somatosensory cortex (Brodmann areas 3,1,2) in the postcentral gyrus Anterolateral (spinothalamic tract) system Carries pain, temperature, and crude touch sensations from the extremities and trunk. First-order neurons: Cell bodies in the dorsal root ganglia. Axons project to the spinal cord and ascend or descend a few levels within the tract of Lissauer. 5 Dr. Merry Beniamen Kostandy Second-order neurons: Substantia gelatinosa cells in the dorsal horn. Axons decussate in the ventral white commissure and ascend in the contralateral ventral part of lateral funiculus forming the spinothalamic tract. Third-order neurons: Ventral posterolateral (VPL) nucleus of the thalamus posterior limb of the internal capsule primary somatosensory cortex (Brodmann areas 3,1,2) in the postcentral gyrus. Syringomyelia Involves enlargement of the central canal of the spinal cord, this lead to damaging the fibers of the spinothalamic tract (the dicussating fibers). Syringomyelia results in a “belt like or “cape-like” loss of pain and temperature because it is most commonly localized in C8– T1 segments. Figure 2-3: Dorsal column–medial lemniscus pathway 6 Dr. Merry Beniamen Kostandy Figure 2-4: Spinothalamic tract Sensory Pathways from head and face Carried by trigeminal nerve Trigeminal ganglion trigeminal nerve nuclei axons from these nuclei cross to opposite sideform trigeminal leminiscus Ventral posteromedial (VPM) nucleus of thalamus primary somatosensory cortex. Spinocerebellar tracts (Fig.2-5). They include: 1- The posterior spinocerebellar tract 2-The anterior spinocerebellar tract 1-The posterior spinocerebellar tract The first-order neuron, has its cell body in the posterior root ganglion. The second-order neuron: nucleus dorsalis (Clarke's column) at the base of dorsal horn. 7 Dr. Merry Beniamen Kostandy The axons of the second-order neurons enter the lateral white column on the same side and ascend the medulla oblongata, joins the inferior cerebellar peduncle and terminates in the ipsilateral cerebellar cortex(Fig.2-5). 2- The anterior spinocerebellar tract The first-order neuron, has its cell body in the posterior root ganglion The second-order neuron: cells at lamina VII in dorsal horn. The axons of the second-order neurons enter the lateral white column on the opposite side, ascend through the brain stem where they cross back before entering the cerebellum via the superior cerebellar peduncle and terminates in the ipsilateral cerebellar cortex (Fig.2- 5). Figure 2-5: Spinocerebellar tracts Anterior cord syndrome: caused by trauma, fracture dislocation of vertebrae, herniation of vertebral discs or occlusion of the anterior spinal artery. Presents with: Bilateral loss of pain and temperature that is carried by (lateral spinothalamic tract) Bilateral spastic paresis due to injury of corticospinal tract. The dorsal columns are spared 8 Lecture (3): Brain stem (Anatomy) Source: First aid for the basic sciences (organ systems): chapter 6, Page 441-442 & 444-445 Source Oxford handbook of medical sciences. Page: 412 Source: Kaplan neuroscience USMLE Lecture notes 2021; Pages: 275-289 Specific learning Objectives 1- Describe main gross anatomical features of nervous system including: brain stem, spinal cord, spinal and cranial nerves and organs of special sense 2- Correlate main gross anatomical features of nervous system with the following clinical situations: cranial nerve injuries, brain stem and spinal cord lesions, stroke, hydrocephalus, cerebellar syndromes Contents: By the end of the lecture the student will be able to: 1. Recognize the cranial nerve nuclei in the brain stem 2. Describe the structure of the pons and medulla oblongata (rhombencephalon). 3. Describe the structure of the midbrain (mesencephalon). NARS: (4.1,). Brain stem consists of three main components, from superior to inferior: Midbrain, pons, and medulla (Fig.3-1). MIDBRAIN The midbrain, or mesencephalon, connects the forebrain to the hindbrain and contains the cerebral aqueduct. It is 2 cm in length. A nearly coronal plane through the cerebral aqueduct divides the midbrain into: Tectum posteriorly, which demonstrates four raised structures: Superior colliculi: The center for visual reflexes. Each colliculus is connected to lateral geniculate body by superior brachium. Inferior colliculi: Major station in the auditory pathway. Each colliculus is connected to the medial geniculate body by inferior brachium. Cerebral peduncles anteriorly: Two thick nervous cords separated by interpeduncular fossa. Formed of (Fig.3-2,3): a-Crus cerebri b -Substantia nigra c - Tegmentum Cranial nerves attached to the midbrain Oculomotor nerve emerges anteriorly. Trochlear nerve emerges from the posterior aspect. 1 Dr. Merry Beniamen Kostandy Figure 3-1: Ventral view of the brainstem showing the locations of the cranial nerves as they enter or exit the midbrain, pons, and medulla. Internal structure of mid brain (Fig.3-2,3): Crus cerebri: occupied by Middle two-thirds of the crus corticospinal and corticonuclear fibers On each side corticopontine fibers. Substantia nigra: A lamina of pigmented neurons (contain melanin) Important site of dopaminergic neurons in the brain A part of the basal ganglia. Loss of dopaminergic neurons in substania nigra cause Parkinson disease Tegmentum 1- Lemniscal tracts: Medial, trigeminal, lateral and spinal leminisci. 2- Cranial nerve nuclei in the midbrain: Oculomotor nerve nuclei and root: Edinger-Westphal nucleus (origin of preganglionic parasympathetic fibers) and motor nucleus. Trochlear nerve nucleus. Mesencephalic nucleus of the trigeminal nerve. 3- The red nucleus. 2 Dr. Merry Beniamen Kostandy 4- Medial Longitudinal Fasciculus A fiber bundle lies close to the median plane along the whole length of the brain stem. Interconnecting centers for conjugate horizontal eye movements, the vestibular nuclei, and the nuclei of CN III, IV, and VI which innervate skeletal muscles that move the eyeball. Lesions of the fasciculus produce internuclear ophthalmoplegia often seen in multiple sclerosis (MS). Midbrain is connected to the cerebellum through the superior cerebellar peduncle. Figure 3-2: Brain stem and cross- section of the midbrain Figure 3-3: Cross section of the midbrain PONS The pons (or metencephalon) lies inferior to the midbrain, superior to the medulla, and on the anterior surface of the cerebellum. Anteriorly, characterized by transverse pontine fibers that converge on each side to form the middle cerebellar peduncle. There is a shallow groove in the midline, the basilar groove, which lodges the basilar artery. 3 Dr. Merry Beniamen Kostandy Posteriorly forms the upper half of the floor of the fourth ventricle. It is divided into a posterior part, the tegmentum, and an anterior basilar part Cranial nerves attached to the pons: 1- Trigeminal nerve: It emerges on each side. Each nerve consists of a smaller, medial motor root, and a larger lateral sensory root. 2- In the groove between the pons and the medulla oblongata, there emerge, from medial to lateral, the abducent nerve and in the cerbellopontine angle (angle of junction between cerebellum, pons and medulla), facial and vestibulocochlear nerves. Internal structure of the pons (Fig.3-4): The basilar part of the pons Pontine nuclei. Transverse pontine fibers, they enter the middle cerebellar peduncle. Corticospinal and corticonuclear tracts. The tegmentum of the pons contains: 1- Lemniscal tracts: Medial, trigeminal, lateral and spinal leminisci 2- Cranial nerve nuclei in the pons: 1-Trigeminal nerve nuclei (motor nucleus, spinal, mesencephalic and main sensory nuclei). 2-Abducent nerve nucleus 3-Facial nerve nucleus: motor nucleus. 4-Vestibular nuclei. 5- Cochlear nuclei. 6- Superior and inferior salivary nuclei at the junction of pons and medulla. 3-Medial Longitudinal Fasciculus N. B Vestibular schwannoma (acoustic neuroma): Benign tumor of vestibulocochlear nerve that arises in the area of the internal auditory meatus and cerebellopontine angle. May impinge on several cranial nerves that pass near the cerebellopontine angle: 4 Dr. Merry Beniamen Kostandy CN VIII results in tinnitus, unilateral sensorineural deafness. CN VII results in ipsilateral facial weakness. CN V results in paresthesia, anesthesia of the ipsilateral face. Figure 3-4: Cross section of the pons MEDULLA OBLONGATA The medulla oblongata connects the pons superiorly with the spinal cord inferiorly. The junction of the medulla and spinal cord is at the origin of the first cervical spinal nerve just below the level of the foramen magnum. The medulla oblongata is conical in shape. The central canal of the spinal cord continues upward into the lower half of the medulla (closed medulla); in the upper half of the medulla (open medulla), it expands as the cavity of the fourth ventricle. On the anterior surface of the medulla is the anterior median fissure. On each side of the median fissure, there is a swelling called the pyramid. Posterolateral to the pyramids are the olives. Posterior to the olives are the inferior cerebellar peduncles, which connect the medulla to the cerebellum. The posterior surface of the inferior half of the medulla is continuous with the posterior aspect of the spinal cord and possesses a posterior median sulcus. On each side of the 5 Dr. Merry Beniamen Kostandy median sulcus, there is the gracile tubercle, overlying gracile nucleus. Lateral to the gracile tubercle is the cuneate tubercle overlying cuneate nucleus. Cranial nerves attached to the medulla oblongata 1- The hypoglossal nerve: In the groove between the pyramid and the olive. 2- The glossopharyngeal, vagus nerves and the cranial roots of the accessory nerve. In the groove between the olive and the inferior cerebellar peduncle The internal structure of the medulla oblongata 1- The nucleus gracilis and nucleus cuneatus give rise to axons that decussate as internal arcuate fibers and ascend in the medial lemniscus. 2- The corticospinal (pyramidal) tracts, which are contained in the pyramids. Most of these fibers decussate and then travel down the spinal cord as the (lateral) corticospinal tract (pyramidal decussation) 3- Inferior olivary nuclei. send olivocerebellar fibers into the cerebellum through the inferior cerebellar peduncle. 4- Spinal lemniscus and the descending hypothalamic fibers. 5-Posterior and anterior spinocerebellar tracts. 3- Vestibulospinal, and the rubrospinal tracts. 7-The medial longitudinal fasciculus. Cranial nerve nuclei in the medulla oblongata 1- Spinal nucleus and spinal tract of trigeminal nerve 2- Solitary nucleus: it receives taste and general visceral sensations carried by facial, glossopharyngeal and vagus nerves. 3- Nucleus ambiguus: joins glossopharyngeal, vagus and cranial accessory nerves supply muscles of the soft palate, pharynx and larynx. 4- Dorsal motor nucleus of vagus. 5-Hypoglossal nucleus. Reticular formation Running through the core of the brainstem tegmentum. Consists of a diffuse network of neurons that exerts a widespread influence on CNS functions. 6 Dr. Merry Beniamen Kostandy The descending hypothalamic fibers arise in the hypothalamus and course without crossing through the brain stem to terminate on preganglionic sympathetic neurons in the spinal cord. Lesions of this pathway produce ipsilateral Horner syndrome. Wallenberg syndrome (lateral medullary syndrome)(Fig.6-5) Is particularly common as a result of occlusion in posterior inferior cerebellar artery (supplying posterolateral part of the medulla) Characterized by: Nystagmus, vertigo due to injury of Vestibular nuclei Ipsilateral cerebellar signs due to injury of Inferior cerebellar peduncle Dysarthria, hoarseness, dysphagia, loss of gag reflex due to injury of Nucleus ambiguus Contralateral loss of pain and temperature sensation from trunk and extremities, with ipsilateral loss of pain and temperature sensation in the face due to injury of Spinothalamic tracts/spinal trigeminal nucleus and tract Ipsilateral Horner syndrome due to injury of Descending sympathetic tract Medial Medullary Syndrome (Vertebral or Basilar Artery Occlusion): o Lesion of corticospinal tract Contralateral hemiparesis. o Lesion of medial lemniscus Contralateral loss of tactile, vibrational, and proprioceptive sense. o Lesion of hypoglossal nerve as a cranial nerve sign Ipsilateral lower motor neuron lesion of hypoglossal nerve (tongue points to side of lesion) inferior alternating hemiplegia. Figure 3-5: Cross section of the medulla 7 Dr. Merry Beniamen Kostandy Types of cranial nerves fibers: Cranial nerves possess 1-Four types of sensory (afferents) fibers: General somatic sensory (GSA): concerned with general sensations from the head. General visceral sensory (GVA) Special somatic sensory (SSA): concerned with hearing and balance (VIII) and vision (II)) Special visceral sensory (SVA) (taste (carried by VII, IX, X nerves) and smell) 2-Three types of motor (efferent) fibers: General somatic motor (GSE) (innervate the extra-ocular muscles (nerves III, IV, VI) and tongue muscles (nerve XII). General visceral motor (GVE)(parasympathetic) innervate the salivary, lacrimal glands and smooth muscles (nerves III, VII, IX and X). Special visceral motor (SVE): controlling the striated (skeletal) muscles associated with the facial expression, chewing, neck, larynx and pharynx movements (nerves V, VII, IX, X, XI) that are derived from the branchial arches during development. 8 Dr. Merry Beniamen Kostandy Cranial nerve Nucleus name (type of Nucleus Function fibers) location Olfactory SVA Smell Optic SSA Vision Oculomotor Oculomotor – GSE Midbrain Eye movements nerve Constricts pupil and Edinger -Westphal Midbrain accommodation nucleus-GVE Trochlear nerve Trochlear -GSE Midbrain Eye movements Trigeminal Principal GSA Pons Facial sensation nerve (touch) Spinal GSA Medulla Facial sensation (pain) Midbrain/Pons Proprioception Mesencephalic GSA Mastication Motor SVE Pons Abducent nerve Abducent – GSE Pons Eye movements Facial nerve Motor SVM Pons Facial expressions Solitary SVA Taste Superior salivatory GVE Salivation, lacrimation Vestibulocochlear Vestibular SSA Pons Balance nerve Cochlear SSA Hearing Glossopharyngeal Nucleus ambiguous SVE Medulla Stylopharyngeus nerve muscle Inferior salivatory GVE Secretory to parotid gland Solitary GVA, SVA Taste Sensation from pharynx Vagus nerve Nucleus ambiguous SVE Medulla Swallowing and Dorsal motor vagal GVE talking Solitary SVA,GVA Accessory nerve Cranial accessory Medulla Swallowing and nucleus ambiguous talking SVE Spinal accesssory- GSE Head and shoulder movements Hypoglossal Hypoglossal GSE Medulla Tongue movements nerve Table 1: Cranial nerves nuclei 9 Dr Raghda Elsherif Lecture (4): Histological structure of the neurons and glia (neuroglia). (Histology) Specific learning Objectives: 1- Recognize the microscopic structure and types of neurons, neuroglia, nerve fibers, meninges, and brain barriers and justify their structural diversity. 2- Correlate the normal microscopic structure of neurons, nerves and neuroglia at the cellular and molecular levels to brain function and hemostasis. 3- Explain signs of some diseases of the neurons, synapses, neuroglia, and cornea on the basis of histological features Contents: By the end of the lecture the student will be able to: 1. Mention the different types of neurons and nerve fibers in the body. 2. Describe the structure of neurons and nerves. 3. Identify different types, structure and function of neuroglia. NARS: (1.8. 4.1, 4.2). Reference books: -Integrated systems; Ch. (2), Pages: 55 &56, -Junqueira’s Basic Histology; Ch. (9), Pages: 161-170. -Kaplan neuroscience USMLE Lecture notes 2021; pages 235:241. NERVOUS TISSUE The nervous system is divided anatomically into the central nervous system )CNS) and the peripheral nervous system (PNS). Nervous tissue of the CNS does not contain connective tissue. The two major classes of cells that make up the nervous tissue are nerve cells, neurones, and supporting cells, glia. Neurones The neuron is the structural and functional unit of the nervous system. The vast majority of neurones is generated before birth. Mature neurones are not mitotically active, i.e. they do not divide. Neurons in several parts of the brain and other parts of the body behave as secretory cells and are referred to as neurosecretory cells. These are nerve cells, such as those of the hypothalamus, which elaborate a chemical substance (for example, releasing factor, neuropeptide, or, more rarely, a true hormone) that influenc es the activity of another structure (for example, anterior lobe of the hypophysis). 84 Dr Raghda Elsherif The nerve cell body (soma or perikaryon): 1. Light microscopic structure: The nucleus is usually situated at the centre of the cell body. It is pale in staining, with well-developed nucleolus. Basophilic granules of various shapes and sizes are present in the cytoplasm of the cell body as well as in the dendrites. They are called Nissl granules. The cell bodies of different neurons differ greatly in their size. When the cytoplasm is stained with silver stain it shows numerous neurofibrils (NF) running parallel to each other. 2. Electron microscopic structure: a) Cell organelles: The EM reveals the structure of Nissl granules to be groups of free ribosomes together with RER. Golgi bodies located around the nucleus. The main function of the Golgi in nerve cells is the formation of lysosomes. Mitochondria are present in large numbers both in cell bodies and in the processes, particularly at their end feet. Neurofibrils (NF) are resolved into bundles of neurofilament. The neurofilaments, which are intermediate filaments, formed of cytokeratin, run parallel to each other in dendrites and the axon. In the cell body the neurofilaments form a network. Microtubules and neurofilaments occupy the cytoplasmic matrix of the neuron in areas where there are no Nissl granules. Neurofilaments and microtubules are important in intracellular transport and also as a part of the cytoskeleton of the neuron. b) Cell inclusions: Nerve cells contain pigments like lipofuscin granules which represent the end product of lysosomal activity that accumulate with age. They also contain melanin pigments which are present in nerve cells of the substantia nigra of the mid brain. Occasionally lipid droplets may be found in nerve cells but no glycogen could be detected. 85 Dr Raghda Elsherif Nissle substance Neurofibrils (silverstain) Fig. (10-1): EM structure of neuron. The shape of the neurone and its processes Neurones have long processes, which extend from the part of the cell body around the nucleus, the perikaryon or soma. The processes can be divided into two functionally and morphologically different groups, dendrites and axons. Dendrites are part of the receptive surface of the neurone. As a rule, neurones have one to several primary dendrites, which emerge from the perikaryon. Primary dendrites may 86 Dr Raghda Elsherif divide into secondary, tertiary etc. dendrites. Dendrites can be smooth, or they can be studded with small, mushroom-shaped appendages, which are called spines. Each neurone has as a rule one axon, and never more than one axon which emerges from the perikaryon. The point of origin of the axon from the perikaryon is the axon hillock. The axon may, like the dendrites, branch as it travels through the nervous tissue to its destination(s). The axon is the "transmitting" process of the neurone. The axon forms small, bulb-shaped swellings called boutons at the ends (terminal boutons) of its branches. Neuronal Processes (Nerve fibers): The processes of a nerve cell may be divided into dendrites and an axon. 1. Dendrites: They are tree – like tapering processes which give a large neuronal surface for the reception of many axon terminals. Dendrites are generally shorter than the axon but they branch repeatedly and their surface is rough because of the presence of spines or gemmules (mountain). The cytoplasm of the dendrite contains all the constituents of the cell body but Nissl granules are usually confined to its proximal part. 2. Axons: The portion of the axon between its origin and where it becomes surrounded by sheath is called "the initial segment ''. They are thin slender uniform processes which arise from the cell bodies at a region devoid of Nissl granules called the ''axon hillock''. The axon may give a large number of terminal branches called telodendria, the enlarged ends of which are called axon terminals. The plasma membrane of the axon is usually called the axolemma while its cytoplasm is called axoplasm. Axoplasm differs from the cytoplasm of the cell body in lacking Nissl granules and Golgi bodies. So, the axon depends on cell bodies to get their need of protein. The axon acts as an important pathway for transport of materials secreted in the cell body (axoplasmic transport). These materials move, at different rates, towards the axon terminals aided by microtubules and probably by filaments (anterograde transport). 87 Dr Raghda Elsherif Backward transport of material towards the cell body also takes place (retrograde transport). These substances are taken for degradation or reuse. Types of neurons: A- According to the number of fibers, there are three types of neurons: 1. Unipolar neurons (Pseudounipolar): Only one process arises from the cell and bifurcates not far from it into two processes which are almost identical from the histological point of view although they act one as a dendrite and the other as an axon. They are present in spinal ganglia and sometimes are called pseudo-unipolar neurons. 2. Bipolar neurons: From each pole of the usually oval cell arises one fiber; one of them acts as a dendrite and the other as an axon. They are located in olfactory mucosa, retina of the eye, spiral ganglion of the cochlea in the inner ear. 3. Multipolar neurons: This type is the most dominating type. From the cell body arise a number of dendrites which end in the vicinity of the cell and only one axon. The axon is usually long and the neuron in this case is called Golgi type I neuron. Some neurons have short axons ending near the cell and the neuron in this case is known as Golgi type II neuron. Multipolar neurons have a variety of shapes particularly with regards to their cell bodies and accordingly there are a number of multipolar neurons: Stellate neurons which have star-shaped cell bodies and make the majority of multipolar cells, eg. anterior horn cells and cells of autonomic ganglia. Pyramidal cells which have cell bodies in the form of pyramids from the corners of which arise dendrites while the axon arises from the centre of the base. They are mainly present in the cerebral cortex. Purkinje cells which have flask – shaped or fusiform cell bodies. Dendrites arise from the apex of the cell while the axon arises from the base. They are located in the cerebellar cortex. 88 Dr Raghda Elsherif Fig. (10-2): Types of neurons. B- According to the function, there are two types of neurons: 1- Sensory neurons: bring signals into the CNS 2- Motor neurons: carry signals out of the CNS. 3- Interneurons, which are found only in the CNS, connect one neuron to another. They receive information from other neurons (either sensory neurons or interneurons) and transmit information to other neurons (either motor neurons or interneurons). Synapses They are morphologically specialised contacts between a bouton formed by one neurone, the presynaptic neurone, and the cell surface of another neurone, the postsynaptic neurone. Synapses are composed of three main parts: The presynaptic ending that contains synaptic vesicles. The synaptic cleft between the two nerve cells The postsynaptic ending that contains receptor sites 89 Dr Raghda Elsherif Synaptic vesicles, which contain the neurotransmitters, typically accumulate close to the site of contact between the bouton and the postsynaptic neurone. The release of the neurotransmitter from the synaptic vesicles into the synaptic cleft, i.e. the space between the bouton and the postsynaptic neurone, mediates the transfer of information from the pre- to the postsynaptic neurone. Both the release of the synaptic vesicles and the mediation of the response to the transmitter require membrane-associated specialisations; the pre and postsynaptic densities. Differences in the morphological appearances of the synapses accompany the functional differences. The pre-and postsynaptic densities are typically of equal width, or symmetric, at inhibitory synapses. The postsynaptic density is thicker than the presynaptic density at asymmetric synapses, which are typically excitatory. Types of Synapses: There are two different types of synapses; chemical; the predominant type; and electrical. 1- In a chemical synapse, electrical activity in the presynaptic neuron triggers the release of a chemical called a neurotransmitter that binds to receptors located in the plasma membrane of the postsynaptic cell. Chemical synapses can further be subdivided into excitatory and inhibitory contacts, which dampen signals in the brain. 2-Electrical synapses: the presynaptic and postsynaptic cell membranes are connected by the special channels called gap junctions which allow direct transfer of ions. Types of Synaptic Contacts: in the central nervous system, where most synapses occur, presynaptic neurons can interact with postsynaptic neurons at three locations; Axodendritic synapse; the axon of a presynaptic neuron contacts the dendrite of a postsynaptic neuron. axosomatic synapse; the axon of a presynaptic neuron contacts the cell body of a postsynaptic neuron. Axoaxonic synapse; the axon of a presynaptic neuron contacts the axon of a postsynaptic neuron. 90 Dr Raghda Elsherif Fig. (10-3): Synapses structure. Glial Cells (Neuroglia) Astrocytes They are star-shaped cells. Proximal regions of the astrocytic processes have bundles of intermediate filaments made of glial fibrillary acid protein (GFAP), which serves as a unique marker for this glial cell. Their processes are often in contact with a blood vessel (perivascular foot processes). Two types of astrocytes exist in the CNS; Protoplasmic astrocytes: have many shorter processes and predominate in the gray matter. Fibrous astrocytes: have long delicate processes, are abundant in white matter. Astrocytes provide mechanical and metabolic support to the neurones of the CNS. Astrocytes are also the scar-forming cells of the CNS following brain injury. Oligodendrocytes Have fewer and shorter processes. Oligodendrocytes form myelin sheath (see below) around axons in the CNS and are the functional homologue of peripheral Schwann cells. Oligodendrocytes may, in contrast to Schwann cells in the periphery, form parts of the myelin 91 Dr Raghda Elsherif sheath around several axons. Guillain-Barre disease involves antibodies against Schwann cells but not oligodendrocytes - the peripheral nervous system is affected instead. Microglia Microglia are small cells, present around blood capillaries and have phagocytic functions. In the case of tissue damage, microglia can proliferate and differentiate into phagocytotic cells. Ependymal cells line the ventricles of the brain and the central canal of the spinal cord. They are ciliated simple cuboidal or low columnar epithelium. The lack of tight junctions between ependymal cells allows a free exchange between cerebrospinal fluid and nervous tissue. Ependymal cells lining the choroid plexus characteristically possess tight junctions. Ependymal cells can specialise into tanycytes, which are rarely ciliated and have long basal processes. Glial cells may also communicate with each other via gap junctions. Fig. (10-4): Neuroglial cells. PNS Neuroglial Cells: 92 Dr Raghda Elsherif Satellite cells cover the cell bodies of neurons in PNS ganglia, support the function of the neurons and might act as a protective barrier. Schwann cells: They are neuroglial cells around the peripheral nerve fibers. Fig. (10-5): Satellite and schwann cells. 93 Dr Sanaa A M Elgayar Lecture (5): Histology of meninges, choroids plexus and brain barriers By the end of the lecture the student will be able to: A.6 Define the meninges A.6 Mention the different types of meninges and their structure. B.3 Explain the structure and importance of choroid plexus. A.6 Describe the different brain barriers. NARS: (4.1, 4.2). Reference books: Integrated systems (pp.51), The nervous system (pp.24) & Basic Histology (pp. 176-183) Brain Meninges The brain and spinal cord are well protected by the skull and vertebral column, within which there are three membranes of connective tissue called the meninges; the outer most layer is called the dura mater, the middle layer is the arachnoid (or arachnoid membrane) and the innermost is the pia mater. It includes both cranial and spinal dura mater 1. Cranial dura mater - It is the external layer and composed of dense connective tissue. - It is subdivided into two layers; the periosteal and the meningeal layers. - The dural venous sinuses are present between these two layers. A- The periosteal (or endosteal) layer of the dura mater - It is simply a layer of periosteum that covers the inner surface of the skull. - It is well vascularized, composed of osteoprogenitor cells, fibroblast and organized bundle of collagen fibers. - The periosteal layer of the dura mater attaches loosely to the inner surface of the skull except at the sutures and the base of the skull, where the attachment is firm. 84 Dr Sanaa A M Elgayar B. The meningeal dura mater - It is composed of fibroblasts with elongated processes, they have dark staining cytoplasm and ovoid nuclei. - They are surrounded by Sheet-like layers of fine collagen fibers. This layer contains small blood vessels. - Internal to the meningeal dura, there is a layer of flattened fibroblasts exhibiting long processes that are occasionally attached to one another by desmosomes and gap junctions, this layer is called border cell layer. - Meningeal lymphatic vessels: are present in the meningeal dura parallel to the dural venous sinuses. They are responsible for draining immune cells, small molecules, and excess fluid (components of the CSF that fills the subarachnoid space) into the deep cervical lymph nodes. 85 Dr Sanaa A M Elgayar Meningeal lymphatic vessels 2- Spinal Dura mater - It forms a continuous tube from the foramen magnum to the second segment of the sacrum and is pierced by the spinal nerves. - It does not adhere to the walls vertebral canal. - Epidural (extra dural) Space: is the space between the dura and bony wall of the vertebral canal. It is filled with loose c.t. containing epidural fat and venous plexus. In the cranium, there is no epidural space because the dura is fused with internal periosteum of cranial bones. - Subdural space: is the potential space between the inner surface of the dura and the outer surface of the arachnoid. It contains a light amount of fluid that is not CSF. 86 Dr Sanaa A M Elgayar It is the middle layer of the meninges. It includes a flat sheet- like membrane in contact with dura and a trabecular region, composed of loosely arranged arachnoid trabecular cells (modified fibroblasts) along with a few collagen fibers that contact with the underlying pia matter. The pia and arachnoid maters are sometimes described as a combined membrane, the leptomeninges. The arachnoid membrane and its trabeculae are composed of delicate collagen fibers and some elastic fibers. Both the outer and the inner surfaces of the membranous roof and the trabeculae are covered with a continuous lining of thin flat cells (modified fibroblasts) linking that of the pia matter. The arachnoid layer is avascular although blood vessels course through it. These vessels are isolated from the arachnoid and subarachnoid by modified fibroblasts. In some areas, the arachnoid extends dura forming what is called arachnoid villi. 87 Dr Sanaa A M Elgayar Arachnoid villi perforate the dura mater to protrude into spaces connected to the lumina of the dural venous sinuses. They transfer the CSF from the subarachnoid space into the blood of the venous sinuses. Sub arachnoid space: is the space between the membrane sheet roof of arachnoid and the pia below, through which the arachnoid trabeculae extend. The space is filled with cerebrospinal fluid. III-Pia mater - It is the innermost layer. - It is delicate and highly vascular layer. - It contains interlacing bundles of collagen fibers and some fine elastic fibers. - It is covered with a continuous membrane of squamous cells. - The pia also contains few fibroblasts, macrophages and many blood vessels. - The abundant blood vessels in this layer are surrounded by pia cells interspersed with macrophages, mast cells and lymphocytes. - Fine collagenous and elastic fibers lie between the pia and neural tissue. - Blood vessels, penetrate the neural tissues are covered by pia mater until they form the continuous capillaries characteristic of the CNS then end-feet of astrocytes, pedicles, rather than pia matter, cover capillaries within the neural tissue. 88 Dr Sanaa A M Elgayar Cranial meninges 011 Dr Sanaa A M Elgayar - The main function of the choroid plexus is to secrete the cerebrospinal fluid (CSF). - The choroid plexus is formed of folds of the pia mater that invaginate into the brain ventricles. - The plexus has a core of loose C. T. of the pia mater rich in macrophages. - This core contains an abundance of fenestrated blood capillaries covered by a lining of specialized ependymal cells which are tightly connected with each other by tight junctions. - The apical surfaces of ependymal cells lining the choroid plexus have a covering of hair-like projections as cilia (which circulate CSF) and microvilli (which help in CSF absorption). - Microvilli significantly increase the surface area of the choroid plexus, permitting increased CSF absorption. - Ependymal cells are essential in the production of CSF as the choroid plexus may secrete up to 500 ml of CSF per day in the adult human brain. Choroid plexus 010 Dr Sanaa A M Elgayar Brain ventricles and choroid plexuses Cerebrospinal Fluid Acts as a cushion, support the brain/spinal cord and acts as a filtration system to circulate nutrients and remove metabolic waste from the central nervous system. Once CSF is in the subarachnoid space, it is reabsorbed via arachnoid granulations which were thought to provide the main pathway for absorption of cerebrospinal fluid into venous circulation. The brain was thought to lack lymphatic vessels. Nowadays, it becomes evident that CSF passes from the subarachnoid space, enters the brain via periarterial spaces, then passes into the interstitium, via perivascular astrocytic aquaporin-4. There, CSF is exchanged with interstitial fluid (ISF) and then drives the perivenous drainage of interstitial fluid (ISF) and its solute, from which it eventually reaches the cervical lymph nodes. This system was given the name glymphatic system, a merging of the words ‘glial’ and ‘lymphatic’. This glymphatic flow can clear solutes from the interstitial space and therefore have the same function as lymph outside the brain. The glymphatic system is formed of a network of para-vascular spaces surrounding arterioles, capillaries and venules that allows for the efficient clearance of interstitial solutes and wastes. Glymphatic transport activity is enhanced during sleep, most efficient when the body posture is in the right lateral position compared with the supine or prone positions. 011 Dr Sanaa A M Elgayar Glymphatic System In spite of the rich blood supply of the CNS, the neural elements are kept in a semi-isolated environment due to a barrier system which regulates the movement of chemicals between arterial blood, CSF and brain cells. There are three barriers in the CNS: The blood- brain barrier, the blood -CSF barrier and the brain -CSF barrier. I-The blood – brain barrier: It is the barrier separating the brain tissue from the blood circulation. The structural basis consists of three parts: 1) Layer of endothelial cells interconnected through tight junctions and not containing fenestrations. 2) Basal lamina consisting of basal lamina of astrocytes and basal lamina of endothelial cells 3) Processes of astrocytes (pedicles), which anneal on the basal membrane. This barrier is a differential filter which allows the passage of selective substances from the blood to brain intercellular fluid. 012 Dr Sanaa A M Elgayar The main functions of BBB - Protection of CNS tissue especially neurons against different noxious substances, which would be otherwise able to penetrate to the CNS. - The selectivity of BBB prevents many harmful substances from passing into the CNS. - Generally, hydrophilic substances do not pass BBBuntil the endothelial cells and astrocytes express appropriate transport channels. The hydrophobic nature of BBB, on the other hand, allows the penetration of lipophilic substances. - Encephalopathy caused by unconjugated hyperbilirubinaemia (Kernicterus) may develop in newborns due to underdeveloped blood-brain barrier that does not prevent the bilirubin from entering the brain tissue. It is however very rare in adults with fully developed BBB. Blood Brain Barrier Brain CSF Barrier III- Brain – CSF barrier: It is formed of the ependymal lining of the brain ventricles, basement membrane and subependymal glial elements. It is relatively weak barrier and therefore many substances gain entrance from CSF to the interstitial fluid among the neurons and neuroglia. 013 Dr Sanaa A M Elgayar II- The blood-CSF barrier: It separates the cerebrospinal fluid from blood. It consists of three structurally distinct parts: 1) Choroidal epithelial cells are interconnected by tight junctions. The side facing the CSF has its surfaced enlarged by the presence of projections called microvilli. 2) Basement membrane. 3) Endothelium of the pia mater capillaries containing fenestrations. The main functions of Blood CSF Barrier - It restricts the passage of substances from the blood into the CSF. - It is more permeable than BBB and many plasma proteins thus enter the cerebrospinal liquid (through pinocytosis or active transport). Their concentrations are - lower than those of blood plasma. - An impairment of blood CSF barrier leads to an increase of the concentration of proteins in CSF. - The transport occurs in the opposite direction as well and the substances from CSF can enter the circulation. - A compromise in the blood-CSF barrier allows dangerous microbes to infect the central nervous system which is present in infections such as meningitis 014 Lectures (6): Cerebral circulation and physiology of cerebrospinal fluid (Physiology) Prof. Mahmoud R Abdel Fadeil Prof. Dalia Gamal Eldin Mostafa Specific learning Objectives: 1- Mention the foundational physiological, concepts relevant to the pathophysiology of brain edema, basal ganglia lesions and cerebellar ataxia. 2- Normal physiological aspects of cerebral circulation, blood brain barrier, cerebrospinal fluid, and its normal function. Contents: By the end of the lecture the student will be able to: 1. Recognize mechanism of regulation and auto regulation of cerebral 2. blood flow. 3. Explain the effect of brain activity on cerebral blood flow. 4. Identify formation, absorption and composition of cerebrospinal fluid (CSF). 5. List functions of CSF. 6. Recognize the blood brain barrier. 7. Describe mechanism of transport of substances across blood brain barrier. 8. Recognize factors influencing the intracranial pressure. 9. Explain mechanisms of brain edema. NARS: (1.8 ; 4.1). References books: Integrated Neuro Book Basic sciences and clinical conditions Adina Michael Titus and Peter Shortland pp. 51; 57; 62, 62f; 7576; 112; 200; 201; 234. Kaplan USMLE Step 1 pp. 109, 110, 242 and 245-249. Cerebral Circulation The metabolism of the brain is high and drives from oxidation of glucose. Since the brain does not contain a significant store of either oxygen or glucose, an adequate cerebral blood flow is essential for its function. Physiologic Considerations: The average cerebral blood flow in normal adult is about 750 - 900 ml/minute 1 or about 15% of the total resting cardiac output. NB: The brain weighs about 1400 gm which is 2% of the total body weight. The average O2 consumption in man is 3.5 mL/100g brain tissue/min. The brain is sensitive to lack of O2. Lack of oxygen for 10 seconds leads to unconsciousness and irreversible damage of brain tissue within few minutes. An obstruction or block in a sizeable cerebral artery due to atherosclerosis may lead to symptoms of stroke. Stroke is usually the result of death of brain tissue either from a lack of blood supply or from bleeding into the tissues. In resting humans, the average blood flow is greater to the grey matter than the white matter and is higher to the cerebral hemispheres than the spinal cord. The capillary exchange vessels of the brain possess unique permeability characteristics which have led to the concept of the 'blood brain barrier'. CSF compared to plasma contains similar concentration of sodium and lower glucose, protein, potassium, calcium and bicarbonate but higher chloride. Regulation of cerebral Blood Flow (CBF): 1- The Cerebral Perfusion Pressure (CPP): Which equal the mean arterial blood pressure (MAP) antagonized by the intracranial pressure (ICP). CPP=MAP-ICP a. Effect of the arterial blood pressure (ABP) (Figure 13 - 1): The cerebral blood flow is autoregulated between the pressure limits of 60 and I40 mmHg, without a significant change in cerebral blood flow. The presence of baroreceptors of the carotid artery is very important to ensure an adequate supply to the brain. If the arterial blood pressure falls, below 60 mmHg, the cerebral blood flow severely decreases, and if the pressure rises above 140 mmHg the blood flow rises rapidly and cause severe overstretch or rupture of the cerebral blood vessels, resulting in serious brain edema. 2 Figure 6 – 1: Effect of arterial blood pressure on the cerebral blood flow. In hypertensive person, this autoregulatory range shifts to higher pressure levels, up to 180 to 200 mmHg. b. Effect of the intracranial pressure (ICP): A slight rise in the intracranial pressure has no effect on the CBF. The effect starts when the pressure rises to about 30 mmHg or higher. If the intracranial pressure approaches the arterial pressure, the cerebral circulation is arrested, and unconsciousness occurs. There is a compensatory rise in the arterial blood pressure to overcome the intracranial resistance and establishes again the CBF (Cushing reflex). Cushing reflex: Marked increase of ICP as in case of brain tumors leads to ischemia of the vasomotor center leading to sympathetic discharge, this results in an increase in ABP to maintain the CBF. Autoregulation: It is a process, by which the flow to brain tissue is maintained at relatively constant levels despite variations in perfusion pressure. Mechanism of autoregulation: a- Myogenic mechanism: The calibers of cerebral arterioles are adjusted to keep blood flow constant, where the vascular smooth muscles of cerebral arterioles respond to stretch by vasoconstriction and vice versa. b- Chemical (metabolic) mechanism: mainly by CO2. 3 When CPP is reduced, CO2 is accumulated which has a strong vasodilator action. On the contrary, when CPP is increased, CO2 is washed out, leading to vasoconstriction. 2 - Effect of O2 deficiency, excess CO2 or H+ concentration (metabolic factors): a. Excess CO2 and H+ concentration: CO2 is the prime chemical regulator to cerebral blood flow. CO2 increases cerebral blood flow by combining with water to from carbonic acid which dissociates to from H+. Increased extravascular H+ concentration leads to vasodilatation of cerebral vessels. This causes an increase in the blood flow which carries both CO2 and carbonic acid away from the brain tissues. This maintains a normal level of neuronal activity as the increase in H+ concentration greatly depresses neuronal activity. b. Oxygen deficiency: It leads to formation of vasodilator substances (CO2, lactic acid, adenosine, adenosine phosphate compounds, histamine, K+ and H+) which immediately cause vasodilatation and increases the blood flow. 3 -Effect of cerebral activity: The CBF is directly proportional to the activity of the brain. The increase in the activity of a localized region in the brain will be accompanied by increase in the blood flow to this segment within seconds (N.B. e.g., an increase in the occipital blood flow (visual area) when a light is flashed in the eye). 4 -Effect of autonomic nerves: The parasympathetic causes mild vasodilatation, but they play little role in the control of CBF. Excessive sympathetic stimulation causes vasoconstriction of the large and 4 intermediate sized cerebral arteries but not of small vessels to prevent the very high arterial blood pressure from reaching the smaller blood vessels, so protecting them from rupture and prevents brain hemorrhage to occur. Also, in case of rupture of small cerebral vessels (cerebral stroke) sympathetic reflexes cause vasoconstriction of large arteries to limit the intracranial hemorrhage. 5- Effect of gravity: If the body is suddenly and intensely accelerated upward (stand up from a sitting position), blood moves toward the feet and arterial pressure at the level of the head decreases, which in turn decreases the CPP and CBF. Conversely, during acceleration downward, force acting toward the head increases arterial pressure at head level, but intracranial pressure also rises, so that CBF is not severely changed. 6- The blood volume: Any decrease in blood volume as in hemorrhage tends to decrease the CBF through the associated decrease in ABP. Figure 6-2: Diagrammatic summary of the factors affecting cerebral blood flow. Brain edema: It is one of the serious conditions, because the brain is encased in a solid vault, the accumulation of edema fluid compresses the blood vessels, with eventual depression of blood flow and destruction of brain tissue. 5 The usual causes of brain edema are: i. Increase the capillary pressure as in sudden increase in the cerebral blood pressure to levels too high for the autoregulatory mechanism to deal with. ii. Damage to the capillary endothelium, the most common cause is brain concussion in which the brain tissues and capillaries are traumatized and capillary fluid leaks into the traumatized tissues. Once brain edema begins it initiates a positive feedback: Edema compresses the blood vessels which causes brain ischemia, arteriolar dilatation and increased capillary pressure → more edema. The Blood-Brain Barrier (BBB): The BBB is a term used to describe the unique properties of the microvasculature of the CNS. There are no fenestrations or clefts in the capillary endothelium and also contain a series of additional properties that allow them to tightly regulate the movement of molecules, ions, and cells between the blood and the CSF. The blood-brain barrier is formed by capillary endothelial cells with their intercellular tight junctions, astrocyte end-feet ensheathing the capillary and pericytes embedded in the capillary basement membrane (Figure 13-3). NB: Circumventricular organs are some brain parts that have fenestrated capillaries, and because of their permeability they are said to be “outside the blood-brain barrier” e.g., the posterior pituitary and the area postrema. Breakdown of the BBB can occur with ischemia, hypoxia and acute hypertension. Permeability of substances into the brain: Substances cross the blood–brain barrier into the CNS by diffusion, by selective transport, and via ion channels Highly permeable to water, CO2, O2 and most lipid-soluble substances. + + - Slightly permeable to the electrolytes such as Na , K and C1. 6 Impermeable to all proteins as plasma proteins and most non-lipid-soluble substances. Limited permeability to H+ and HCO3–. Glucose transport into CSF is enhanced by the presence of specific transporters, including the glucose transporter 1 (GLUT1) in the cerebral capillaries. Figure 6-3 Blood- Brain Barrier layers. Therefore, the blood-brain barrier functions to: 1- Maintain the constancy of the environment of the neurons in the CNS because of the sensitivity of the cortical neurons to minor ionic change of K+, Ca2+, Mg2+, H+, and other ions. 2- Protect the brain from endogenous and exogenous toxins in the blood. 3- Prevent the escape of neurotransmitters into the general circulation The Cerebrospinal Fluid 7 It is clear colorless fluid, which fills the ventricles of the brain, the cisterns around the brain and in the subarachnoid space around both the brain and spinal cord. All these chambers are connected with each other, and the pressure of the fluid is regulated at constant level. Formation and flow of CSF: (Figure13-4) It is formed at a rate of about 500 ml each day. It is formed mainly by the choroid plexuses in the lateral, third and fourth ventricles. Absorption of CSF occurs through the arachnoid villi, which are fingerlike projections of the arachnoid membrane through the walls of the venous sinuses and finally empties into venous blood. Composition of CSF: CSF similar to ultrafiltrate of plasma, but it contains proteins in trace, free plasma lipids, its glucose concentration is 30% less, its potassium is 40% less, the calcium concentration is lower, the chloride is greater 15% but sodium and osmotic pressure in the two fluids is identical. Figure 6-4: The arrows show the pathway of CSF flow from the choroid plexuses in the lateral ventricles to the arachnoid villi protruding into the dural sinuses. Function of CSF: 1- Protection: The major function of CSF is to protect the brain and the spinal 8 cord, which acts as a cushion, without its protection the brain would probably be unable to withstand even the minor traumas. 2- Homeostasis: It provides an optimal chemical environment for accurate neurological signaling as it acts as a mechanism of exchange of nutrients and removal of waste products. Cerebrospinal fluid pressure: In a normal person lying in a horizontal position, the maximum CSF pressure is 180 mm H2O. ⚫ The cranium forms a rigid cavity enclosing: the brain cerebral blood vessels and blood cerebrospinal fluid (CSF). An increase in volume of any one of these will lead to raised intracranial pressure. Factors influencing the intracranial pressure: 1- Gravity: The CSF is continuing in the ventricles and in the subarachnoid space of the brain and spinal cord. In the recumbent position the pressure equal in all these situations, in sitting or standing the pressure rises in the lumbar region and drops in the cranial region. 2- Volume of CSF: The volume of CSF is kept constant because the rate of formation equals the rate of absorption, moderate changes in the volume do not affect the intracranial pressure. Under pathological conditions as in subarachnoid hemorrhage and blockage of foramina because the rate of formation exceeds the rate of absorption, the ICP rises. 3- Systemic venous pressure: The intracranial pressure follows the systemic venous pressure but not the arterial. With forced expiration with glottis closed, the venous pressure rises (decrease venous return from brain) and if maintained the ABP drops due to a 9 decrease in the cardiac output but ICP rises. 4- Volume of the brain substance: Large brain tumor elevates the CSF pressure by decreasing the rate of absorption of fluid. Decrease in the brain volume by atrophy or surgical removal of parts of the brain results in little decrease in the intracranial pressure.. Hypertonic solutions intravenously will lead to the movement of water from the interstitial and intracellular fluid in the brain to the intravascular space. So, the brain tissue volume will decrease and therefore, ICP is lowered. Effects of increase in intracranial pressure: 1- Headache: Caused by traction on the meninges, blood vessels and nerves. 2-Vomiting: Due to stimulation of the medullary centers. 3-Papilledema (edema of the optic disc when the CSF pressure rises, it also rises in the optic nerve sheath which decreases the flow of blood in the retinal vein, thereby increases the retinal capillary pressure through the eye, which results in retinal edema. 10 Prof. Enas A Hamed Lecture # 7: Basic functions of the synapses (Physiology) By the end of the lecture the student will be able to: Specific learning Objectives: 1. Explain the principles of normal function of the nervous system including: receptors, synapses. 2. Mention main neurotransmitters of central nervous system and their receptors and functions. Contents: 1. Identify the basic functional unit of the CNS. 2. Recognized functions of afferent, interneuron and efferent neurons. 3. Compare chemical and electrical synapses and Summarize the mechanism of their actions. 4. Explore steps of chemical synaptic transmission. NARS: (4.1; 4.2). References books: Kaplan USMLE Step 1, pp. 32-34. SOMATIC REFLEX ARC It is basic unit of all integrated neural activity. COMPONENTS OF SOMATIC REFLEX ARC: 1) Receptors or sense organ. 2) Afferent (sensory) neuron: It carries impulses from receptors to center. 1 Prof. Enas A Hamed 3) Centre: It is the site of integration. 4) Efferent (motor) neurons: Arise from center which is either nucleus of motor cranial nerve or anterior horn cell (AHC) for spinal motor nerve giving its axon. 5) Effector organ: It is the organ that shows the response. 1. AFFERENT NEURONS It begins at sensory receptor in periphery, has its cell body in dorsal root ganglion (DRG) & ends in spinal cord. FUNCTIONS OF AFFERENTS: 1) Conduction of impulses from receptors to centers. 2) Divergence: one afferent fiber divided into many branches which diverge towards many cell bodies. 3) Convergence: fibers from several afferent neurons may converge towards one cell body in grey matter. Figure 7.1: Functions of afferent neurons (divergence and convergence). 2 Prof. Enas A Hamed 2. INTERNEURONS They are called "intercalated" or "internuntial" or "intermediate" neurons. They are small sized, highly excitable & numerous neurons. They provide a link between central terminals of neurons and efferent neurons (motor) or afferent neurons (sensory). Some of them are excitatory and others are inhibitory. FUNCTIONS OF INTERNEURONS: The functions of interneurons are related mainly to extensive interconnections between each other and formation of different types of interneuronal circuits. These interneuronal circuits are involved in: a) Divergence. b) Convergence. c) After discharge. d) Inhibitory circuits. 1- DIVERGENCE: It is a phenomenon in which a single input (afferent) neuron or (more commonly) interneuron divide to give several collaterals and reach a larger number of efferent neurons. Significance: spread of response from single afferent signal to several sites. 2- CONVERGENCE: It is a phenomenon in which multiple collaterals (= branches) from input (afferent) neurons or (more commonly) interneurons converge (collect) on a relatively fewer number of output (efferent) neurons. 3 Prof. Enas A Hamed These collaterals may come from a single or from multiple sources. Significance: spatial summation of post-synaptic potentials at efferent neurons. 3- AFTER DISCHARGE: Definition: prolonged (continuous) discharge from an efferent neuron even after stoppage of stimulation of afferent nerves. Mechanism: - After discharge occurs because afferent impulse doesn’t reach efferent neuron directly but through multiple interneurons which have different arrangement called “interneuronal circuits”. - There are 2 types of interneuronal circuits: i) Open chain (= parallel) circuits. ii) Closed chain (= reverberating) circuits. Figure 7.2: After discharge (parallel and reverberating) circuits. i) Open chain (parallel) circuits: 4 Prof. Enas A Hamed - In this circuit afferent neuron stimulates efferent neuron both directly and indirectly through a variable number of interneurons. Each interneuron stimulates efferent neuron and gives collateral which stimulate an adjacent interneuron & so on. - A delay of 0.5 msec occurs at each synapse in this circuit. Thus, impulse reaches efferent neuron after variable periods of delay leading to prolonged discharge even after afferent signal stoppage. ii) Closed chain (reverberating) circuits: - Afferent nerve is connected to efferent neuron through a principal interneuron. This interneuron terminates directly on efferent neuron, and at same time it gives branches which return to re-stimulate principle interneuron either directly or through interneurons. - It is clear that entry of a brief afferent signal into these circuits causes re-circulation (reverberation) of signal in circuit for prolonged periods, leading to repetitive excitation of efferent neuron. - The activity of these circuits continues till stopped by either: a) Fatigue in synapse, OR b) Inhibition from other neurons. 4- INHIBITORY CIRCUITS: - Types of inhibitory circuits: i) Lateral inhibitory circuits. ii) Recurrent inhibitory circuits. i) Lateral inhibitory circuits: - They are inhibitory interneurons which are stimulated by collaterals from mostly active or strongly stimulated neurons to inhibit less 5 Prof. Enas A Hamed stimulated neurons. - Example: ascending sensory tracts during their course to cerebral cortex. Figure 7.3: Lateral inhibitory circuits of interneurons. - Mechanism: When a certain sensory stimulus applied to skin, it produces strong stimulation of receptors and afferents of central part of receptive field. During their ascent in central nervous system they send collaterals that stimulate inhibitory interneurons resulting in inhibit less stimulated afferents arising from peripheral parts of field. - Significance: help in sharpening or focusing the sensation. ii) Recurrent inhibitory circuits: 6 Prof. Enas A Hamed Figure 7.4: Recurrent inhibitory circuits. Feedback inhibition (1) and lateral inhibition (2) of ventral horn cells through inhibitory interneuron “Renshaw cell”. - In these circuits, collaterals arise from axon of efferent neuron before leaving spinal cord that synapse with inhibitory interneurons called “Renshaw cells”. These cells send their axon terminals back on surrounding -motor neurons. Renshaw cells are stimulated by acetylcholine and when stimulated they release glycine (inhibitory neurotransmitter). The glycine released by Renshaw cells will inhibit: a) The neighboring -motor neurons: Thus, helps in focusing the motor activity to most facilitated -motor neurons. b) The originally stimulated -motor neuron: Thus, helps to limit its discharge (-ve feedback control). 3. EFFERENT NEURONS - In the spinal reflexes the efferent neurons are of two types: 1- Autonomic efferent neurons (L.H.Cs) - mediate autonomic reflexes. 2- Somatic efferent neurons (A.H.Cs)- mediate somatic spinal reflexes. Divided into 2 groups: -motor neurons and -motor neurons. Efferent neuron = AHC axon (in spinal reflex). SYNAPSES 7 Prof. Enas A Hamed Synapse: Areas of contact between neurons in the C.N.S. TYPES OF SYNAPSES: I) ACCORDING TO SITE OF CONTACT BETWEEN PRE- AND POST SYNAPTIC NEURONS: 1. Axodendretic synapses. 2. Axonsomatic synapses. 3. Axonaxonic synapses. II) ACCORDING TO TRANSMISSION MODE: It is either electrical, chemical, or by both mechanisms. a) Electrical Synapses: At electrical synapses, pre- and postsynaptic membranes are pulled so closely together that interstitial space almost disappears. Electrical synapses possess symmetrical morphology. Pre- and postsynaptic membranes are spanned by barrel-like structures called gap junction channels. When a pair of gap-junction channels lines up -- one in presynaptic membrane and a second in postsynaptic membrane -- a direct connection between cytoplasm of two cells is created. This connection allows diffusion of small molecules -- including ions -- directly from one cell to other. They resist fatigue. They can allow either unidirectional or bidirectional transfer of information. They are common in cardiac and smooth muscles and rare in CNS. They have following advantage: Fast and synchronous signal transmission between neurons. They are always 8 Prof. Enas A Hamed excitatory. b) Chemical synapses (most of synapses in CNS): At chemical synapse, neuronal membranes are separated by space called synaptic cleft (200 -300 nm). Chemical substances, called neurotransmitters, are released from sending axon and carries signals to next neuron. The transfer of information is unidirectional from pre-synaptic to post synaptic membrane. Chemical synapses carry signals more slowly in CNS than electrical synapses. They show fatigue. but, 1) Signals may be excitatory or inhibitory according to neurotransmitter released. 2) Signals can be modified as it passes from one neuron to next. c) Conjoint synapses: These are very rare in CNS. SYNAPTIC TRANSMISSION: 3 steps 1) Release of neurotransmitter into synaptic cleft. 2) Action of the neurotransmitter on postsynaptic membrane. 3) Termination of synaptic transmission. 1- Release of the neurotransmitter: - During rest, both synaptic knobs and post synaptic membrane are in a polarized state i.e.: resting membrane potential is about -70 mV. - When presynaptic neuron is stimulated and an action potential reaches synaptic knob, it causes transient opening of voltage sensitive Ca2+ channels led to Ca2+ entry into knob, Ca2+ will bind transmitter vesicles with the knob membrane at certain areas known 9 Prof. Enas A Hamed as active zones led to then open into cleft and release transmitter into cleft by exocytosis. Action of Neurotransmitters on the Postsynaptic neuron: - After a chemical transmitter is released into the cleft, it produces its effects through binding specific receptors located in post-synaptic membrane. The postsynaptic membrane receptors are of 2 types: a) Ligand-gated ionic channels. b) G-protein coupled receptors. Ligand-Gated ionic channels G-Protein coupled Receptors - Binding of these receptors to - Binding of these receptors to their specific neurotransmitter their specific transmitters produces structural changes makes them able to bind a G- that increase channels Protein molecules become permeability to ions. activated Via replacement of - There are 2 types of ligand- GDP with GTP Separation gated ion channels: of component of G-protein i) Cation channels: which can produce these effects - When activated allow passage of in postsynapticneurons: Na+, K+ and Ca2+. However, influx i) Opening of specific of Na+ is greater than K+ efflux ion channels as: because driving force for Na+ influx A second-messenger gated K+ is greater led to depolarization of channels increase K+ efflux postsynaptic membrane. hyperpolarizes postsynaptic - Transmitter which produces this neuronal membrane. effect called Excitatory ii) Activation of enzyme Transmitter. systems in cell membrane: - These channels are lined with with subsequent formation of negative charges so not allow 2nd messengers as: cAMP, - passage of anions as Cl. cGMP, IP3, DAG, arachidonic ii) Anion channels: acid control metabolic - When activated allow passage of 10 Prof. Enas A Hamed anions mainly Cl- increase pathways. Cl- influx causing iii) Regulation of gene hyperpolarization of post transcription: With alteration in synaptic membrane. Transmitter mRNA molecules which control producing such effect called synthesis of certain proteins which Inhibitory Transmitter. act as receptors & enzymes. - These channels not allow passage of Na+, K+ and Ca2+ because hydrated sizes of their molecules are larger than size of pores in the membrane. 3- Termination of synaptic transmission: By enzymatic deactivation, reuptake into nerve terminals or escape to circulation. 11 Prof. Enas A Hamed Lectures (8): Basic functions of the synapses (Physiology) By the end of the lecture the student will be able to: Specific learning Objectives: 1. Explain the principles of normal function of the nervous system including: receptors, synapses. 2. Mention main neurotransmitters of central nervous system and their receptors and functions. Contents: 1. Recognize different types of pre- and post-synaptic potentials. 2. Explain the mechanisms of pre- and post-synaptic potentials. 3. Differentiate between synaptic potential and action potential. 4. Design the mechanism and types of synaptic inhibitions. 5. Recognize the types of summation of postsynaptic potentials. 6. Know factors affecting synaptic transmissions. 7. List different properties of chemical synaptic transmission. 8. Recognize the differences between motor and reflex tetanus. 9. Paraphrase the mechanism by which synaptic plasticity occurs. NARS: (4.1). References books: Kaplan USMLE Step 1, pp. 32-34. NATURE OF POST-SYNAPTIC POTENTIALS: - The postsynaptic potentials are of 2 types: 1) Excitatory Post-Synaptic Potential (EPSP). 2) Inhibitory Post-Synaptic Potential (IPSP). 1 Prof. Enas A Hamed Table 8-1: Excitatory and inhibitory post- synaptic potentials. EPSP IPSP During rest: some Ca2+ enters the synaptic knob During rest: some Ca2+ enters the miniature EPSP. synaptic knob miniature IPSP. On stimulation: presynaptic terminals release On stimulation: presynaptic knob excitatory neurotransmitter which opens ligand-gated release inhibitory neurotransmitter cation channels with increase in Na+ ions influx more which open ligand-gated anion than K+ efflux area of depolarization (partial state) channel e.g.: Cl- influx or increase called EPSP. in K+ efflux area of hyperpolarization called IPSP. During it: excitability of the neuron is increased so During it: excitability of the neuron called excitatory. is decreased so called inhibitory. * Characterized by: * Characterized by: 1. Associated with increase in excitability of 1. Associated with decrease in postsynaptic membrane because it carries excitability of postsynaptic membrane potential towards firing level. membrane because it carries 2. Localized i.e. not spread. membrane potential away from 3. Short duration i.e. decays within 15 m.sec. the firing level. 4. Its amplitude for a single impulse is very small 2. Localized i.e. not spread. about 0.5 mv and cannot reduce membrane potential to threshold level, so to produce action 3. Short duration i.e. decays within potential must be summated. 15 m.sec. The summation is of 2 types: 4. Its amplitude for a single impulse a) Spatial: It occurs by stimulation of several is very small and cannot increase presynaptic fibers that converge on post synaptic the membrane potential to level neuron at same time. of block. To produce an effective b) Temporal: It occurs by stimulation of a single inhibition must be summated presynaptic neuron repetitively (successively) within either spatially or temporarily. very short duration (less than 15 m.sec). Examples of excitatory transmitters: Examples of inhibitory acetylcholine, norepinephrine, serotonin, L- transmitters: GABA, taurine, glutamate, L-asparate. alanine. When the summated EPSPs reach the firing level (-59 mV), This will generate an action potential at the initial segment of the axon (axon hillock). Figure 8-1: Excitatory postsynaptic potential (EPSP). 2 Prof. Enas A Hamed Figure 8-2: Inhibitory postsynaptic potential (IPSP). 3) Grand post synaptic potential (GPSP): It is sum of all EPSPs & IPSPs occurring at same time in one postsynaptic neuron. According to GPSP magnitude we have 4 possibilities: 1. Action potential if GPSP reaches the firing level. 2. Facilitation i.e. partial depolarization. 3. Inhibition i.e. hyperpolarization. 4. A balance between facilitation & inhibition no affected. Table 8-2: Difference between postsynaptic potentials and action potentials of neurons. Post synaptic potentials Action potentials Do not obey all or non-law Obey all or none law Graded Cannot be graded No absolute refractory period Absolute refractory period Summated Cannot be summated Not blocked by anesthesia Anesthesia block them Not propagated Propagated Lasts 20msec. Each lasts 1msec A) PRESYNAPTIC POTENTIALS: It is caused by third neuron acting on presynaptic neuron to cause presynaptic inhibition or facilitation. 1) Presynaptic inhibition: Axon terminals of a 3 rd inhibitory neuron end at axon terminals of an excitatory neuron. 3 rd neuron releases an inhibitory transmitter (e.g. GABA) which either closes voltage gated Ca ++ or Na + channels of excitatory neuron or opens K + or Cl - channels. 3 Prof. Enas A Hamed The end result is reduced Ca ++ entry to synaptic knob and in turn inhibits release of chemical transmitter. It develops slowly & lasts minutes to hours. 2) Presynaptic facilitation: 3 rd neuron is excitatory neuron secretes excitatory neurotransmitter (e.g. serotonin) that increased cAMP in presynaptic terminals which phosphorylates a protein in potassium channels and closes them; this prevents repolarization & prolongs depolarization. Prolonged depolarization keeps Ca ++ channels opens → increase release of chemical transmitter that remain for 3 weeks. Figure 8-3: Presynaptic facilitation. TYPES OF SYNAPTIC INHIBITION: 1) Pre-synaptic inhibition. 2) Post synaptic inhibition. 3) Collateral (or lateral) & feedback inhibitions. Figure 8-4: Presynaptic (1) & postsynaptic (2) inhibition. FACTORS AFFECTING SYNAPTIC TRANSMISSION: 1) Changes in composition of internal environment (extracellular fluid, ECF): a. pH of blood: 4 Prof. Enas A Hamed 1. Alkalosis: increase excitability and may lead to convulsion. 2. Acidosis: decrease ex